FIGURE 126-1. The acquisition, storage and trafficking of cholesterol in steroidogenic cells. ACAT1, acylcoenzyme A cholesterolacyl transferase 1; FFA, free fatty acid; HMG-CoA, 3-hydroxy-3-methylglutaryl-coenzyme A; HDL, high-density lipoprotein; LDL, low-density lipoprotein; MLN64, START domain protein 3; P450scc, cholesterol side chain–cleavage enzyme; PBR, peripheral benzodiazepine receptor; SCP2, sterol carrier protein 2; StAR, steroidogenic acute regulatory protein; StARD4, START domain protein 4.
Active steroidogenic glands accumulate the majority of their cholesterol from lipoproteins. The quantitative importance of circulating cholesterol as a hormone precursor is demonstrated by the fact that radiolabeled plasma cholesterol in man is almost fully equilibrated with the steroidogenic pool of cholesterol.12 The access of lipoproteins to certain ovarian cells may affect their ability to synthesize hormones. A partial blood-follicle barrier excludes high-molecular-weight substances including LDL from the follicular antrum.7 Thus, LDL cannot reach the granulosa cells until after the preovulatory surge of LH initiates breakdown of the follicular wall and vascularization of the developing corpus luteum.
A characteristic feature of steroidogenic cells is the presence of numerous cytoplasmic lipid droplets containing cholesterol esters.13 Sterol esters in these droplets are synthesized by acyl coenzyme A:cholesterol acyltransferase (ACAT) type 1, an endoplasmic reticulum enzyme.14 The esters generated by ACAT accumulate within the endoplasmic reticulum membranes and subsequently bud off as lipid droplets. The sterol esters in lipid droplets are hydrolyzed by the soluble cholesterol ester hydrolase (CEH) (see Fig. 126-1). Gonadotropin stimulation of cAMP-dependent protein kinases activate CEH by phosphorylation of serine residues, which promotes the association of the enzyme with perilipins, proteins that coat lipid droplets and normally restrain mobilization of sterol esters. But when phosphorylated by cAMP-activated protein kinases, perilipins facilitate the action of hormone-activated CEH, resulting in hydrolysis of the lipid droplet sterol esters.15,16 The size and the number of lipid droplets change as the ester pool expands or contracts. Cellular cholesterol ester storage is dependent on the acquisition of cholesterol by de novo synthesis or accumulation of lipoprotein-carried cholesterol on the one hand and cholesterol depletion through steroidogenic activity of the cell on the other. Trophic stimulation promotes cholesterol ester hydrolysis and diverts cholesterol into the steroidogenic pool away from ACAT, preventing re-esterification and resulting in a net depletion of cholesterol from the lipid droplets.
THE CHOLESTEROL SIDE CHAIN–CLEAVAGE REACTION
The cholesterol side chain–cleavage reaction is the first committed step, as well as the rate-limiting process, in steroid hormone synthesis.17 This reaction takes place on the inner mitochondrial membranes, catalyzed by P450scc (Fig. 126-2) and its associated electron transport system, consisting of a flavoprotein reductase (ferredoxin or adrenodoxin reductase) and an iron sulfoprotein (ferredoxin or adrenodoxin). The dissociation constant (Kd) for binding of cholesterol is about 5000 nM, whereas P450scc’s affinities for the intermediate substrates (22R) 22-hydroxycholesterol and (20R, 22R) 20,22-dihydroxycholesterol are more than 60-fold higher (Kd = 4.9 and 81 nM, respectively). Thus, once cholesterol is bound to the enzyme, it is committed to complete the reaction sequence. The estimated Kd for pregnenolone is 2900 nM, which permits the dissociation of the final reaction product from the enzyme.
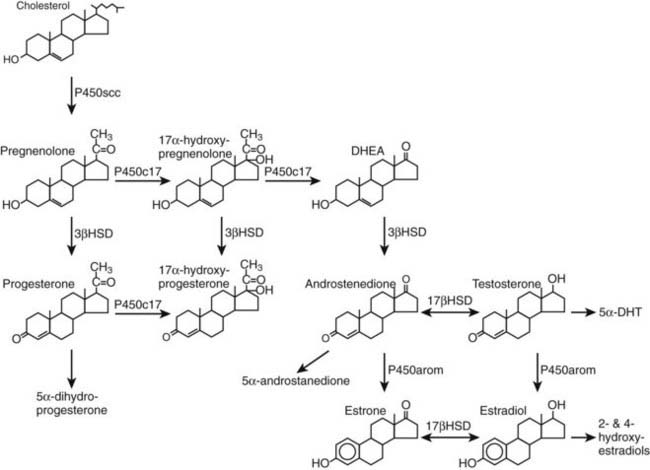
FIGURE 126-2. Major pathways of steroid biosynthesis and metabolism in the human ovary. 17βHSD, 17β-Hydroxysteroid dehydrogenase; 3βHSD, 3β-hydroxysteroid dehydrogenase; DHEA, dehydroepiandrosterone; DHT, 5α-dihydrotestosterone. Steroid metabolites in italics have unclear roles in the ovary, and their speculative functions are described in the text.
The rate of formation of pregnenolone is determined by multiple factors: (1) the delivery of cholesterol to the mitochondria; (2) the access of cholesterol to the inner mitochondrial membranes; (3) the amount of cholesterol side chain–cleavage enzyme and secondarily its flavoprotein and iron-sulfur protein electron transport chain; and (4) the catalytic activity of P450scc. Acute alterations in steroidogenesis generally result from changes in the delivery of cholesterol to P450scc, whereas long-term alterations involve changes in the quantities of enzyme proteins as well as cholesterol delivery.
The mechanisms involved in the transport of cholesterol from various substrate pools (including lipid droplets and the plasma membrane) to the mitochondria remain unclear but presumably involve the action of sterol transport proteins, including sterol carrier protein-2 and members of the steroidogenic acute regulatory protein-related lipid transfer (START) domain family. Steroid transport proteins may interact with other cytosolic proteins, including CEH, to facilitate cholesterol movement to the mitochondria.24 However, it is the translocation of cholesterol deposited in the outer mitochondrial membranes to the relatively sterol-poor inner mitochondrial membranes that is the critical step in the cholesterol side chain–cleavage process. The steroidogenic acute regulatory protein (StAR or StarD1), the prototypical member of the START domain family and a protein with a short biological half-life, appears to play a pivotal role in this translocation process.18,19 Human StAR is synthesized in the cytoplasm as a preprotein containing an amino-terminal leader sequence that directs StAR to mitochondria. After entering into the mitochondria, the leader sequence is cleaved to yield the 30-kD mature form of the protein. Phosphorylation is believed to be the mechanism by which preexisting or newly synthesized StAR can be rapidly activated. StAR contains several consensus sequences for cAMP-dependent protein kinase phosphorylation, and experimental evidence indicates that serine-195 must be phosphorylated to achieve StAR’s maximal steroidogenic activity.
Multiple lines of evidence demonstrate that StAR plays a critical role in steroid hormone synthesis. Expression of StAR mRNA and protein is correlated with steroidogenesis, and transfection of cells with a StAR expression plasmid enhances pregnenolone synthesis. The etiologic identification of congenital lipoid adrenal hyperplasia (lipoid CAH), caused by inactivating mutations of the StAR gene, conclusively established an essential role for StAR in adrenal and gonadal steroidogenesis20; patients suffering from this relatively rare autosomal recessive disorder are characterized by impaired biosynthesis of all classes of adrenal and gonadal steroid hormones due to a defect at the cholesterol side chain–cleavage step. However, 46,XX females with lipoid CAH develop female sexual characteristics at puberty and menstrual bleeding despite nonfunctional StAR proteins and adrenal insufficiency.21 The latter findings suggest that although biosynthesis of large amounts of steroids in organs such as the adrenal gland requires StAR protein function, secretion of small amounts of estrogens by the ovary sufficient to produce secondary sexual development and endometrial growth can take place independently of StAR.
The exact mechanism by which StAR increases mitochondrial cholesterol metabolism is not fully understood. Although StAR can bind cholesterol, sterol binding does not appear to be a requirement for its activity. Consequently, it has been speculated that StAR interacts with the outer mitochondrial membranes as a molten globule, unfolding in response to the perimitochondrial reduced pH and effecting an alteration in structure of the outer membrane that causes cholesterol to flow down a chemical gradient to the inner membranes and cytochrome P450scc.20 One possibility is that StAR can bind to and activate the peripheral benzodiazepine receptor (PBR), a protein integrated into the outer mitochondrial membrane. The five transmembrane domains of PBR are thought to be capable of forming a cholesterol pore, through which a cholesterol molecule can pass through the membrane.22,23 The import of StAR into the mitochondria is thought to represent the mechanism by which StAR’s actions are terminated. This model holds that StAR has two key domains, the N-terminal mitochondrial targeting sequence, which insures that the protein is directed to the site of action, and the C-terminus, which promotes cholesterol movement.
3β-HYDROXYSTEROID/Δ5-Δ4 ISOMERASE (3β-HSD)
The 3β-hydroxysteroid/Δ5-Δ4 isomerases convert pregnenolone to progesterone, 17α-hydroxypregnenolone to 17α-hydroxyprogesterone, and dehydroepiandrosterone to androstenedione (see Fig. 126-2). Using NAD+ as cofactor, these microsomal enzymes catalyze the dehydrogenation of the 3β-hydroxyl group and the subsequent isomerization of the Δ5 olefinic bond to yield the Δ4,3-ketone structure.25 The importance of 3β-HSD to steroidogenesis is highlighted by the observation that inhibitors of 3β-HSD such as epostane effectively block progesterone synthesis and interrupt early human pregnancy. Two different but highly homologous human 3β-HSD genes have been identified and localized to chromosome 1p13.1.26 The type I gene (HSD3B1) is expressed primarily in the human placenta, skin, and adipose tissue. The type II gene (HSD3B2) encodes the primary 3β-HSD enzyme expressed in the gonads and adrenal cortex. DNA sequences of the exons of HSD3B1 and HSD3B2 are so similar that the two encoded proteins—372 and 371 amino acids, respectively—differ in only 23 amino acid residues.
Before the midcycle LH surge, 3β-HSD is localized to the theca but not granulosa cells; this differential distribution of the enzyme is concordant with the androgen-synthesizing function of theca cells. The type II 3β-HSD in human corpus luteum has been localized to the perimitochondrial endoplasmic reticulum by electron microscope cytochemistry.27 The enzyme is, therefore, positioned to convert pregnenolone produced by the mitochondrial cholesterol side chain–cleavage system to progesterone. Because steroidogenic cells have a large capacity to generate progesterone when presented with exogenous pregnenolone, 3β-HSD is not thought to be a rate-determining enzyme. However, the level of 3β-HSD increases following trophic hormone stimulation.28
17α-HYDROXYLASE/17,20 DESMOLASE (P450c17 OR CYP17)
Theca cells of the follicle, theca-lutein cells of the corpus luteum, as well as the ovarian stroma express P450c17. This single microsomal enzyme catalyzes two reactions in the ovary: (1) hydroxylation of pregnenolone and progesterone at C17 and (2) conversion of 17α-hydroxypregnenolone into dehydroepiandrosterone (lyase reaction).29 The 17α-hydroxylation reaction requires a pair of electrons and one molecule of O2; a second electron pair and O2 molecule are needed for the lyase reaction. The electrons are transferred from NADPH to the P450c17 heme iron by NADPH-cytochrome P450 reductase, the importance of which is demonstrated by disordered steroidogenesis in patients harboring mutations of the POR gene encoding NADPH-cytochrome P450 reductase.30 Several factors determine whether substrates undergo only 17α-hydroxylation or the subsequent scission of the 17,20 bond. These include the nature of the substrate, the flux of reducing equivalents, posttranslational modification of P450c17, and allosteric effectors.28 The human P450c17 only utilizes Δ5 substrates for 17,20 bond cleavage (see Fig. 126-2). Phosphorylation of P450c17 appears to be necessary for maximal 17,20-lyase activity,31 and cytochrome b5 increases 17,20-lyase activity by acting as an allosteric effector rather than an electron donor.32
AROMATASE (P450arom OR CYP19) AND THE SYNTHESIS OF ESTRADIOL
Estrogens are synthesized in granulosa cells and lutein cells by the action of P450arom (CYP19).33 In most cases, P450arom-expressing cells utilize androgen precursors derived from another cell type, placing ovarian estrogen synthesis under complex regulation epitomized by the “two cell/two gonadotropin” model of follicular steroid biosynthesis. According to this model, LH drives thecal synthesis of androgens, which are subsequently aromatized to estrogens in adjacent granulosa cells (Fig. 126-3). FSH is the primary stimulator of P450arom gene expression in granulosa cells, which contain more than 99% of the aromatization capacity of the follicle.34 However, all granulosa cells are not equal in their capacity to form estrogens. There is a gradient of activity, with highest aromatase expression located in the mural granulosa cells and lowest levels in the proliferating cells near the antrum.
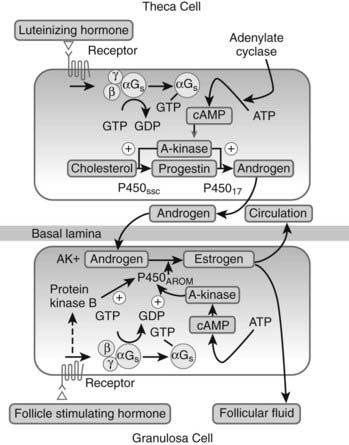
FIGURE 126-3. Gonadotropin regulation of follicular androgen and estrogen biosynthesis and the two cell/two gonadotropin model. P450arom, Aromatase; P450c17, 17α-hydroxylase; P450scc, cholesterol side chain–cleavage enzyme.
Aromatase, a microsomal member of the cytochrome P450 family, catalyzes three sequential hydroxylations of a C19 substrate using 3 molecules of NADPH and 3 molecules of oxygen to produce a C18 estrogen characterized by a phenolic A-ring, with the elimination of the C19 methyl group as formic acid.35 The first hydroxylation yields a C19 hydroxyl derivative; this is followed by a second hydroxylation step to produce an unstable gem-diol, which collapses to yield a C19 aldehyde. The final hydroxylation event involves the formation of a 19-hydroxy-19-hydroperoxide intermediate, resulting in the subsequent elimination of a formic acid molecule and aromatization of the steroid A-ring. This sequence of reactions takes place at a single active site on the enzyme, with reducing equivalents transferred to P450arom by NADPH-cytochrome P450 reductase.36 The aromatase protein is encoded by a single gene (CYP19) on chromosome 15q21.1, which gives rise to mRNAs of various sizes as a result of the use of alternative promoters within the large gene spanning more than 123 kb.33,37,38 The promoter (promoter II) that drives ovarian aromatase expression lies adjacent to the exon encoding the translation start site. In granulosa cells, FSH stimulates transcription of both CYP19 and the gene encoding NADPH-cytochrome P450 reductase.36
Gonadal tissues reduce 17-ketosteroids into 17β-hydroxysteroids, which have greater biological potency.39 Some 13 different 17β-HSDs, designated types 1 through 13 according to the chronological order in which their respective cDNAs were cloned, have been identified; most are members of the short-chain dehydrogenase/reductase (SDR) family. The type I enzyme is also referred to as the “estrogenic” 17β-HSD, since it catalyzes the final step in estrogen biosynthesis by preferentially reducing the weak estrogen, estrone, to yield 17β-estradiol, a potent estrogen, using either NADH or NADPH as cofactor. Having 100-fold higher affinity for C18 steroids over C19 steroids, the type I enzyme is located in the cytosol and is expressed in granulosa cells under the influence of FSH.40,41 The type III enzyme is referred to as the “androgenic” 17β-HSD because it catalyzes the final step in androgen biosynthesis by reducing androstenedione, a weak androgen, to testosterone, a potent androgen, using NADPH as cofactor. The type III enzyme does not appear to be expressed in the human ovary, and the primary androgen produced by the theca is androstenedione.42 The absence of the type III enzyme mRNA from the human ovary indicates that another 17β-HSD is responsible for ovarian testosterone production. The most likely 17β-HSD participating in ovarian testosterone synthesis is the type V enzyme, which is expressed in theca cells and the corpus luteum. Type VII 17β-HSD is also expressed in ovary43; its murine homologue prefers estrone as a substrate and is most abundantly expressed in the ovaries of pregnant mice.44
POSSIBLE ROLES FOR CHOLESTEROL PRECURSORS IN OVARIAN FUNCTION
Intermediates in the cholesterol biosynthetic pathway from lanosterol have been found to induce oocytes to resume meiosis.45 4,4-Dimethyl-5α-cholesta-8,14,24-triene-3β-ol was extracted from human follicular fluid and named follicular fluid meiosis activating substance, or FF-MAS. A related compound, 4,4-dimethyl-5α-cholest-8,24-diene-3β-ol, was isolated from bull testis and called T-MAS.46 Present in micromolar concentrations in preovulatory follicle follicular fluid, both compounds are synthesized from lanosterol by P450 14α-demethylase encoded by the CYP51 gene. The accumulation of FF-MAS and T-MAS in mature follicles may be the result of increased synthesis or inhibition of cholesterol synthesis at steps beyond the formation of FF-MAS and T-MAS. Gonadotropins have been reported to cause a several-fold increase in Cyp51 gene expression in rodent ovaries, which could contribute to enhanced MAS formation.47 Additionally, progestins at concentrations which are found in follicular fluid in the preovulatory period are known also to block cholesterol synthesis at late steps, which would result in an accumulation of FF-MAS and T-MAS. The physiologic roles of FF-MAS and T-MAS in oocyte maturation, if any, remain uncertain at this time. Some but not all studies on in vitro oocyte maturation suggest effects of these compounds on maturation by stimulating progression to metaphase II or increasing the survival of oocytes without affecting maturation.
OTHER STEROID HORMONES
All three classes of sex steroids—namely, progesterone, androgens, and estrogens—are converted to metabolites of uncertain functions in the ovary. This is particularly true for the corpus luteum, which produces substantial amounts of 17α-hydroxyprogesterone48 and 5α-dihydroprogesterone.49 The secretion of both of these progesterone metabolites parallels the production of progesterone during the luteal phase. Since pregnancy can be established and maintained in the absence of these molecules, they do not appear to have essential roles in reproductive physiology.
Two distinct isozymes of 5α-reductase, namely type I (SRD5A1) and type II (SRD5A2), have been identified. Both isozymes reduce the double bond between C4 and C5 and can convert progesterone to 5α-dihydroprogesterone, testosterone to dihydrotestosterone (DHT), and androstenedione to 5α-androstanedione. Whereas SRD5A1 mRNA expression has been clearly shown in the ovary, mRNA expression of SRD5A2 is contentious.50,51 Although DHT is present in follicular fluid, its precise role in normal human ovarian physiology is unclear. In rhesus monkeys which received a GnRH antagonist to interrupt spontaneous gonadotropin secretion and were infused with human FSH and LH, systemic administration of DHT significantly reduced estrogen secretion,52 whereas elevated DHT may also inhibit aromatase activity (discussed later). Together, these observations suggest that high concentrations of DHT are antagonistic to gonadotropin-stimulated estradiol secretion in primates.
The primary estrogens, estradiol and estrone, can undergo hydroxylation at C2 and C4 positions in the ovary, resulting in the formation of catechol estrogens, which are estrogens containing a dihydroxybenzene A-ring. Conversion of estradiol is catalyzed by the enzymes estrogen 2-hydroxylase (CYP1A1) and estrogen 4-hydroxylase (CYP1B1) to the catechol estrogens 2-hydroxyestradiol and 4-hydroxyestradiol, respectively. Both of these metabolites bind to the two known estrogen receptors ERα and ERβ with 7% to 13% relative binding affinity compared with estradiol.53 Catechol estrogens can be further O-methylated by catechol-O-methyl transferase (COMT) to form methoxy estrogens. The precise physiologic functions of these steroid metabolites in the ovary are unclear. Some studies suggest their roles as paracrine/autocrine regulators of steroidogenesis and follicular development.54 Catechol estrogens can also be oxidized to potent genotoxic molecules implicated in carcinogenesis,55 whereas 2-methoxyestradiol has antiangiogenic and antitumor activities. Part of the activity of 2-methoxyestradiol can be ascribed to the inhibition of the transcription factor, hypoxia-induced factor-1a.56 Thus, 2-methoxyestradiol may have a role in controlling follicular function and neovascularization prior to the ovulatory surge of LH.
Gonadotropin Control of Ovarian Steroid Production
FSH AND LH
Gonadotropins control the growth and differentiation of the steroid hormone–secreting cells of the ovary, intrinsically linking form and function. A defined sequence of gonadotropin action propels the growth of follicles and the production of steroid hormones. Positive feedback on the pituitary by high concentrations of estrogens leads to the ovulatory surge of LH, which in turn triggers a dramatic differentiation event, resulting in structural reorganization of the preovulatory follicle, release of the ovum, and striking changes in the steroidogenic capacity of the luteinizing cells.
Follicular growth, which culminates in ovulation and corpus luteum formation, requires both FSH and LH. Steroidogenic competence of the ovarian follicle is not achieved in the absence of FSH, even if LH is present in abundance. FSH promotes proliferation of the granulosa cells and induces the expression of genes involved in estradiol biosynthesis.33,36,37 Thus, mutations that lead to the synthesis of an inactive FSH β subunit57 or inactivate both alleles of the FSH receptor gene58,59 produce symptoms of primary hypogonadism with deficient ovarian estrogen production in the face of elevated plasma LH levels. Likewise, FSH cannot achieve complete regulation of follicular development by itself. In gonadotropin-deficient women or LH-deprived monkeys treated with FSH alone, follicles grow to a preovulatory size, but because of a relative deficiency in androgen precursors, there is no parallel increase in estradiol production60–62 (Fig. 126-4). The small amount of estradiol that is made in this situation may be derived from androgens secreted by the adrenal cortex or thecal androgens produced in response to paracrine factors released by the granulosa cells. During the last phase of follicular maturation, when granulosa cells acquire LH receptors, LH is then able to sustain follicular estradiol synthesis.63 This LH substitution is thought to compensate for the diminished levels of FSH of the late follicular phase consequent to negative-feedback action of estradiol and inhibin. LH action on the granulosa thus rescues the dominant LH-expressing follicle from the fate of atresia of the remainder of the cohort.63,64
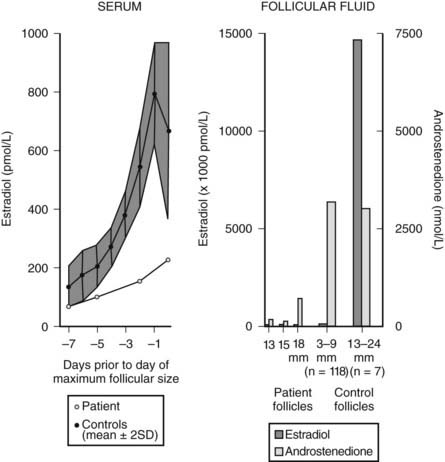
FIGURE 126-4. Left panel, Serum estradiol concentrations of a patient with isolated gonadotropin deficiency following administration of recombinant FSH prior to the day where ovarian follicles reach their maximum size (day 17). As a reference, daily serum estradiol levels (mean ± 2SD) are shown in seven normally cycling women up to the day of LH peak. Right panel, Follicular fluid concentrations of estradiol and androstenedione in three separate follicles (13, 15, 18 mm diameter) from a patient with isolated gonadotropin deficiency after administration of recombinant FSH. Median estradiol (E2) and androstenedione (AD) concentrations in small (3 to 9 mm) follicles (n = 118) and large (13 to 24 mm) follicles (n = 7) are shown.
(Data from Schoot DC, Coelingh Bennick H, Mannaerts BM, et al: Human recombinant follicle-stimulating hormone induces growth of preovulatory follicles without concomitant increase in androgen and estrogen biosynthesis in a woman with isolated gonadotropin deficiency, J Clin Endocrinol Metab 74:1471–1473, 1992.)
LH stimulation is indispensable for normal ovarian hormone production not only before but also after ovulation. Suppression of LH release leads to a prompt decline in progesterone levels that precede changes in the abundance of mRNAs encoding steroidogenic enzymes or structural changes in the corpus luteum.65 This acute regulation of ovarian progesterone secretion is controlled by LH via the expression of StAR. StAR mRNA and protein, present in both theca-lutein and granulosa-lutein cells throughout the luteal phase, are highly expressed in early and midluteal phase, whereas declining StAR mRNA and protein levels are characteristic of late luteal phase. Moreover, StAR protein levels in the corpus luteum are highly correlated with plasma progesterone levels; suppression of LH levels during the midluteal phase markedly decreases plasma progesterone levels and abundance of StAR mRNA transcripts in the corpus luteum.66
MECHANISMS OF GONADOTROPIN ACTION
LH stimulates an almost immediate increase in gonadal steroid secretion, mediated mainly by proteins phosphorylated by the cAMP-dependent protein kinase and ERK½.3 The rapid enhancement of cholesterol delivery to the inner mitochondrial membranes is driven by posttranslational changes in existing proteins, resulting in increases in their activities. These include the phosphorylation and activation of CEH,67 the phosphorylation of StAR,68 which appears to be necessary for maximal steroidogenic activity, and possibly the phosphorylation of components of the cholesterol side chain–cleavage system.69
Longer-term regulation of steroid hormone production over hours or days is exerted at the level of gene transcription. In general, increases in cAMP concentrations stimulate transcription of all of the genes involved in steroid hormone synthesis.5 However, many of these genes do not contain consensus cAMP response elements in their promoters, so transcriptional activation is mediated by other sequence motifs. Steroidogenic factor 1 (SF-1), encoded by the NR5A1 gene, is involved in the control of most of these genes.70,71 SF-1 binding elements are present in the promoters of the StAR, P450scc, type II 3β-HSD, P450c17, and P450arom genes. The interaction of SF-1 with co-activators, including cAMP response element–binding protein (CREB), binding protein (CBP), and steroid receptor coactivator-1 (SRC-1), may coordinate the cAMP response.72,73 Whereas SF-1 expression decreases after ovulation, mRNA levels of the orphan nuclear receptor LRH-1 (encoded by NR5A2) are higher in corpus luteum than in mature follicles. Unlike SF-1, the expression of which is reduced in the ovary during gestation, the expression of LRH-1 is induced significantly in the corpus luteum during pregnancy.74 The stage-specific expression of LRH-1 in the ovary, which differs from that of SF-1, implies its specific role in regulating follicular and luteal functions. Cotransfection of granulosa cells demonstrated a dose-dependant effect of LRH-1 on 3βHSD type II promoter activity, suggesting that LRH-1 can contribute to progesterone synthesis in the corpus luteum.75 Mice lacking LRH-1 expression in granulosa cells fail to ovulate and expression of the StAR and Cyp11A genes is impaired.76
The GATA family of transcription factors has recently emerged as an important group of transcription factors regulating expression of multiple steroidogenic enzymes. This basic helix-loop-helix (bHLH) family includes GATA-4 and GATA-6, which have roles in regulating the expression of StAR, P450scc, and P450c17, among other steroidogenic enzymes.77 The GATA family of transcription factors also interacts with other transcription factors, including SF-1 and Sp1, as part of a combinatorial code that regulates steroidogenic enzyme gene expression.
Some aspects of FSH-mediated granulosa-cell differentiation have been shown to be protein kinase B (PKB)-dependent. Expression of constitutively active LH receptors in rat granulosa cells resulted in increased cAMP production without increasing aromatase activity or LH receptor mRNA levels, whereas stimulation of granulosa cells by FSH in the presence of PKB led to an amplification of FSH-induced aromatase and LH-receptor mRNA levels.6 FSH via cAMP stimulates the activation of the PI3-kinase/PKB pathway in granulosa cells, leading to the activation of multiple intracellular signaling molecules.78 However, expression of constitutively active protein kinase A at high levels was able to mimic most of the actions of FSH in granulosa cells. PKA or FSH stimulation of P450scc and 3β-HSD were very similar in magnitude, but PKA was unable to stimulate expression of aromatase to the same level as FSH. This implies that there are other pathways in addition to PKA necessary for optimal aromatase expression.79
The transcription of genes encoding steroidogenic enzymes is also suppressed at specific stages of follicular and luteal function. For example, the expression of thecal P450c17 declines transiently after the LH surge, as does the expression of aromatase and type I 17β-HSD in the luteinizing granulosa cells.39,73,80 Activation of the LH receptor also activates the inositol phosphate cascade, which is able to reduce aromatase expression and enhance progesterone production. Aromatase is similarly suppressed with activation of protein kinase C or expression of the constitutively active form of Gαq, but mutant forms of the LH receptor that do not activate the phosphoinositol cascade do not inhibit aromatase.81,82 A decline in expression of the StAR gene is associated with functional luteolysis.83 Factors that block expression of steroidogenic proteins include the orphan nuclear receptor, DAX-1, which interacts directly with SF-1 to prevent activation of the transcriptional machinery.84,85 Stimulation of P450arom promoter activity by SF-1 in ovarian granulosa cells is inhibited by the transcription factors DAX-1 and WT186; all of the three transcription factors are expressed in granulosa cells of the human ovarian follicle, indicating the presence of a finely coordinated regulation of P450arom expression.87,88 Posttranscriptional control (e.g., changes in mRNA stability) is also important in certain circumstances and may account in part for the actions of insulin-like growth factors on the expression of components of the steroidogenic machinery.89,90
OTHER GONADOTROPIC HORMONES WITH POSSIBLE ACTIONS IN THE OVARY
Besides FSH and LH, other hormones also exhibit gonadotropic activity; these include prolactin, growth hormone, insulin, and insulin-like growth factors. Although prolactin plays an important role in regulating luteal function in rodents, the physiologic role of prolactin in human ovarian function is less certain. The human ovary expresses prolactin and prolactin receptors, and high levels of prolactin suppress steroidogenesis by granulosa cells.91,92 However, the menstrual disturbances observed in hyperprolactinemic women are primarily due to the consequences of reduced gonadotropin secretion; the impact of elevated prolactin levels on the ovary appears to be secondary.
Growth hormone (GH) has been reported to have direct effects on animal and human granulosa cells; its cognate receptor is expressed on granulosa cells of human antral follicles and luteal cells.93 In addition, GH administration increases the ovarian response to gonadotropins in hypopituitary subjects. Yet, supplemental GH does not appear to have dramatic effects on gonadotropin action in individuals with normal pituitary function.94
Ovarian theca and granulosa cells express the insulin receptor through which insulin augments steroid production.95 Mechanisms of this insulin effect on steroidogenesis remain to be clarified. Insulin can increase steady-state levels of steroidogenic enzyme mRNAs, but it is not yet known whether this is a transcriptional or posttranscriptional response. To date, insulin-response elements have not been identified in steroidogenic enzyme genes or their promoters. There is, however, evidence for insulin effects on mRNA stability. Additionally, insulin is known to rapidly activate translation, but it is yet to be determined if such a translational action of insulin takes place in ovarian cells.
Intraovarian Control Mechanisms
The growth of follicles and the function of the corpus luteum, while under the primary direction of the pituitary, are highly influenced by intraovarian factors that modulate the action of gonadotropins. These intraovarian factors most likely account for gonadotropin-independent follicular growth, observed differences in the rate and extent of development of ovarian follicles, arrest and initiation of meiosis, dominant follicle selection, and luteolysis. The list of potential paracrine factors that can influence steroid production by theca and granulosa cells is long and diverse. It includes various growth factors, cytokines, peptide hormones, and steroids such as epidermal growth factor, transforming growth factor β (TGF-β), platelet-derived growth factor, fibroblast growth factors, transforming growth factor α (TGF-α), activins, inhibins, antimüllerian hormone, insulin-like growth factors, estradiol, progesterone, and GnRH (Table 126-1).96–99
Table 126-1. Intraovarian Regulators Affecting Ovarian Hormone Production
Growth Factor/Cytokine | Ovarian Actions |
---|---|
Activin | |
EGF | |
FGF | |
GDF-9 | |
GnRH | |
IFN-γ | Decreases granulosa cell steroidogenesis |
IGF-1, IGF-2 | Stimulates granulosa- and theca-cell mitosis and steroidogenesis, augments gonadotropic-stimulated steroidogenesis |
IL-1 | |
IL-2 | Augments progesterone production |
IL-6/IL-11/OSM/LIF | Suppresses FSH-stimulated steroidogenesis |
Inhibins A & B | Increase thecal androgen synthesis |
KGF | |
PDGF | Augments FSH-induced LH receptor expression and steroidogenesis, enhances theca proliferation |
TGF-α | Same as EGF |
TGF-β | |
TNF-α | Inhibits gonadotropin-stimulated steroidogenesis |
EGF, Epidermal growth factor; FGF, fibroblast growth factor; GDF-9, growth and differentiation factor 9; GnRH, gonadotropin-releasing hormone; IFN, interferon; IGF, insulin-like growth factor; IL, interleukin; KGF, keratinocyte growth factor; PDGF, platelet-derived growth factor; TGF, transforming growth factor; TNF, tumor necrosis factor.
INSULIN-LIKE GROWTH FACTORS AND THEIR BINDING PROTEINS
In response to FSH, granulosa cells produce insulin-like growth factors (IGFs) as well as IGF binding proteins (IGFBPs), which have higher affinities for the IGFs than the receptor that mediates IGF action.97–99 In animal follicles, IGF-1 is the primary IGF produced, whereas in the human ovary, IGF-2 is the major IGF. In general, IGFs amplify the actions of FSH on granulosa cells, including effects on cell proliferation, cAMP accumulation, and transcriptional stimulation of some genes, including the StAR gene.89,90 Similarly, IGFs increase theca-cell androgen production and amplify the stimulatory effects of LH.100 There are also reports that IGF-1 and IGF-2 have differential effects on human ovarian cell function. While they both stimulate progesterone secretion by granulosa-lutein cells, IGF-1 but not IGF-2 increases basal aromatase activity and synergizes with FSH to increase aromatase expression.
The pattern of expression of IGF binding proteins (BPs) is determined by the stage of follicular maturation and the status of the follicle.97–99101 IGFBP-2 is expressed by theca and granulosa cells of small antral atretic follicles, whereas IGFBP-3 is expressed by theca and granulosa cells of the dominant follicle. IGFBP-4, expressed by ovarian stroma, theca cells, and the granulosa cells of small antral follicles and the dominant follicle, is a potent inhibitor of IGF action in vitro. Therefore, its cleavage by IGFBP-4 protease, also known as pregnancy-associated plasma protein-A (PAPP-A), acts as a positive regulatory mechanism of IGF bioavailability.102 Consistent with the notion that PAPP-A is a marker of ovarian follicle selection, the metalloproteinase is secreted by cultured luteinizing granulosa cells and granulosa cells from large antral follicles but not small antral follicles.103 IGFBP-5 is expressed in stroma as well as theca and granulosa cells in follicles of all sizes. IGFBP-6 is not detectable in the human ovary.
Healthy follicles are characterized by a microenvironment in which IGFs are present in the unbound form. The high levels of free IGF are achieved in part through reduced levels of IGFBP-2 and the production of an FSH-stimulated IGFBP protease by granulosa cells. In contrast, IGFBP-2 and IGFBP-4 are abundant in atretic follicles, restricting availability of free IGF. The IGF system represents a prototype for amplifying the effects of FSH during the follicular phase, permitting the dominant follicle to survive in the face of declining FSH levels.
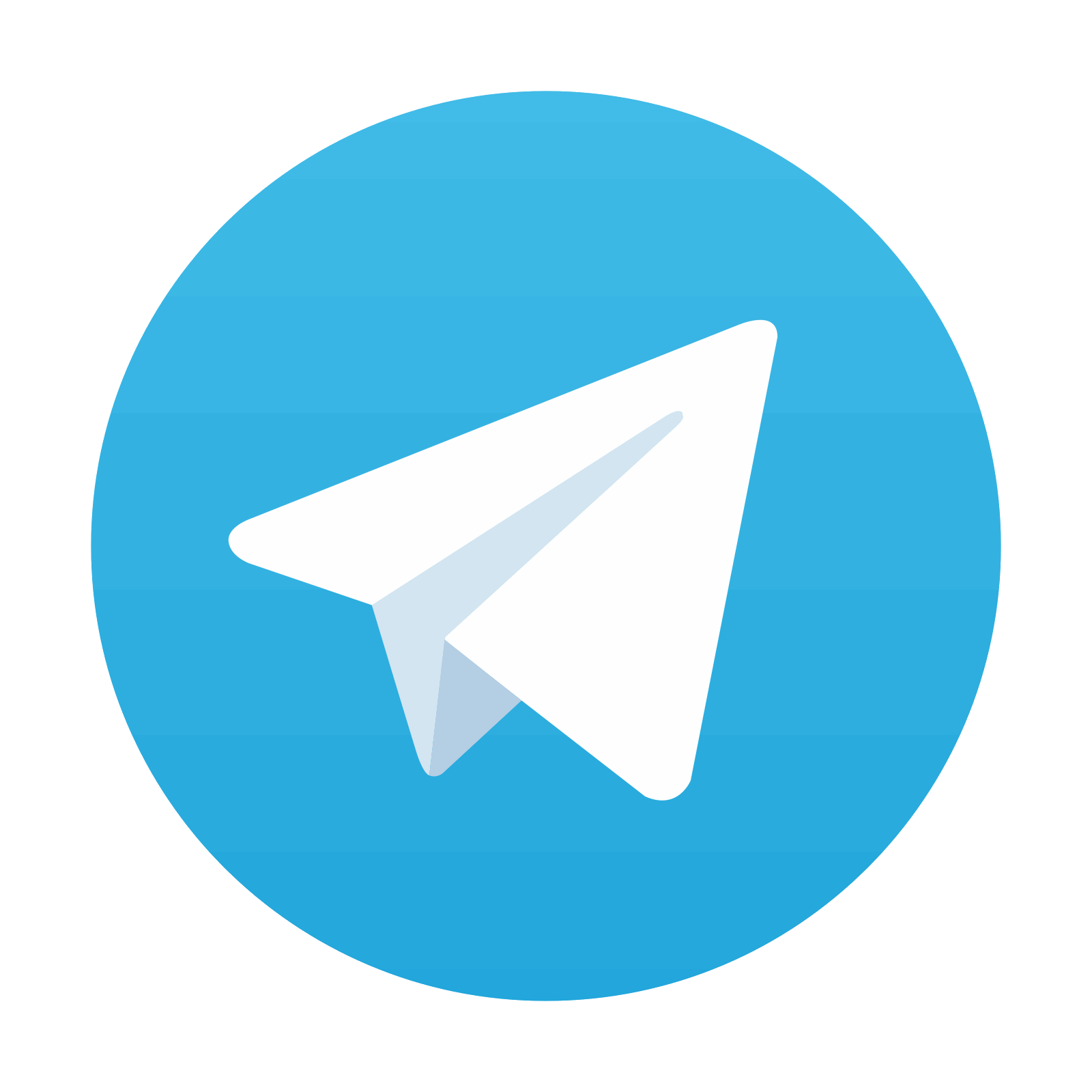
Stay updated, free articles. Join our Telegram channel

Full access? Get Clinical Tree
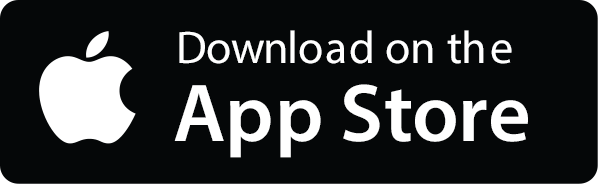
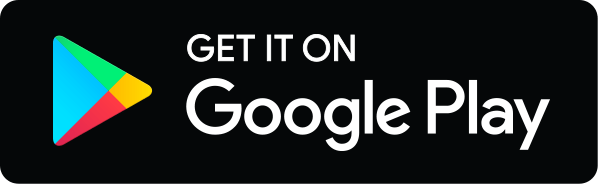