hemostasis is restored, however, if ASA-inhibited platelets are mixed with untreated platelets.14 After a single dose of aspirin, ASA-exposed platelets are inhibited for the rest of their lifespan, but platelets produced after clearance of the drug restore hemostatic function. Therefore, platelets from donors who have taken ASA are acceptable as long as 48 hours have elapsed from the last dose. Platelets donated within 2 days of ASA ingestion are acceptable for use if mixed with platelets from unexposed donors.5
significantly.51 2,3-DPG levels improve rapidly within the first 6 hours after transfusion, and return to near-normal levels within 24 hours.48,52
is carried out in a closed system, the platelets can be stored for 5 days in the appropriate plastic bag.2,5,77 Apheresis platelets are usually collected from random donors but can also be collected from HLA-compatible donors to support alloimmunized patients. There is no significant functional difference between apheresis platelets and those from whole blood donations.75 However, pheresis platelets typically contain fewer WBCs and, therefore, are less likely to cause febrile transfusion reactions than whole blood-derived platelet concentrates,78,79 unless these concentrates have been leukoreduced prior to storage.80,81 The use of apheresis platelets also results in fewer donor exposures for recipients.
of transmitting enveloped viruses, such as HIV, HCV, and HBV. It appears to be therapeutically equivalent to FFP.122 A version of this product introduced in the US in the late 1990s was withdrawn after reports of thromboembolic complications. European versions of this product, however, are in current use and have a good safety record. In contrast to individual plasma units, this pooled plasma product has not been associated with transfusion-related acute lung injury (TRALI), presumably because of dilution of antibodies from individual donors during plasma pooling.
for the irradiation of blood and blood products is 2500 cGy at the center of the irradiation field, with a minimum dose of 1500 cGy at any point in the field.5,131 This dose of radiation has no significant adverse effect on red cell, platelet, or granulocyte function. However, there are changes in the red cell membrane that result in an increased loss of potassium from the cell, limiting the storage time of red cell concentrates to 28 days.46,47 The amount of accumulated free potassium in the supernatant of irradiated red cells may be clinically important in massive transfusion, especially in the neonate.132 It may be desirable to irradiate proximate to transfusion, or wash stored irradiated RBCs if massive transfusion of irradiated products is required for a patient at risk for hyperkalemia. The dose of irradiation used for cellular blood components is not sufficient to inactivate pathogens.133 The irradiation doses required for pathogen inactivation would irreparably damage blood components.
It appears that there is not an increased risk associated with infusion of unwashed shed blood if the volume reinfused is limited to approximately 1 L. There is little value to salvaging shed blood in settings where the volume of fluid drained from the surgical site is small or has a low hematocrit. Because these devices are expensive, patient selection is important.152
TABLE 21.1 BLOOD COMPONENTS AND INDICATIONS FOR USE | ||||||||||||||||||||||||||||||||||||||||||||||||||||||||||||||||||
---|---|---|---|---|---|---|---|---|---|---|---|---|---|---|---|---|---|---|---|---|---|---|---|---|---|---|---|---|---|---|---|---|---|---|---|---|---|---|---|---|---|---|---|---|---|---|---|---|---|---|---|---|---|---|---|---|---|---|---|---|---|---|---|---|---|---|
|
90-day mortality.191 This was confirmed by a subsequent192 randomized, prospective study. Prospective, randomized trials in patients undergoing cardiac surgery193 and receiving critical care194 have each demonstrated that such patients can tolerate anemia without transfusion to hemoglobin levels between 7 and 8 g/dl, with equivalent clinical outcomes comparable to patients maintained at hemoglobin levels of greater than 10 g/dl. A Cochrane meta-analysis of prospective randomized trials comparing “high” versus “low” hemoglobin thresholds on more than 3700 patients195 found that a hemoglobin of 7 g/dl was sufficient for the majority of patients.
scenarios.206 Despite the need for consensus on the management of patients with massive bleeding, currently no such consensus exists.
TABLE 21.2 CLINICAL SIGNS OF BLOOD LOSS | ||||||||||||||||||||
---|---|---|---|---|---|---|---|---|---|---|---|---|---|---|---|---|---|---|---|---|
|
reacts with all RBCs including their own, and the transfusion of serologically incompatible red cells may be necessary. At a hemoglobin <6 g/dl, most patients require transfusion. In these cases, withholding transfusion in the absence of “compatible” RBCs places the patient in needless danger. Although “incompatible” cells will have a shorter than normal lifespan, transfusion reactions are infrequent. The risk of complications is increased if the patient has brisk hemolysis and a large volume of blood is infused, or if the patient has an undetected alloantibody in addition to the autoantibody. If time allows, special techniques should be used to evaluate these patients for alloantibodies prior to transfusion (see “Autoagglutination”). Consultation with a transfusion medicine specialist is recommended in these cases.
antisera may be modified by the manufacturer with the addition of high concentrations of protein or by chemically altering the immunoglobulin (Ig) G molecules in such a way that they perform as direct agglutinins in the laboratory. This permits rapid, reliable testing to determine the D antigen status of the cells. However, these high-protein reagents may cause false positive reactions because of spontaneous aggregation of some red cells in their presence. If this happens, an Rh-negative patient could be typed as Rh-positive if the recommended Rh control is not tested simultaneously. This problem has led to the development of low-protein, saline-reactive monoclonal reagents. Monoclonal anti-D reagents contain both human IgM and polyclonal IgG antibodies and are currently most widely used. They can also be used in the antiglobulin test for weak D. As with all reagents, the manufacturers’ instructions must be followed.2
TABLE 21.3 ABO GROUPING | |||||||||||||||||||||||||||||||||||
---|---|---|---|---|---|---|---|---|---|---|---|---|---|---|---|---|---|---|---|---|---|---|---|---|---|---|---|---|---|---|---|---|---|---|---|
|
TABLE 21.4 SIGNIFICANCE OF CERTAIN BLOOD GROUP ANTIBODIES | |||||||||||||||||||||||||||||||||||||||||||||||||||||||||||||||||||||||||||||||||||||||||||||||||||||||||||||||||||
---|---|---|---|---|---|---|---|---|---|---|---|---|---|---|---|---|---|---|---|---|---|---|---|---|---|---|---|---|---|---|---|---|---|---|---|---|---|---|---|---|---|---|---|---|---|---|---|---|---|---|---|---|---|---|---|---|---|---|---|---|---|---|---|---|---|---|---|---|---|---|---|---|---|---|---|---|---|---|---|---|---|---|---|---|---|---|---|---|---|---|---|---|---|---|---|---|---|---|---|---|---|---|---|---|---|---|---|---|---|---|---|---|---|---|---|
|
the Lewis, MN, P, and Ii blood group systems. With the important exception of ABO system antibodies, many of the others detected with this test are of no clinical significance, as they are not reactive at 37°C.
red cells should be used; if plasma is required, group AB plasma should be used because it contains no anti-A or anti-B.
TABLE 21.5 SELECTION OF BLOOD AND PLASMA BY ABO TYPE | |||||||||||||||||||||||||||||||||||||||||||||
---|---|---|---|---|---|---|---|---|---|---|---|---|---|---|---|---|---|---|---|---|---|---|---|---|---|---|---|---|---|---|---|---|---|---|---|---|---|---|---|---|---|---|---|---|---|
|
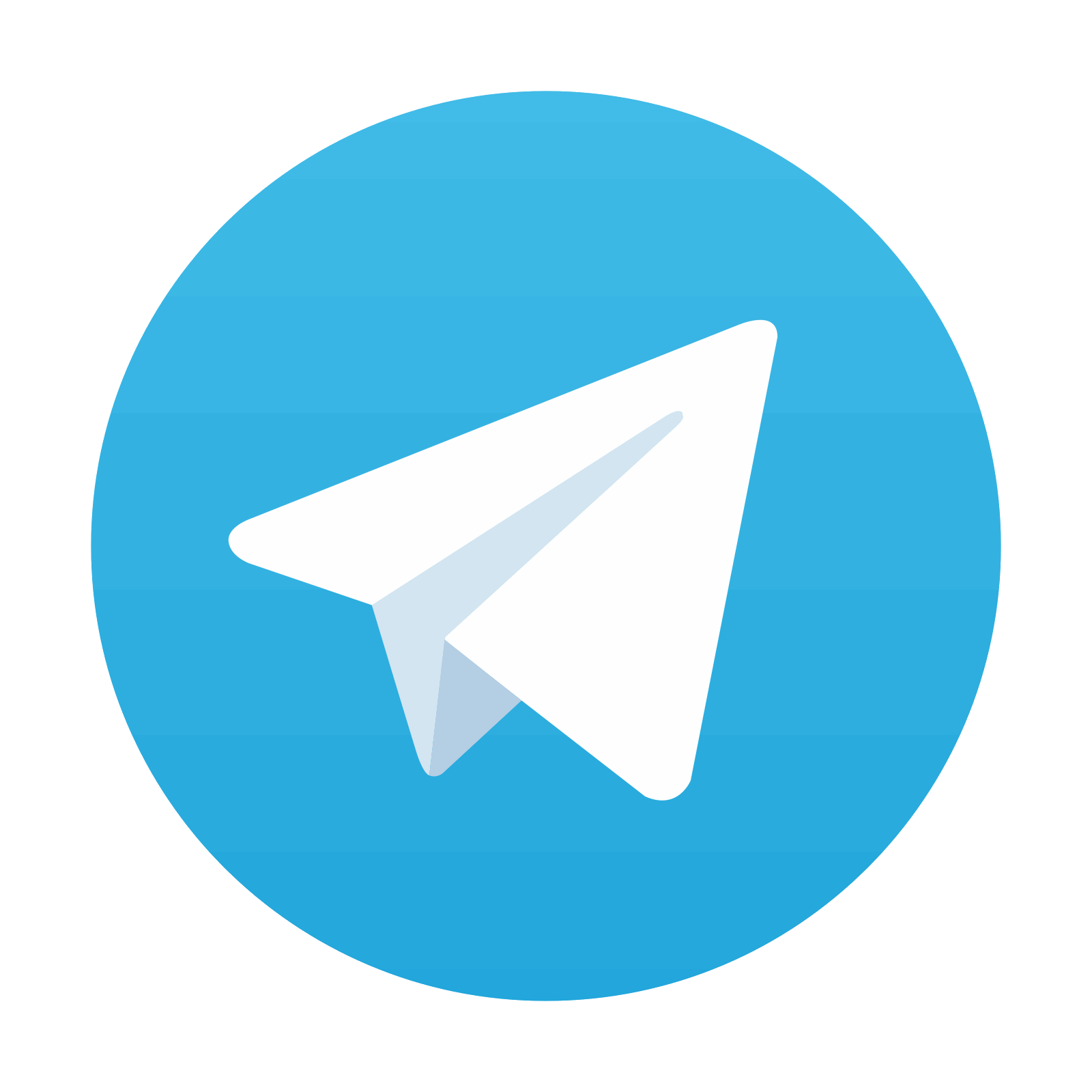
Stay updated, free articles. Join our Telegram channel

Full access? Get Clinical Tree
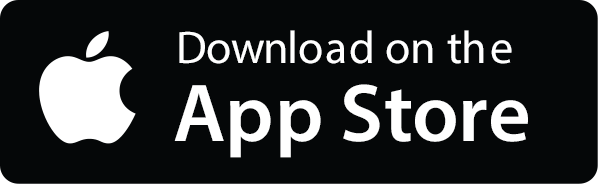
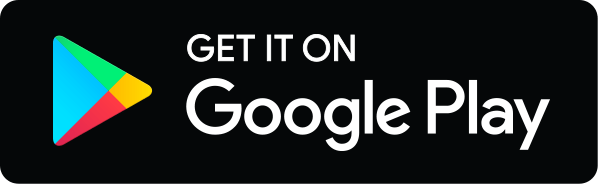
