FIGURE 1-1. Timeline of selected advances in endocrinology. (ATDs, Antithyroid drugs; CAH, congenital adrenal hyperplasia; DA, dopamine; I-, iodine; OCPs, oral contraceptive pills; Pheo, pheocromocytoma; RAI, radioactive iodine; Rx, treatment; Sms A, somatostatin analogues.)
In the late 1800s, the clinical manifestations of many endocrine disorders were described. The Report on Myxedema by the Clinical Society of London (1888) is a remarkable example of the power of astute clinical observation. In addition to recognition that the adult disorder of myxedema shared certain clinical features of cretinism,3 a tenuous connection to thyroid gland dysfunction was proposed. The plates shown in Fig. 1-2 illustrate some of the clinical manifestations of hypothyroidism as described by William Ord, who coined the term myxedema (mucinous edema).4 Several years later, George Murray tested the role of the thyroid gland in myxedema by demonstrating that repeated subcutaneous injections of sheep thyroid extract corrected the disorder.5 This was probably the first example of successful hormone replacement and spawned parallel efforts for other glandular diseases. By the turn of the century, the clinical manifestations of Graves’ disease, acromegaly, Addison’s disease, diabetes mellitus, and pheochromocytoma were well established. Hormone isolation and replacement strategies became a major research effort, culminating in the characterization of corticosteroids, thyroid hormones, and sex steroids. The history of endocrinology is replete with colorful renditions of hormone isolation and discovery. A recurring theme is teamwork and parallel observations by different teams working on the same problem—a testimony to the impact of scientific communication and the need for technology to drive advances.
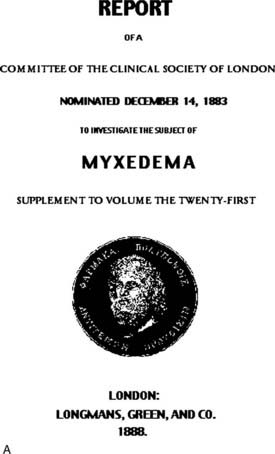
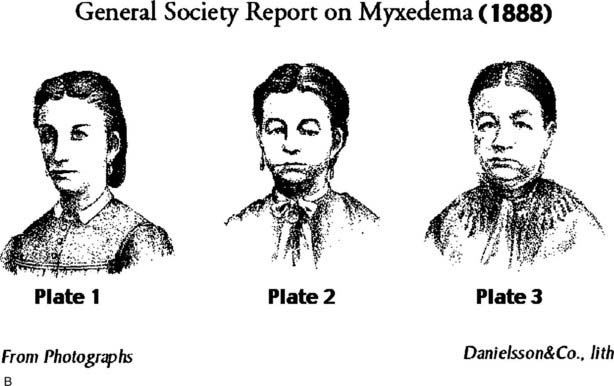
FIGURE 1-2. A, Cover page from the 1888 Society of London Report on Myxoedema. B, Clinical manifestations of myxedema. Plates taken from serial photographs of a woman with untreated hypothyroidism. Plate 1: Age 21, before onset of myxedema; Plate 2: Age 28, showing early features of myxedema; Plate 3: Age 32, illustrating overt features of myxedema.
(Source: Clinical Society of London Report on Myxedema. Boston: Francis A. Countway Library of Medicine, 1888. Photographs originally published in Ord WM: On myxoedema, a term proposed to be applied to an essential condition in the “cretinoid” affection occasionally observed in middle-aged women. Medico-chirurgical Trans 61:57–78, 1978.)
The discovery of insulin in 1921 has been chronicled extensively and is a true inflection point in endocrinology.6 The pancreatic islets form clusters that are embedded within the exocrine pancreas. Early experiments in dogs by Minkowski7 showed that pancreatectomy caused diabetes, demonstrating the pancreas as the organ responsible for regulating glucose. Banting and Best set out to isolate insulin, a process that was greatly aided by the expertise of Collip, a protein chemist who isolated several other peptide hormones, including parathyroid hormone.8,9 Despite erratic initial results in diabetic dogs, Banting and Best soon achieved unequivocal success using partially purified insulin. At the time of insulin isolation, children with type 1 diabetes had no treatment options aside from starvation therapy, which could not prevent their ultimate demise from hyperglycemia and ketoacidosis. The initial insulin treatment results were stunningly successful, providing immediate clinical benefits soon followed by the ability to achieve long-term management with repeated use of insulin injections (Fig. 1-3). This dramatic treatment strategy was stymied initially by the limited supply of purified insulin, a problem that ultimately was solved by the development of recombinant human insulin. In this current era, pancreas and islet transplantation represent alternative treatment approaches. However, limited human donor tissue and complications of immunosuppression have restricted the use of transplantation to patients with severe type 1 diabetes. There is hope, however, that stem cell biology or the ability to regenerate pancreatic islet β cells, might overcome these limitations.
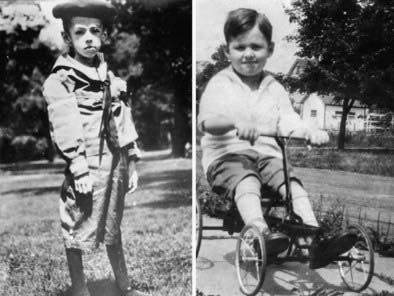
FIGURE 1-3. Treatment of type 1 diabetes mellitus with insulin. Teddy Ryder was one of the first patients treated by Dr. Banting. After undergoing “starvation treatment” (left panel), which was the only therapy available at the time, he began insulin treatment at age 5 (July 10, 1922) (right panel). One year later (July 10, 1923), he is seen “cured.” Teddy Ryder lived to age 76.
(Source: Adapted with permission from the University of Toronto Libraries Discovery and Early Development of Insulin online collection, http://digital.library.utoronto.ca/insulin/.)
Recognition that the hypothalamus produces a variety of pituitary regulatory factors was another major advance. In addition to establishing a link between the brain and the “master gland,” the hypothalamic-pituitary system underscored the critical importance of anatomic proximity and vascular delivery for the regulation of hormone action. It is now appreciated that discrete pulses of hypothalamic gonadotropin-releasing hormone (GnRH), growth hormone releasing hormone (GHRH), thyrotropin-releasing hormone (TRH), and corticotropin-releasing hormone (CRH) act locally on the pituitary and exert little, if any, physiologic effect at more distal sites in the body.
Following the isolation of many steroid and peptide hormones during the first half of the twentieth century, a conceptual framework was outlined for mechanisms of hormone action. For peptide hormones, Sutherland established the idea of a second messenger system in which a hormone binds to a membrane receptor, thereby activating intracellular second messenger pathways such as cyclic adenosine monophosphate (cAMP).10 For steroid and thyroid hormones, Tata established the concept of hormone action at the nuclear level, acting via intracellular receptors that altered gene expression, which in turn caused changes in protein levels.11 The development of the radioimmunoassay (RIA) by Berson and Yalow revolutionized endocrine physiology and diagnosis by allowing accurate measurement of minute amounts of circulating hormones.12 The impact of RIAs on physiology, endocrinology, and clinical medicine cannot be overemphasized. RIAs and related assays are now used routinely for almost all hormone measurements and have replaced many less sensitive chemical methods and bioassays. RIAs were once the province of specialty endocrine laboratories but have gradually become automated and integrated into clinical pathology laboratories. Mass spectroscopy methods are being used increasingly as a means to measure steroids and peptides. These methods not only are sensitive and highly quantitative but do not depend upon antibodies to detect specific molecules.
Important advances in therapeutic modalities have accompanied our improved understanding of endocrine diseases. Hormone replacement strategies have been refined along with advances in surgical approaches for endocrine tumors. Many hormone excess syndromes are primarily managed surgically, including transsphenoidal surgery for pituitary tumors or excision of parathyroid, adrenal, and pancreatic tumors. Many glandular surgeries are now performed via minimally invasive techniques, such as laparoscopy or video-assisted resection through very small incisions. In addition to hormonal replacements, important medical therapies that have been developed include the use of radioactive iodine13 and antithyroid drugs14 for hyperthyroidism, bromocriptine for prolactinomas and acromegaly,15 oral hypoglycemics for diabetes,16 gonadal steroids as contraceptives,17 and somatostatin analogues for acromegaly and tumors of the gastrointestinal tract.18
In recent years, the tools of molecular genetics have dramatically accelerated our understanding of endocrinology. DNA sequences encoding hormones such as somatostatin,19 growth hormone,20 insulin,21 and chorionic gonadotropin22 were among the first human cDNAs cloned. Recombinant DNA techniques are now used routinely to identify new hormones and receptors and to elucidate hormone function.
Hormone genes have provided important models for understanding mechanisms of transcriptional regulation. Hormones typically are expressed in a cell-specific manner (e.g., growth hormone, thyroglobulin), providing prototypes for identifying transcription factors (e.g., Pit-1, TTF-1) that restrict expression to particular cells or tissues. Hormone-regulated pathways have provided experimental variables that can be switched on or off, thereby revealing highly regulated target genes that can be used as experimental models. Thus, studies of the cAMP signaling system have unraveled the protein kinase A cascade and transcription factor targets, such as cAMP response element binding protein (CREB) (see Chapter 4). Nuclear receptor pathways have been particularly illuminating. In addition to identifying target genes regulated by hormones such as estrogen, glucocorticoid, or thyroid hormone, detailed analyses of these pathways have helped to define how DNA binding specificity is encoded in promoters, and how transcription factors suppress or enhance gene expression by recruiting corepressor or coactivator complexes (see Chapter 6). Transcription by nuclear receptors is arguably the best understood paradigm for how transcription factors initiate transcription, assemble a transcription complex, and renew the process to ensure multiple rounds of RNA synthesis. The genetic basis for several hundred endocrine disorders has been determined through the use of molecular biological approaches, and these tests are being used increasingly in clinical practice (see Chapter 7).
In addition to technical advances such as RIAs and recombinant DNA technology, endocrinology has contributed disproportionately to pivotal conceptual advances in science and medicine. Almost every aspect of physiology is tied to rhythms. The endocrine system has provided models for rapid rhythms such as luteinizing hormone (LH) or GH pulsatility, circadian rhythms such as cortisol or vasopressin production, and longer rhythms such as the menstrual cycle or bone remodeling. Concepts of hormone-receptor interaction and second messengers established signal transduction paradigms that proliferated into innumerable signaling networks. Polypeptide precursors, such as pro-opiomelanocortin (POMC), preproglucagon, and others, established pathways for protein processing, transport, and secretion. Studies of growth factors helped to refine concepts of autocrine and paracrine action, which can be viewed as an extension of classic endocrine action. Hormone replacement formed the foundation for the use of biologic agents such as factor VIII, G-CSF, and erythropoietin. The genetic basis for cancer has been elucidated by studies of the multiple endocrine neoplasia syndromes, types I and II.
Principles of Hormone Action
The principles of hormone action include fundamental concepts such as hormone biosynthesis and secretion, feedback regulation, hormone-receptor binding, and initiation of intracellular signaling. These principles are broadly applicable and can be applied to the physiology of other subspecialties.
HORMONE BIOSYNTHESIS AND SECRETION
Hormones can be divided into five major classes: (1) amino acid derivatives such as dopamine, catecholamines, and thyroid hormone; (2) small neuropeptides such as GnRH, TRH, somatostatin, and vasopressin; (3) large proteins such as insulin, LH, and PTH produced by classic endocrine glands; (4) steroid hormones such as cortisol and estrogen that are synthesized from cholesterol-based precursors; and (5) vitamin derivatives such as retinoids (vitamin A) and vitamin D. As a rule, amino acid derivatives and peptide hormones interact with cell-surface membrane receptors. Steroids, thyroid hormones, vitamin D, and retinoids are lipid soluble and interact with intracellular nuclear receptors.
Many peptide hormones are produced from precursor polypeptides. Characteristic signal or leader sequences target these peptides for extracellular transport via secretory granules. Some precursors, such as the POMC or preproglucagon, encode multiple biologically active peptides that are generated by specific processing enzymes; other precursors, such as preproPTH, preproinsulin, and vasopressin, encode single hormones that are excised from larger proteins. The secretion of peptide hormones is tightly controlled by intracellular signals that regulate vesicle transport and fusion with the plasma membrane, resulting in hormone release into the extracellular milieu (see Chapter 3). Steroid hormones such as progesterone, cortisol, and testosterone are synthesized from cholesterol derivatives through a series of enzymatic steps. These enzymes are expressed specifically in steroidogenic tissues such as the adrenal gland and gonads. Their enzymatic activities are regulated in response to trophic hormones such as adrenocorticotropic hormone (ACTH), LH, or follicle-stimulating hormone (FSH). Thyroid hormone is produced by modifications (iodination) of tyrosines in thyroglobulin. Vitamin D and retinoic acid are derived in part from dietary sources but can also be generated and activated by endogenous synthetic pathways.
FEEDBACK REGULATION
The elucidation of negative feedback has had a profound impact on endocrinology. This principle holds that hormones have a particular set point that is controlled by downregulating stimulatory pathways when the set point is exceeded, and upregulating stimulatory pathways when hormone levels fall below the set point. Probably every hormone is regulated in this manner, although the regulatory pathways might not be immediately evident for new hormones. These regulatory loops are well illustrated by the major hypothalamic-pituitary-hormone axes and include both stimulatory (e.g., TRH stimulates thyroid-stimulating hormone [TSH]; TSH stimulates T4/T3 production) and inhibitory components (e.g., T4/T3 suppress TRH and TSH) (Fig. 1-4). Feedback regulation also occurs for endocrine systems that do not involve the pituitary gland. For example, calcium feeds back to inhibit PTH, glucose inhibits insulin secretion, and leptin acts on hypothalamic pathways to suppress appetite. Although these feedback mechanisms oversimplify the complex physiologic pathways that regulate hormone levels, they provide useful insight into endocrine testing paradigms. For example, hypothyroidism is characterized by elevated TSH, an appropriate physiologic response to deficient thyroid hormone levels. Dexamethasone suppression of the CRH/ACTH axis is used to diagnose Cushing’s disease, which is characterized by impaired negative feedback regulation. A deficient adrenal response to exogenous ACTH is used to document primary adrenal insufficiency.
PARACRINE AND AUTOCRINE REGULATION
Whereas feedback mechanisms control many classic endocrine pathways, local regulatory systems, often involving growth factors, play critical roles in all tissues (Fig. 1-5). Paracrine regulation refers to factors released by one cell that act on an adjacent cell in the same tissue. For example, somatostatin secretion by pancreatic islet delta cells inhibits insulin secretion from nearby β cells. The oocyte produces growth and differentiation factor-9 (GDF-9), which acts on adjacent granulosa cells to stimulate the transition of primary follicles to secondary follicles. The anatomic relationships of cells have an important influence on paracrine regulation. Seminiferous tubules are exposed to a very high testosterone concentration from the interstitial Leydig cell compartment. On the other hand, the Sertoli cell product, androgen-binding protein (ABP), helps to retain high local testosterone concentrations. Activin exerts paracrine effects in the pituitary, where it stimulates FSH production. However, activin also exerts biologic activity in many other tissues, perhaps explaining why it is regulated locally and is neutralized by binding proteins such as follistatin. Autocrine regulation describes the action of a factor on the same cell from which it is produced. IGF-1 acts on many cells that produce it, including chondrocytes, breast epithelium, and gonadal cells. Intracrine regulation refers to effects within a cell. The term is not commonly used but captures the important concept that many signaling and enzymatic pathways are influenced by other pathways or by substrate or product concentrations. For example, 3-hydroxy-3-methylglutaryl coenzyme A (HMG-CoA) reductase, the rate-limiting enzyme in cholesterol biosynthesis, is inhibited by the end product, cholesterol.
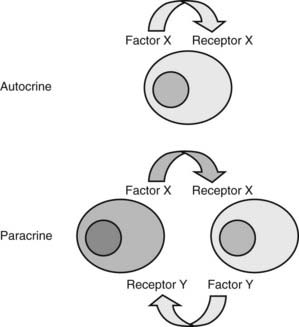
FIGURE 1-5. Autocrine and paracrine regulation. Many growth factors act locally to regulate cell growth, differentiation, and function. Autocrine regulation describes the action of a factor that acts on the same cell, whereas paracrine regulation describes a circumstance in which the product of one cell acts on a different cell type.
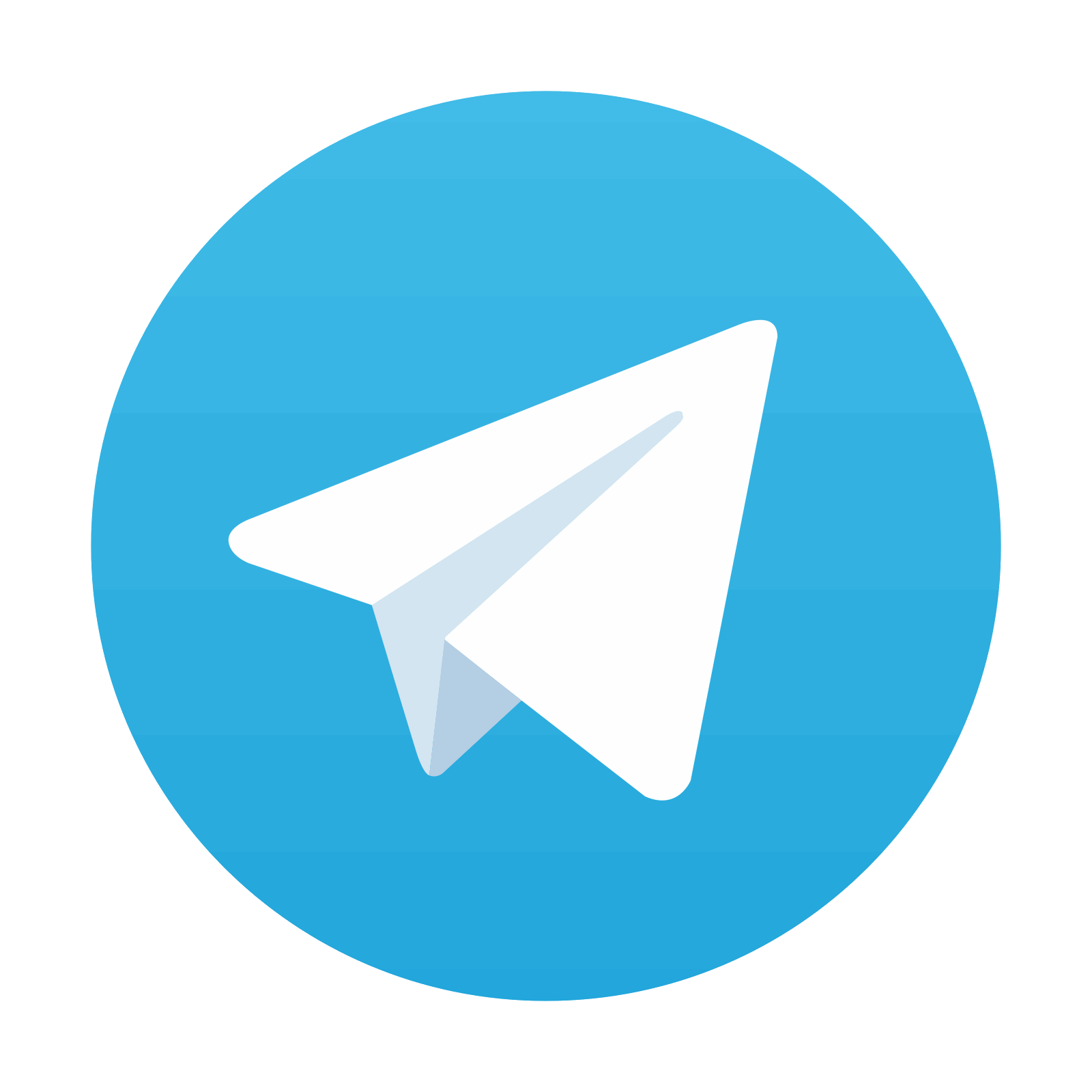
Stay updated, free articles. Join our Telegram channel

Full access? Get Clinical Tree
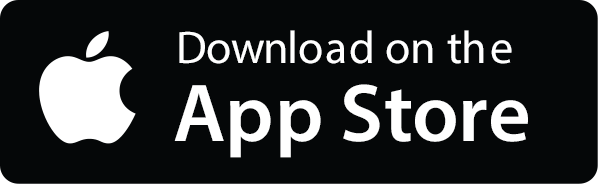
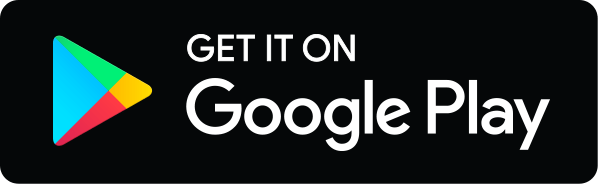