Iodine deficiency remains a public health problem in 47 countries (Fig. 88-1). However, progress has been made since 2003; 12 countries have progressed to optimum iodine status, and the percentage of school-aged children at risk of iodine deficiency has decreased by 5%.5 However, iodine intake is more than adequate or even excessive in 34 countries, increased from 27 in 2003. In Australia and the United States, two countries that were previously iodine sufficient, iodine intake is falling. Australia is now mildly iodine deficient, and in the United States, the median urinary iodine is 145 µg/L, as mentioned, which is still adequate but half the median value of 311 µg/L noted in 1970. These changes emphasize the importance of regular monitoring of iodine status in countries to detect both low and excessive intakes of iodine.
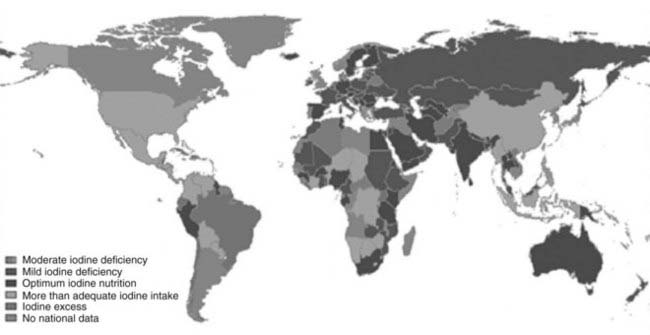
FIGURE 88-1. Current status of global distribution of iodine nutrition based on the median urinary iodine concentration by country, according to WHO database on iodine deficiency.
(Data from de Benoist B, McLean E, Andersson M: Iodine deficiency in 2007: Global progress since 2003, Food Nutr Bull 29:195–202, 2008.)
Recent reports on goiter and iodine deficiency in Europe20 have indicated, however, that goiter persists in adults (but is seldom seen in children) in Bulgaria, Czechoslovakia, the Netherlands, Belgium, and Switzerland.21 According to a relatively recent study, the iodine status of the Swiss population is currently adequate.23 Substantial areas of high goiter prevalence persist in Austria, Hungary, Romania, Poland, the constituent countries of the former Yugoslavia (Slovenia, Croatia, Bosnia and Herzegovina, Macedonia, Serbia, Montenegro), and western Russia. In other countries (southwestern Germany, Greece, Italy, Portugal, Spain, and Turkey), iodine prophylaxis is not mandatory, and goiter and even endemic cretinism continue to be major problems, either nationally or regionally (goiter prevalence rates of 18% to 22%).
Iodine deficiency has been a public health problem in most Latin American countries. Iodine status has been reassessed over the last 15 years, and programs have been implemented for the control of IDD. Great progress has been made, particularly in the aggressive push for iodized salt use. But problems remain, such as weak governmental support and lack of effective monitoring of salt iodization in some countries, threatening the effective and sustained elimination of IDD in the region. Data on the present situation, however, are incomplete because regular monitoring is carried out in only a few countries. Moreover, different methods were used to measure iodine in urine and salt, and goiter was evaluated only by palpation. Using the ThyroMobil model, which has proven to be a convenient and efficient model for standardized and rapid evaluation for urinary iodine and goiter prevalence in Europe, 163 sites in 13 countries were visited to assess randomly selected schoolchildren of both genders, 6 to 12 years of age. The median urinary iodine concentration (8208 samples) varied from 72 to 540 µg/L. The Guatemala median was below the recommended range of 100 to 200 µg/L; Bolivia, Nicaragua, El Salvador, Mexico, and Argentina were at 100 to 200 µg/L; and Peru, Honduras, Paraguay, Venezuela, Brazil, Ecuador, and Chile were higher than 200 µg/L, including three (Brazil, Ecuador, Chile) greater than 300 µg/L. Urinary iodine concentration correlated with the iodine content of salt in all countries. Median values of thyroid volume were within the normal range for age in all countries, but the goiter prevalence varied markedly from 3.1% in Bolivia to 25.0% in Nicaragua because of scatter.24,25
In the sub-Himalayan area of Pakistan, the overall prevalence of goiter is 39.7%, and endemic cretinism is common.26 In India, despite intensive efforts to promote iodized salt, only about half the population is covered, and coverage is especially poor in low socioeconomic populations. Iodized salt is unavailable in many rural markets, or salt sold as iodized is poorly or incompletely iodized or both.27 The Himalayas of India, Nepal, Bhutan, and southern China, as well as the mountains extending into northern Burma, Thailand, Laos, and Vietnam, have long been known as goitrous areas.
It is estimated that 30 million Chinese have goiter, and possibly 200,000 suffer from the consequences of endemic cretinism.28 Accordingly, new cases of cretinism have been recently detected in isolated regions of Western China.29 An intensive program of salt iodination and administration of iodized oil (orally and intramuscularly), however, has reduced the prevalence of goiter and iodine deficiency in China. A 1995 national survey among children aged 8 to 12 years showed a total goiter rate of 20.4%. A 1997 national survey of children in the same age group reported a total goiter rate of 10.9% and a median urinary iodine level of 300.2 µg/L. According to the surveillance results of 2002, the coverage of iodized salt had reached 95.2%, and the coverage of qualified iodized salt had reached 88.8%. With the implementation of the new standards for edible salt, the quality of iodized salt improved, and in 2002, the median urinary iodine among children 8 to 10 years of age was 241.2 µg/L. Furthermore, the study confirmed that the goiter rate among children in the 8-to-10 age group was continuing to decline over time, from 20.4% in 1995 down to 5.8% by 2002, so IDD can presently be considered as extensively eliminated in this country30 The Philippines and Indonesia are severely iodine deficient.31 Worse conditions persist in the remote regions of African countries.32 In sub-Saharan Africa, 64% of households use iodized salt, but coverage varies widely from country to country.33 In countries such as Sudan, Mauritania, Guinea-Bissau, and the Gambia, coverage is less than 10%, whereas in Burundi, Kenya, Nigeria, Tunisia, Uganda, and Zimbabwe, it is more than 90%. Further, iodine status varies from iodine deficiency in countries such as Ethiopia, Sierra Leone, and Angola to iodine excess in Democratic Republic of the Congo, Uganda, and Kenya.34,35 However, many countries have outstanding programs—Nigeria, for example, which has been recognized as the first African country to successfully eliminate iodine deficiency.36 In South Africa, coverage of adequately iodized household salt (i.e., iodized at >15 mg/kg) was 62.4% of households 2 years after the introduction of compulsory iodization at a level of 40 to 60 mg/kg. A total of 7.3% of households used noniodized agricultural salt and salt obtained directly from producers. The iodine concentration in salt was lower in rural areas than in urban and periurban areas. The consequences of using underiodized or noniodized salt were most likely to be experienced in the country’s three northern provinces, among people in the low socioeconomic categories, and in rural households37 (Fig. 88-2).
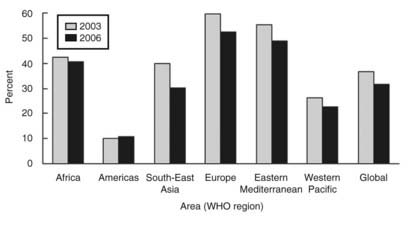
FIGURE 88-2. Change in the prevalence of individuals with an insufficient iodine intake between 2003 and 2006. Insufficient iodine intake in school-aged children has decreased by 5%, the largest decreases occurring in Southeast Asia and Europe. The Americas remained stable.
(Data from de Benoist B, McLean E, Andersson M: Iodine deficiency in 2007: Global progress since 2003, Food Nutr Bull 29:195–202, 2008.)
As assessed by measurements of urinary iodine, many countries have achieved the elimination of iodine deficiency—for example, Algeria, Kenya, Cameroon, Tanzania (Africa); Iran, Lebanon, Tunisia (Eastern Mediterranean); Bhutan, China, Indonesia, India, Thailand (Asia); Venezuela, Peru, Ecuador (Latin America); and Switzerland, Austria, Great Britain, Finland, Norway, Sweden, Poland, Macedonia, Croatia, the Czech Republic, Slovakia, and Bulgaria in Europe.5 However, in spite of the tremendous improvement of the implementation of programs of iodized salt, 35.2% of the general population in the world still had a urinary iodine below 100 µg per liter in 2003,22 and the percentage of the world population affected by goiter did not appreciably change between 1990 (12%)40 and 1999 (13%).41
Etiology
Absolute and chronic iodine deficiency is the main cause of endemic goiter and allied disorders. It is entirely possible that in certain limited situations other etiologic factors such as genetic predisposition in highly inbred and isolated groups and the presence of effective goitrogens in unusual dietary situations (Table 88-3). The arguments supporting iodine deficiency as the cause of endemic goiter are: (1) an association between low iodine content in the food and water and the appearance of the disease in the population; (2) a reduction in goiter incidence that occurs when iodine is added to the diet; and (3) demonstration that the metabolism of iodine and thyroid changes in patients with endemic goiter are similar to those produced in animals subjected to a low-iodine diet.
Table 88-3. Natural Goitrogens Associated With Endemic Goiter
Goitrogens | Agent | Action |
---|---|---|
Millet, soy | Flavonoids | Impair thyroperoxidase activity |
Cassava, sweet potato, sorghum | Cyanogenic glucosides metabolized to thiocyanates | Inhibit iodine thyroidal uptake |
Babassu coconut, mandioca | Flavonoids | Inhibit thyroperoxidase |
Cruciferous vegetables: cabbage, cauliflower, broccoli, turnips | Glucosinolates | Impair iodine thyroidal uptake |
Seaweed (kelp) | Iodine excess | Inhibits release of thyroidal hormones |
Malnutrition | Vitamin A deficiency | Increases TSH stimulation |
Iron deficiency | Reduces heme-dependent thyroperoxidase thyroidal activity | |
Selenium deficiency | Accumulates peroxides and causes deiodinase deficiency; impairs thyroid hormone synthesis |
Adapted from Zimmermann MB, Jooste PL, Pandav CS: Iodine-deficiency disorders, Lancet 372:1251–1262, 2008.
Natural goitrogens (see Table 88-3) may be considered significant determinants of the prevalence of endemic goiter, either in iodine-deficient areas or in localities where iodine intake is abundant, as in the coal-rich Appalachian area of eastern Kentucky.42 Goitrogenic effects may be related to the consumption of certain foodstuffs (cassava, millet, babassu coconut, piñon, vegetables from the genus Brassica, and soybean).44 The goitrogenic factor in cassava is related to the hydrocyanic acid liberated from the cyanogenetic glucoside (linamarin) and endogenously changed to thiocyanate, which competitively inhibits trapping and promotes the efflux of intrathyroidal iodine.43 Pearl millet is one of the most important food crops in the semiarid tropics (large portions of Africa and Asia). Millet porridge is rich in C-glucosylflavones and also contains thiocyanate. Both are additive in their antithyroid effects. In Darfur province of western Sudan, the goiter prevalence in schoolchildren was linked to the level of consumption of millet.44 Babassu coconut is largely consumed in northern Brazil, and studies have demonstrated the possible presence of flavonoids in the edible part of the nut.45 Thus in areas where millet and babassu coconut are a major component of the diet, their ingestion may contribute to the genesis of goiter. Furthermore, flavonoids, besides being potent inhibitors of thyroid peroxidase, also interact with thyroid hormone at the peripheral level.46 Turnips, like cabbage, cauliflower, and broccoli, contain glucosinolates whose metabolites compete with iodine for thyroidal uptake.44 Soy-containing foods and dietary supplements are widely consumed for putative health benefits (e.g., cancer chemoprevention, beneficial effects on serum lipids associated with cardiovascular health, reduction of osteoporosis, relief of menopausal symptoms), but studies of soy isoflavones in experimental animals suggest possible adverse effects as well, like enhancement of reproductive organ cancer, modulation of endocrine function, and antithyroid effects (due to flavonoids which impair thyroid peroxidase activity).47 Antithyroid effects may also be extended by increasing the loss of T4 from the blood via bile into the gut and may cause goiter when iodine intake is limited.48
Excess consumption of iodine-rich kelp (dry seaweed, 80 to 200 mg iodine per day) has caused sporadic and even endemic goiter in humans. In this case, goiter is common in some families and more frequent in girls at puberty, which suggests possible influences of additional genetic and hormonal factors. The organification of iodine and, consequently, the synthesis of T4 and T3 were lower than normal, and iodine-rich colloid goiter was observed in patients from the goiter-endemic coast of Hokkaido, Japan, after thyroidectomy.49
Generalized malnutrition (protein-calorie deprivation) has been recognized as an additive factor in the prevalence of endemic goiter in afflicted populations. On the basis of epidemiologic data recorded in 5- to 14-year-old South African children, it was recently shown that vitamin A supplements are effective in treating vitamin A deficiency in areas of mild ID. It also has an additional benefit: through suppression of the pituitary TSHβ gene, vitamin A supplements can decrease TSH thyroid hyperstimulation and thereby reduce the risk of goiter.50 Vitamin A deficiency was also reported to impair thyroglobulin (TG) synthesis and thyroidal iodine uptake.51
Another stimulator of follicular cell growth that acts synergistically with endogenous TSH is the anti-GAL antibody.52 This human polyclonal antibody was found to mimic the in vitro TSH effects of stimulation of cyclic adenosine monophosphate synthesis, 125I uptake, and cellular proliferation of cultured porcine thyrocytes. Anti-GAL antibodies were found to be higher in goitrous individuals and positively correlated with the size of goiter. Whether these antibodies contribute to the pathogenesis of the disease needs further clarification.
Besides iodine, several minerals and trace elements such as iron, selenium, and zinc are essential for normal thyroid hormone metabolism. Iron deficiency impairs thyroid hormone synthesis by reducing activity of heme-dependent thyroid peroxidase. Iron-deficiency anemia blunts and iron supplementation improves the efficacy of iodine supplementation.53 In several regions of the world, people are exposed to inadequate selenium supply because the selenium content of surface soil has been depleted by erosion and glacial washout, similar to iodine. In spite of that, it was shown that selenium did not significantly influence thyroid volume in borderline iodine sufficiency, because the iodine status was most likely the more important determinant.54
Zinc status also affects thyroid function. Research established a relationship between zinc deficiency and thyroid hormone levels. Zinc is required for the proper function of 1,5′-deiodinase, the enzyme required for the conversion of thyroxine to triiodothyronine.55 In animal studies, severely zinc-deficient rats had flattened epithelial cells, colloid accumulation, and lower T3 concentration; marked alterations of follicle cellular architecture, including signs of apoptosis, were found.56 In a zinc deletion–repletion study carried out in humans, TSH, total T4, and free T4 tended to decrease during the depletion phase and returned to control levels after zinc repletion.57
In addition to the aforementioned natural goitrogens, exposure to environmental chemicals may have deleterious thyroid-system effects in humans during development, especially in the nervous system, and also may adversely impact thyroid hormone metabolism (Table 88-4). The effects of some chemicals may be profound: the antithyroperoxidase (anti-TPO) activity of resorcinol is 26 times the activity of propylthiouracil. Many halogenated compounds compete with natural hormones for binding to protein carriers (transthyretin and to a lesser degree thyroxine-binding globulin), although the clinical consequences are unclear. Polychlorinated biphenyls (PCBs) may have a possible effect on thyroid hormone–regulated genes.58 Disulfides from coal processes44 and from sedimentary rock drained by water into deep wells are believed to be the cause of the incomplete reduction of endemic goiter after the use of iodized salt in Colombia.59 Perchlorate is a competitive inhibitor of the sodium/iodine symporter, decreasing the active transport of iodine into the thyroid. There has been concern that naturally occurring perchlorate and industrial contamination of water supplies with perchlorate might pose a health hazard by inducing or aggravating underlying thyroid dysfunction. So far, available evidence has demonstrated that long-term, large but intermittent exposure to perchlorate does not adversely affect thyroid function, despite a lowering of the thyroid radioactive iodide uptake (RAIU).60 Tobacco smoking is a major source of thiocyanate in humans, which inhibits the function of the iodide transporter in the lactating mammary gland. Smoking during the period of breastfeeding dose-dependently reduces breast milk iodine content to about half and, consequently, exposes the infant to increased risk of iodine deficiency.61 In brief, it seems that most goitrogens do not have a major clinical effect unless there is coexisting iodine deficiency.
Pathophysiology
Goiter was regarded as an obligatory response to prolonged and severe iodine deficiency, and an increase in thyroid iodine clearance was shown to be the basic mechanism of iodine conservation (for a review of iodine deficiency disorders, see ref. 63). Subsequently, a shift in thyroid hormone synthesis in favor of T3 indicated an additional mechanism. These concepts have improved our understanding of how humans cope with low iodine intake, as well as the effects that both lack of iodine and adaptation mechanisms have on thyroid physiology. Thus, adaptation to iodine deficiency involves a number of biochemical and physiologic adjustments that ultimately result in maintenance of the intracellular concentration of T3 within normal limits. These mechanisms are listed in Table 88-5.
Table 88-5. Mechanisms Involved in the Adaptation to Iodine Deficiency
Increased thyroid clearance of plasma inorganic iodine |
Hyperplasia of the thyroid and morphologic abnormalities |
Changes in iodine stores and thyroglobulin synthesis |
Modifications of the iodoamino acid content of the gland |
Enrichment of thyroid secretion in T3 |
Enhanced peripheral conversion of T4 to T3 in some tissues |
Increased thyroid-stimulating hormone production |
T3, Triiodothyronine; T4, thyroxine.
INCREASE IN THYROID CLEARANCE OF PLASMA INORGANIC IODINE
An increase in thyroid clearance of plasma inorganic iodine is the fundamental adaptive mechanism by which the thyroid gland maintains a constant concentration of accumulated iodine in the presence of chronic iodine deficiency. A clear inverse relationship between the plasma inorganic iodine concentration and thyroid clearance was found by several authors. The relationship is such that the product of thyroid clearance and iodine concentration is constant within the observed range of serum iodine concentrations. This product represents absolute iodine uptake, which is the mass of iodine available to the gland per unit of time. Despite the elevated clearance, absolute iodine uptake tends to be lower in iodine-deficient areas, thus indicating that the compensatory mechanism is neither perfect nor complete. An inability to fully compensate for the low plasma inorganic iodine with an appropriate increase in thyroid clearance probably accounts for the fall in iodine concentration in endemic goiter. The increased iodine trapping reflects TSH stimulation, as well as an intrinsic autoregulatory mechanism dependent on the intrathyroidal iodine concentration.
HYPERPLASIA OF THE THYROID
Although thyroid clearance may be increased without a demonstrable goiter, the anatomic accompaniment of functional activity is an increase in gland mass. Another interesting point is that iodine-concentrating ability is not uniformly distributed among follicular cells, even in normal glands. A certain level of TSH-dependent, autonomous iodine trapping is a feature of normal thyroid follicles, and the generation of new follicles from mother cells with an inherently high capacity for iodine trapping could well explain the heterogeneity in iodine metabolism among the follicles of glands affected by endemic goiter.64 Partial autonomy of iodine trapping could also account for the persistently high uptake after the administration of iodine supplements. Deficiency of cytosolic superoxide dismutase in endemic goitrous tissue has been claimed to cause more prolonged exposure to oxygen free radicals and contribute to the degenerative changes found in these tissues.65
As long as adaptation to iodine is effective, increased thyroid volume may be considered as a mechanism to store iodine during periods of increased supply to provide for less favorable periods. However, this adaptive mechanism has its limits.66 The capacity to synthesize thyroid hormones is not proportional to the increase of volume, and particularly in voluminous goiter, the thyroid function becomes insufficient.
CHANGES IN IODINE STORES AND THYROGLOBULIN SYNTHESIS
A constant finding reported in endemic goiter is a drastic reduction in iodine concentration, expressed in iodine per gram of tissue. The amount of organic iodine in a thyroid affected by endemic goiter may range from 1.0 to 2.5 mg, in contrast with values of 10 mg obtained in normal control glands. Concomitantly, thyroid iodine turns over much faster, as shown by an increase in the rate of release of 131I from the gland. The presence of two compartments of organic iodine in an iodine-deficient gland has been postulated: a slow- and a fast-releasing compartment, with different sizes. The fast-release pattern is seen in children and adolescents with small, diffuse goiters and is associated with a rapid rise in plasma-bound 131I. Most adult goitrous patients have a slow-release pattern, with normal or low protein-bound 131I and a prolonged biological half-life of thyroid 131I. Such observations suggest that intrathyroidal iodine in these longstanding multinodular glands is turning over at a subnormal rate. Slow secretion of the tracer is apparently due to dilution in a large endogenous pool of stable iodine, largely as monoiodotyrosine (MIT) and diiodotyrosine (DIT), which are present to an excessive degree in the poorly iodinated TG.
MODIFICATION OF THE IODOAMINO ACID CONTENT OF THE GLAND
Experimental studies in the rat show that thyroid hyperplasia induced by iodine deficiency is associated with an altered pattern of iodine distribution within the gland.67 An increase in labeled MIT and a decrease in the concentration of DIT, as well as a progressive increase in the ratio of T3 to T4, are the main changes in the thyroid gland occurring during prolonged iodine deficiency and are directly related to the degree of iodine depletion of the gland. These alterations caused by iodine deficiency appear to be associated with a structural change in TG. Experimental studies have shown a greater degree of heterogeneity in the TG molecule. Its altered sedimentation peak, significantly lower than 19 S, indicates failure of TG maturation. In large human goiters, as the concentration of iodine is reduced, the MIT/DIT ratio increases, and the fraction of tracer found in the form of T4 and T3 is markedly reduced. Possibly, many of the iodotyrosyl groups do not have the spatial configuration that favors the normal coupling process, and therefore only a small fraction of the iodine accumulated is actually incorporated into the normal pathway of hormone synthesis and secretion. A significant amount of iodine seems to be wasted by incorporation into iodocompounds that are clearly different from TG, that are resistant to hydrolysis, and that have a very long half-life and low molecular weight. These iodocompounds are at least in part fragments of TG.
PREFERENTIAL SECRETION OF TRIIODOTHYRONINE
The enhanced synthesis and release of T3 at the expense of T4 constitute an additional adaptive mechanism entirely different from those described above. T3 contains one iodine atom less than T4 does, and its biological activity is greater. Therefore, increasing the T3/T4 ratio of the hormones actually secreted by the thyroid makes the secretion biologically more active, although it contains less iodine. Data on thyroidal and extrathyroidal iodine kinetics obtained from experimental models and in humans with goiter have suggested preferential T3 release. Coupling of MIT and DIT seems to be favored over that of two DIT molecules and is directly related to the decreasing levels of TG iodination. A low level of TG iodination and intense TSH stimulation are necessary conditions for increasing T3 biosynthesis and release.
ENHANCED PERIPHERAL CONVERSION OF THYROXINE TO TRIIODOTHYRONINE
A compensatory increase in the peripheral conversion of T4 to T3 can occur in those with chronic iodine deficiency. It has been demonstrated in iodine-deficient animals that a striking increase in the conversion of T4 to T3 is observed in the cerebral cortex, whereas the liver shows a change in the opposite direction.68 Thus tissues highly dependent on T4 for their intracellular content of T3, such as the brain, undergo a significant increase in conversion of T4 to T3 in the presence of chronic deficiency of iodine, and this adaptation may prevent harmful consequences on brain development in the early stages of life. Brain growth is characterized by two periods of maximal growth velocity. The first one occurs during the first and second trimesters between the third and fifth months of gestation. This phase corresponds to neuronal multiplication, migration, and organization. The second phase takes place from the third trimester onwards up to the second and third years postnatally. It corresponds to glial cell multiplication, migration, and myelination. The first phase occurs before fetal thyroid has reached its functional capacity. During this phase, the supply of thyroid hormones to the growing fetus is almost exclusively of maternal origin, whereas during the second phase, the supply of thyroid hormones to the fetus is essentially of fetal origin (Fig. 88-3).69 An important recent issue regarding thyroid function and regulation in the fetus is the concept that thyroid hormones are transferred from mother to fetus both before and probably after the onset of fetal thyroid function.70 Thyroid hormones, especially T4, are already available to embryonic and fetal tissues before the onset of fetal thyroid function, which occurs in humans at mid-gestation (about 22 weeks). Thus the T4 and T3 found in early human fetuses up to mid-gestation are likely to be entirely or mostly of maternal origin. This transfer decreases but persists during later gestation. Iodine deficiency in the fetus is the result of iodine deficiency in the mother, and an insufficient supply of thyroid hormones to the developing brain may result in mental retardation.
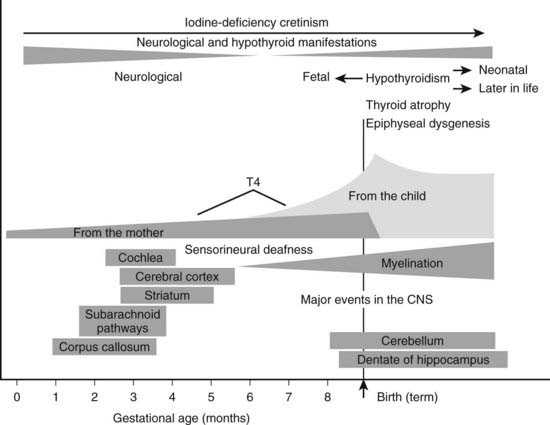
FIGURE 88-3. Proposed time course of the neurodevelopmental events and neurologic alterations associated with iodine deficiency during fetal and neonatal life. Prenatal maternal hypothyroxinemia secondary to severe iodine deficiency results in fetal neurodevelopmental damage. In addition, continuing postnatal thyroid hormone deficiency acts to determine the severity and is responsible for the varied clinical manifestations of postnatal hypothyroidism.
(Data from Berbel P, Obregón MJ, Bernal J et al: Iodine supplementation during pregnancy: A public health challenge, Trends Endocrinol Metab 18:338–343, 2007.)
INCREASED THYROTROPIN PRODUCTION
In iodine deficiency, as in other thyroid conditions with a limited glandular reserve, subjects with normal serum T3 and low T4 levels may have elevated levels of serum TSH even though they are clinically euthyroid. A clear-cut increase in the mean level of serum TSH was found in subjects living in areas where the iodine supply was reduced, and no difference was evident between individuals with and without goiter.71 Also, it has been demonstrated that serum TSH levels correlate much better with serum T4 than with serum T3. When T4 is low, the pituitary seems to be hypothyroid, whereas most other tissues are not metabolically affected, provided that the serum T3 level is normal or elevated. The most elevated TSH values have been observed in newborns and young adults living in areas with severe endemic goiter, whereas in longstanding multinodular goiter, the increased thyroid mass and the presence of autonomous areas may bring serum TSH levels toward the normal range. An increased sensitivity of endemic goiter tissue to TSH has been proposed as an additional factor for continuous goiter growth. Both thyroid peroxidase activity and 5′-deiodinase (5′DI) activity are elevated in the presence of normal serum TSH, and this increased activity has been claimed to be related to increased tissue sensitivity to TSH. In an attempt to further delineate the role of TSH in the pathogenesis of goiter, various investigators have administered thyrotropin-releasing hormone (TRH) to goitrous patients. The exaggerated and sustained TSH response to TRH observed in most studies indicates an increase in pituitary TSH reserve and less than optimal T4-induced TSH suppression at the pituitary level. This finding further documents the role in the pituitary of intracellular T3 generated from T4 in suppressing TSH (see Fig. 88-2).
ASSOCIATED PATHOLOGY
In most regions of the globe in which selenium deficiency is endemic, iodine deficiency is also endemic, but the converse is not true. Selenium deficiency is more severe in China and Tibet72 than in central Africa73 and could affect many organs, including the thyroid gland. The essential selenium is involved in thyroid hormone synthesis, metabolism, and action.74 Selenium is an integral component of two important enzymes: glutathione peroxidase and iodothyronine deiodinase. The former catalyzes the breakdown of hydrogen peroxide, thereby protecting against oxidative damage. The later catalyzes the deiodination of T4 to T3. Selenium and iodine are thus linked biochemically because both are involved in thyroid hormone production. Selenium deficiency impairs the function of 5′ DI, a selenocysteine-containing protein which plays a major role in T4 deiodination in peripheral tissues.
Clinical and Laboratory Diagnosis
The clinical picture of endemic goiter is identical to that of sporadic or simple goiter, the difference being only epidemiologic. Classically, infants and children up to school age have only diffuse enlargement of the thyroid gland. Further thyroid growth is often observed until puberty (mostly in girls) and constitutes what is commonly called diffuse colloid goiter. After adolescence, the gland becomes more nodular and grows in size as the adult ages. A few patients (less than 15% of the adult goitrous population) may exhibit very large multinodular glands, with the total mass estimated to be over 150 g. Age and gender influence the prevalence of goiter, females being more often affected than males.
The presence of endemic goiter does not cause any other recognized changes in the body unless the patient is hypothyroid, which is unusual. Goitrous individuals in areas of endemic goiter seem to feel perfectly good, are able to perform hard work, and show no signs of intellectual or physical impairment. A few patients with very large goiters may show symptoms of tracheal compression, with dyspnea or other symptoms caused by compression of the jugular veins. The intensity of the symptoms and signs resulting from compression on structures surrounding the goiter is not necessarily dependent on goiter size. Large, pendulous goiters can be seen in patients who do not have any other complaint. On the other hand, relatively small goiters enclosed in the upper thoracic region can generate signs of tracheal obstruction.
A frequent complication in large multinodular goiters is hemorrhage or infarction of a thyroid nodule, often accompanied by an inflammatory reaction and an abrupt rise in serum TG concentration. Hyperthyroidism, often caused by an autonomously functioning adenoma, is frequently observed if patients have access to even a small iodine load.75 Thyroiditis, a rare complication, is often subacute and sometimes focal. The pathologic features of endemic goiter do not materially differ from those of simple nodular goiter. Follicular and anaplastic carcinomas and especially sarcomas are more frequent in regions of endemic goiter. The prevalence of these tumor types means that highly aggressive thyroid cancer prevails in countries with endemic goiter, whereas relatively benign forms (papillary carcinomas) are less frequently recognized.76 The prognosis of thyroid cancer in regions of endemic goiter is worse than in goiter-free areas because most patients are first seen with a tumor stage in which no cure by surgery can be expected. Highly aggressive, prognostically poor types of thyroid malignancy preponderate in patients with endemic goiter. Iodine supplementation results in a relative decrease in these tumor types and hence forms of thyroid cancer with a better prognosis.
As a whole, the following tests are advised for assessment of iodine nutrition in populations: urinary iodine concentration, goiter rate, serum TSH, thyroid hormones, and serum TG. These indicators are complementary in that urine iodine concentration is a sensitive indicator of recent iodine intake (days), and TG shows an intermediate response (weeks to months), whereas changes in the goiter rate show long-term iodine nutrition (months to years).5
Serum thyroid hormone levels are a further index of the effects of iodine deficiency. Thyroid hormone concentrations are generally poor indicators of iodine status. In iodine-deficient populations, serum T3 and TSH rise or remain unchanged, and serum T4 usually falls. However, these changes are often within the normal range, and the overlap with iodine-sufficient populations is large enough to make thyroid hormone concentrations an insensitive measure of iodine nutrition. However, TSH is a sensitive indicator of iodine status in the newborn period. Elevated serum TSH, but for exceptional pathologic situations, indicates an insufficiency in the saturation of the T3 receptor in the brain, whatever the level of serum thyroid hormones. Therefore, elevated serum TSH constitutes an indicator of the potential risk of iodine deficiency on brain development. Serum T4 and T3 are less specific indicators of iodine deficiency because they are modified usually only in conditions of at least moderate iodine deficiency.41 In moderate and severe iodine deficiency, serum T4 is low, but T3 is variable or occasionally high due to preferential T3 secretion by the thyroid. Elevated serum T3 despite low serum T4 is considered a protective mechanism for most parts of the body, except the brain, where T3 is produced locally and not derived from the circulating T3.
Serum T3 levels in inhabitants of regions where goiter is endemic are typically normal or moderately elevated at a time when serum T4 and free T4 are low normal or low. Elevated serum T3 levels provide an explanation for the apparent paradox of clinical euthyroidism despite subnormal T4 levels. Serum TSH is elevated in goitrous patients with low T4 and correlates better with serum T4 than with serum T3 levels. The serum T3/T4 ratio is commonly used to express the adaptive processes that were described earlier. Euthyroid subjects living in areas where iodine is abundant have a mean ratio of 15:1, whereas in areas of endemic goiter, mean T3/T4 ratios are higher. Treatment with iodized oil causes a progressive fall in this ratio. Serum reverse T3 (rT3) tends to follow the direction of serum T4, but in the serum of pregnant women from areas of endemic goiter, rT3 is significantly higher than rT3 levels in nonpregnant subjects from the same region. An increased binding capacity of thyroxine-binding globulin is observed and may be related to the elevated serum T3 concentrations. This increased capacity could play an important role in the maintenance of a normal level of free T3.
Serum TG, which represents a sensitive marker of thyroid abnormalities and iodine deficiency in epidemiologic studies, is often elevated in patients with endemic goiter.77,78 Increased serum TG concentrations in endemic goiter could be partly related to the reduced concentration of iodine in goitrous tissue and the intrathyroidal metabolic changes secondary to persistent and chronic iodine deficiency. In areas of endemic goiter, serum TG increases owing to greater thyroid mass and TSH stimulation. Serum TG is well correlated with the severity of iodine deficiency as measured by urinary iodine.77 Whole blood from finger pricks spotted on filter paper cards can be used to measure serum TSH and serum TG as indicators of thyroid hyperstimulation and the consequence of the state of hyperstimulation, respectively.79
EVALUATION OF THE IODINE STATUS IN IODINE DEFICIENCY
In addition to the determination of serum levels of TSH, thyroid hormones, and TG, the following indicators of iodine status are evaluated in an iodine-deficient population: (1) the urinary iodine concentration and (2) the estimation of thyroid size.41
According to WHO recommendations, quantification of urinary iodine concentration expressed as µg of iodine per volume (µg I/L, pg I/dL or µg I/dL) is accepted as a good marker of the dietary iodine intake. Therefore, it is the index of choice for evaluating the degree of iodine deficiency and for measuring the improvement in iodine status after iodine prophylaxis. Iodine intake for metabolic studies is best determined from a 24-hour urine sample, but logistics make it impractical to use such measurements for epidemiologic studies. Collections of 24-hour urine are difficult to obtain and are not necessary. Relating urinary iodine to creatinine is also not practical, because urinary creatinine decomposes after 3 days without refrigeration. Furthermore, the creatinine level varies depending on age, sex, muscle mass, diseases, pH conditions, and nutritional status of the population. For these reasons and to avoid errors introduced in the performance of different creatinine assays, the WHO has recommended for epidemiologic studies the evaluation of iodine concentrations in casual spot samples, provided a sufficient number of specimens is collected. Because the frequency distribution of urinary iodine is usually skewed towards elevated values, the median is considered instead of the mean. It is appropriate to mention that the concentration of iodine in a spot or casual urine sample cannot be used to diagnose iodine deficiency in an individual. The urinary iodine concentration may vary up to threefold in an individual during a day.80 This means that it is necessary to collect repeated urine samples from an individual over a period of time and estimate the median or average, in order to evaluate their iodine status. Also, the urinary iodine (UI) concentration (µg/L) is not interchangeable with 24-hour UI excretion (µg/24 h). The two values are interchangeable only if the volume of urine passed in 24 hours is one liter. The average volume of urine passed by an adult is approximately 1.5 L/24 h. Therefore, the median UI excretion given as µg/24 h will be 50% higher than the median iodine excretion given as µg/L.81
Several methods of determination of urinary iodine have been reported, and almost all depend on the Sandell-Kolthoff reaction, in which iodide catalyzes the reduction of yellow ceric ammonium sulfate to the colorless cerous form in the presence of arsenious acid; the rate of color disappearance is proportional to the amount of iodide.82 An optimal urinary iodine concentration is 100 to 200 µg/L, corresponding approximately to a daily intake of 150 to 300 µg for adults.
The prevalence of goiter is an index of longstanding iodine deficiency and, therefore, is less sensitive than urinary iodine in the evaluation of a recent change in the status of iodine intake. Traditionally, neck palpation can detect the enlarged thyroid of moderate or severe iodine deficiency but is less reliable in mild deficiency. Recently, thyroid ultrasonography has introduced a more precise and accurate means for quantitative estimate of thyroid volume. Norms have been established for the thyroid volume of iodine-sufficient children related to age, gender, and body mass. The total goiter rate is related to the severity of iodine deficiency as follows: no iodine deficiency, 0% to 4.9%; mild deficiency, 5.0% to 19.9%; moderate deficiency, 20.0% to 29.9% and severe deficiency, more than 30%.41 Furthermore, a thyroid is considered as goitrous when its volume is above the 97th percentile established for sex, age, and body surface area in iodine-replete populations.83 In areas of endemic goiter, although the thyroid size predictably decreases in response to increases in iodine intake, thyroid size might not return to normal for months or years after correction of iodine deficiency. During this transition period, the goiter rate is difficult to interpret, because it indicates both a population’s history of iodine nutrition and its present status. Moreover, palpation of goiter in regions of mild iodine deficiency has poor sensitivity and specificity; in such areas, measurement of thyroid volume by ultrasound is preferable for the classification of goiter.84
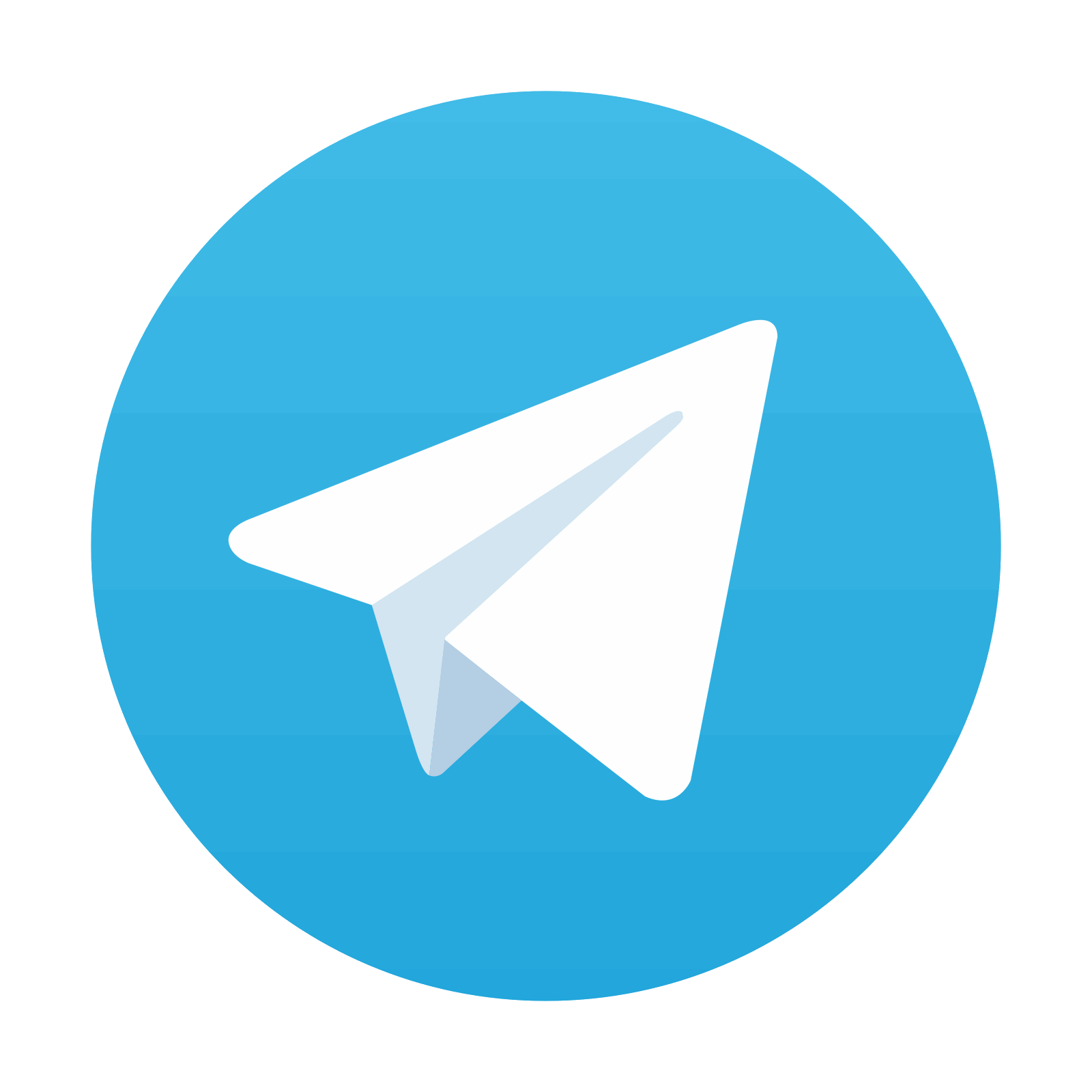
Stay updated, free articles. Join our Telegram channel

Full access? Get Clinical Tree
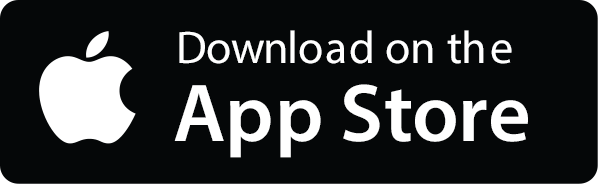
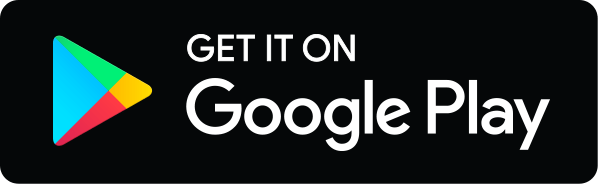