Fig. 12.1
Trafficking of nucleic acid-sensing Toll-like receptors (TLRs). To avoid risks of recognizing self-nucleic acids in an extracellular milieu, nucleic acid-sensing TLRs exhibit distinctive intracellular compartmentalization and trafficking. Unc-93 homolog B1 (UNC93B1) physically associates with nucleic acid-sensing TLRs in the endoplasmic reticulum (ER) and facilitates delivery of TLR9 to COPII (coat protein complex II) vesicles, which transport cargo from the ER to Golgi. TLR9 traffics via the cell surface en route to endosomes, accompanying UNC93B1 and adaptor protein (AP)-2, a complex that directly interacts with UNC93B1 and facilitates endocytosis from the cell surface. TLR7 does not go through this route but traffics from Golgi directly to endosomes, accompanying UNC93B1 and AP-4 (not depicted)
Trafficking of nucleic acid-sensing TLRs from the ER to the endosomal compartments is controlled by distinctive mechanisms (Fig. 12.1). The multiple membrane-spanning protein UNC93B1 (Unc-93 homolog B1) is a crucial regulator of TLR trafficking from the ER (Kim et al. 2008). UNC93B1 physically associates with TLRs in the ER and facilitates delivery of TLR9 to COPII (coat protein complex II) vesicles, which transport cargo from the ER to Golgi (Lee et al. 2013). Notably, UNC93B1 remains associated with TLRs through post-Golgi sorting steps. Furthermore, the sorting routes are different among TLRs (Lee et al. 2013). TLR9 traffics via the cell surface en route to endosomes, accompanying UNC93B1 and adaptor protein (AP)-2, a complex that directly interacts with UNC93B1 and facilitates endocytosis from the cell surface. TLR7 does not go through this route but traffics from Golgi directly to endosomes, accompanying UNC93B1 and AP-4, which has been implicated in vesicular trafficking between the trans-Golgi network and endosomes. Such a difference in trafficking may result in access of TLR9 and TLR7 to compartments with different functional properties.
12.4 Endosomal Transport of CpG DNA
After arriving at the endosomal compartments, nucleic acid-sensing TLRs recognize endocytosed DNA or RNA. When it occurs in dendritic cells (DCs) and macrophages, they produce two classes of cytokines: type I IFN (e.g., IFN-α, IFN-β) and pro-inflammatory cytokines [e.g., tumor necrosis factor (TNF), interleukin (IL)-6, IL-12]. Importantly, pDCs produce a vast amount of type I IFN, particularly IFN-α, together with pro-inflammatory cytokines in response to TLR7 and TLR9 engagement (Kadowaki et al. 2000; Ito et al. 2002), whereas myeloid antigen-presenting cells, such as cDCs and macrophages, produce a comparatively small amount of type I IFN (Ito et al. 2002). Here, important questions are as follows: (1) what is the mechanism by which the same cell differentially produces type I IFN and pro-inflammatory cytokines?; and (2) what is the mechanism by which pDCs produce a vast amount of type I IFN? A seminal work by Honda et al. presented an initial conceptual breakthrough for these questions (Honda et al. 2005). It had been shown that different classes of oligonucleotides (ODNs) elicit different cytokine production by pDCs (Krieg 2002). CpG-A ODNs form large multimeric aggregates and strongly induce type I IFN, whereas CpG-B ODNs remain monomeric and preferentially induce pro-inflammatory cytokines (Kerkmann et al. 2005). Remarkably, Honda et al. has shown using mouse pDCs that this phenomenon is due to differential trafficking of the two classes of ODNs to endosomal compartments where TLR9 signaling leads to activation of distinct transcription factors (Fig. 12.2a). CpG-A preferentially traffics to and is retained for a long period in early endosomes where TLR9 signaling recruits IFN regulatory factor (IRF) 7, leading to the production of type I IFN. In contrast, CpG-B preferentially traffics to late endosomes where TLR9 signaling recruits nuclear factor (NF)-κB, leading to the production of pro-inflammatory cytokines. Guiducci et al. have shown similar results using human pDCs (Guiducci et al. 2006). These studies indicate that the structural conformations of ODNs determine their distinct localization, which leads to different cytokine production. Consistent with this, complexing CpG-B with a cationic lipid alters the localization of CpG-B to early endosomes in pDCs, resulting in type I IFN production (Honda et al. 2005). Furthermore, whereas CpG-A induces cDCs and macrophages to produce pro-inflammatory cytokines alone, complexing with a cationic lipid induces these cells to produce type I IFN. Thus, pDCs and the myeloid cells share functionally distinctive signaling components for type I IFN production. However, some unique biological characters of pDCs are likely to provide these cells with the capacity to preferentially produce a large amount of type I IFN.
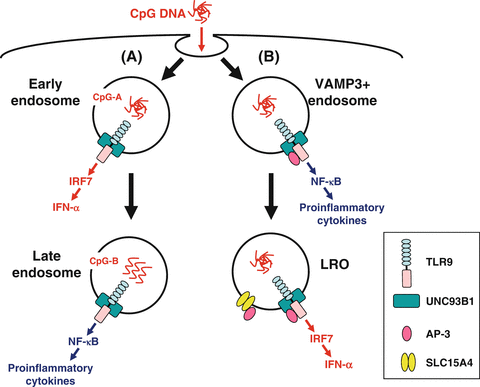
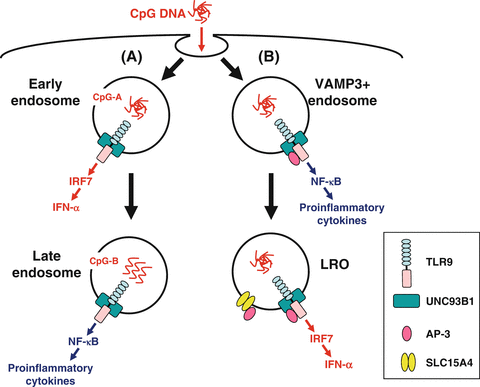
Fig. 12.2
Endosomal transport of CpG DNA. Two models have been proposed regarding the mechanisms by which plasmacytoid dendritic cells (pDCs) produce a large amount of interferon (IFN)-α and differentially produce IFN-α and pro-inflammatory cytokines. (a) CpG-A [multimeric aggregated oligonucleotides (ODNs)] preferentially traffics to and is retained for a long period in early endosomes where Toll-like receptor (TLR) 9 signaling recruits interferon regulatory factor (IRF) 7, leading to the production of IFN-α. In contrast, CpG-B (monomeric ODNs) preferentially traffics to late endosomes where TLR9 signaling recruits nuclear factor (NF)-κB, leading to the production of pro-inflammatory cytokines. (b) Adaptor protein (AP)-3 interacts directly with TLR9 and facilitates trafficking from vesicle-associated membrane protein (VAMP) 3+ endosomes to specialized lysosome-related organelle (LRO) compartments. VAMP3+ endosomes recruit NF-κB signaling and initiate pro-inflammatory cytokine production, whereas LROs recruit IRF7 signaling and initiate IFN-α production. AP-3 also interacts with SLC15A4 and may recruit it to LROs, where SLC15A4 plays an essential role in IFN-α production
More recently, two groups have shown that AP-3 is crucial for TLR7-/9-dependent type I IFN production (Sasai et al. 2010; Blasius et al. 2010). AP-3 is a heterotetrameric adaptor complex that associates with clathrin coats on the trans-Golgi network and endosomes and directs cargo to late endosomes/lysosomes or, in certain cell types, to lysosome-related organelles (LROs) (Bonifacino and Traub 2003). LROs comprise a heterogeneous set of organelles that share features with late endosomes/lysosomes but have specialized functions (Raposo et al. 2007). Examples of LROs are lytic granules in cytotoxic lymphocytes, MHC class II compartments in antigen-presenting cells, and melanosomes in melanocytes. Sasai et al. have shown that AP-3 interacts directly with TLR9 and facilitates trafficking from endosomes marked by an early endosome marker vesicle-associated membrane protein (VAMP) 3 to specialized LRO compartments marked by lysosome-associated membrane protein (LAMP) 2 (Sasai et al. 2010) (Fig. 12.2b). pDCs from Ap3b1 (a subunit of the AP-3 complex)-deficient mice fail to produce type I IFN in response to TLR7/9 ligands, whereas these cells exhibit normal or moderately enhanced production of IL-12p40. Sasai et al. concluded that TLR9 in VAMP3+ NF-κB endosomes transmits a pro-inflammatory cytokine-inducing signal, whereas TLR9 conveyed by AP-3 to LAMP2+ LROs (IRF7 endosomes) transmits a type I IFN-inducing signal. Blasius et al. reported cytokine profiles of pDCs that disagree with this; mice deficient in any of three protein complexes involved in Hermansky-Pudlak syndrome [AP-3, biogenesis of LROs complex (BLOC)-1, BLOC-2] fail to produce both type I IFN and a pro-inflammatory cytokine TNF (Blasius et al. 2010). Furthermore, mice deficient in solute carrier family 15 (SLC15) A4, a proton-coupled histidine and oligopeptide co-transporter, also fail to produce both type I IFN and pro-inflammatory cytokines TNF, IL-6, and IL-12p40 (Blasius et al. 2010). Importantly, neither AP-3 nor SLC15A4 is necessary for TLR7/9 signaling in cDCs. As SLC15A4 has an AP-3-binding motif in its cytoplasmic domain, Blasius et al. proposed that LRO trafficking capacitates the endosome by directing the incorporation of proteins, including SLC15A4, that allow TLR signaling within the specialized environment of pDCs (Fig. 12.2b). Of note, Sasawatari et al. have shown that exogenously added histidine suppresses TLR9-triggered cytokine production by wild-type but not SLC15A4-deficient DCs (Sasawatari et al. 2011). As SLC15A4 transports histidine out of lysosomes, Sasawatari et al. proposed that SLC15A4 deficiency may result in accumulation of histidine in lysosomes, which may abrogate acidification of lysosomes and type I IFN production by pDCs.
The involvement of LROs in TLR9-mediated type I IFN signaling is difficult to reconcile with the previous studies identifying early endosomes as the key compartment (Honda et al. 2005; Guiducci et al. 2006) because these two organelles are distinct. Nonetheless, a series of studies have revealed that pDCs possess specialized endosomal compartments, apparently LROs, that are well-equipped with machinery for a vast amount of type I IFN production upon TLR7/9 triggering.
12.5 A Proteasome Inhibitor Bortezomib Suppresses the Activity of Plasmacytoid Dendritic Cells (pDCs) by Targeting Intracellular Trafficking of TLR9
The elaborate vesicular traffic system in pDCs is likely to be maintained by multiple layers of signaling pathways, and may thus be susceptible to pharmacological intervention.
Proper functioning of highly secretory cells such as pDCs depends on the unfolded protein response (UPR), i.e., coordinated handling of ER stress caused by a burden of unfolded proteins in the lumen of the ER (Todd et al. 2008). After sensing unfolded proteins, an ER-resident transmembrane endoribonuclease IRE1 splices the mRNA of a transcription factor XBP1, which results in the conversion of an inactive unspliced XBP1 (XBP1u) to an active spliced XBP1 (XBP1s) protein (Fig. 12.3a). XBP1s induces transcription of a broad array of UPR genes that assist in protein synthesis and secretion (Lee et al. 2003b). It has been shown that development of XBP1-deficient pDCs is reduced, likely due to their increased sensitivity to apoptosis induced by ER stress (Iwakoshi et al. 2007).
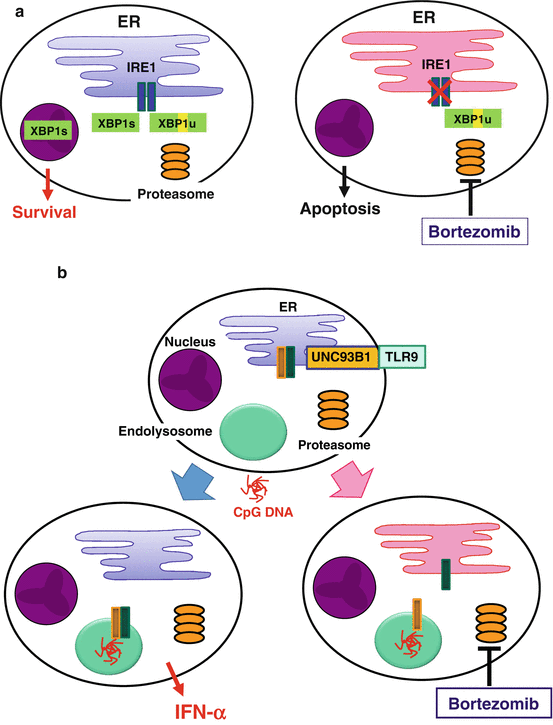
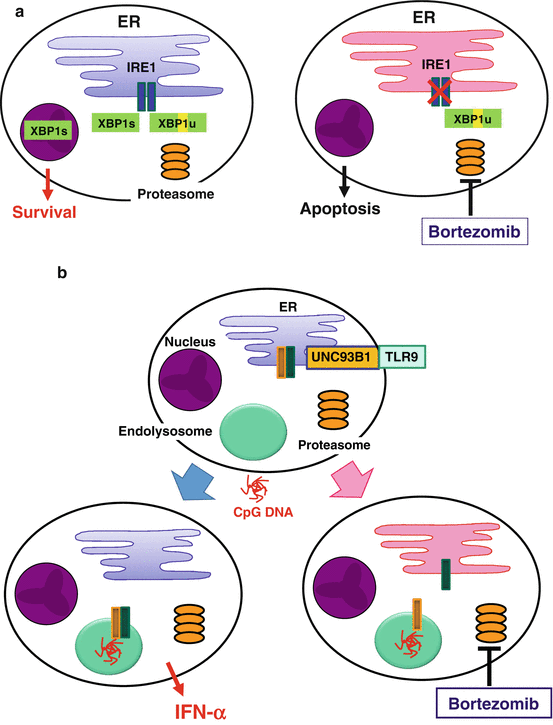
Fig. 12.3
Suppression of plasmacytoid dendritic cells (pDCs) by a proteasome inhibitor, bortezomib. (a) In highly secretory cells such as pDCs and plasma cells, coordinated handling of endoplasmic reticulum (ER) stress is necessary for maintaining survival and function. For example, an ER-resident endonuclease IRE1 transforms unspliced XBP1 (XBP1u) into spliced XBP1 (XBP1s), which induces transcription of a broad array of unfolded protein response (UPR) genes. Bortezomib increases ER stress and suppresses the activity of IRE1, leading to apoptosis. (b) Stimulation with CpG DNA induces coordinated trafficking of Toll-like receptor (TLR) 9 and Unc-93 homolog B1 (UNC93B1) from the ER to endolysosomes, which may depend on proper function of the ER. Bortezomib disturbs the ER function and dissociates TLR9 from UNC93B1, leading to suppression of IFN-α production
A selective inhibitor of the 26S proteasome bortezomib has been established as an effective drug for plasma cell myeloma (Richardson et al. 2006). The selectivity of bortezomib for myeloma appears to be due to increased susceptibility of myeloma cells to ER stress-induced apoptosis (Lee et al. 2003a; Obeng et al. 2006). Proteasome inhibitors suppress the activity of IRE1 and stabilize the dominant negative XBP1u protein, resulting in a decrease in the activity of XBP1s in myeloma cells (Lee et al. 2003a) (Fig. 12.3a). Proteasome inhibitors also prevent retrograde translocation of misfolded proteins in the ER to the cytosol, resulting in the accumulation of a large amount of misfolded immunoglobulin in the ER in myeloma cells (Mancini et al. 2000). Such overloading of the ER might compromise physiological functions of the ER. Importantly, pDCs resemble plasma cells, in that both have the developed ER (Grouard et al. 1997), are highly secretory, and depend on ER homeostasis, particularly on XBP1, for their development and survival (Reimold et al. 2001; Iwakoshi et al. 2003, 2007). Furthermore, coordinated trafficking of the two ER-resident proteins, TLR and UNC93B1, is necessary for pDCs to respond to the TLR ligands (Kim et al. 2008). Therefore, it is possible that bortezomib suppresses the activity of pDCs by targeting the two critical events in the ER: the UPR and the coordinated function of the ER-resident TLRs and UNC93B1. Indeed, we found that pDCs were most susceptible to the killing effect of bortezomib among immune cells in human blood (Hirai et al. 2011). This correlates with a decrease in the generation of XBP1s (Fig. 12.3a). Bortezomib suppressed the production of IFN-α and IL-6 by pDCs activated with a TLR9 ligand, which appears to be partially independent of apoptosis. Importantly, bortezomib inhibited translocation of TLR9, but not of UNC93B1, from the ER to endolysosomes (Fig. 12.3b). Thus, bortezomib suppresses the activity of pDCs by disturbing ER homeostasis, which results in disruption of the coordinated trafficking of TLR9 and UNC93B1 from the ER to endolysosomes and eventually in apoptosis of pDCs. This study illustrates that proper functioning of pDCs depends on undisturbed ER, which may represent a target to treat pDC-mediated inflammatory disorders.
12.6 A Tyrosine Kinase Inhibitor Dasatinib Suppresses the Activity of pDCs by Targeting Endosome Transport of CpG DNA
In addition to trafficking of TLRs from the ER to endolysosomes, trafficking of TLR ligands through the endosomal compartments may be susceptible to pharmacological intervention. Protein phosphorylation is at the heart of controlling endosomal trafficking and of integrating them with signal transduction networks of the cell (Liberali et al. 2008). Thus, protein kinases may represent another target to suppress the activity of pDCs.
Dasatinib, a tyrosine kinase inhibitor for chronic myeloid leukemia, is capable of inhibiting a broad array of tyrosine kinases, among which SRC family kinases (SFKs) are prominent targets (Lombardo et al. 2004). We found that dasatinib and SFK inhibitors strongly suppressed the production of IFN-α and pro-inflammatory cytokines (TNF, IL-6) by pDCs stimulated with CpG-A without reducing viability, whereas these inhibitors did not suppress the cytokine production induced by CpG-B as strongly in comparison (Fujita et al. 2013). Neither dasatinib nor SFK inhibitors inhibited endocytosis of CpG ODNs or the trafficking of TLR9 from the ER to endosomes induced by CpG ODNs. Whereas chloroquine, which inhibits proteolysis of TLRs in endolysosomes, did not suppress global tyrosine phosphorylation induced by CpG ODNs, dasatinib and SFK inhibitors suppressed it, indicating that these inhibitors suppress intracellular events upstream of TLR9. Importantly, dasatinib, but not SFK inhibitors, abrogated prolonged localization of CpG-A in early endosomes (Fig. 12.4). These data suggest that dasatinib inhibits SFK-independent endosomal retention of CpG-A as well as certain SFK-dependent steps upstream of TLR9, resulting in suppressing the production of IFN-α and pro-inflammatory cytokines. This study provides a clue to being able to dissect molecular mechanisms for the distinctive behavior of endosomes in pDCs as well as possibilities to develop novel therapies for inflammatory disorders by targeting the endosomal trafficking in pDCs.
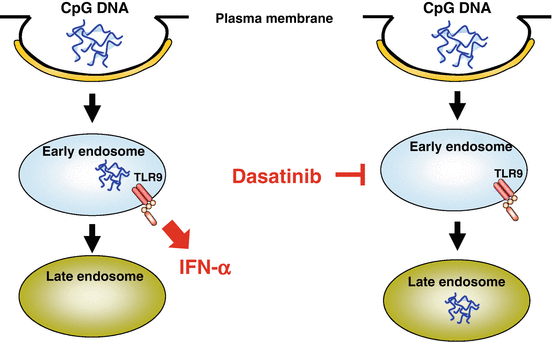
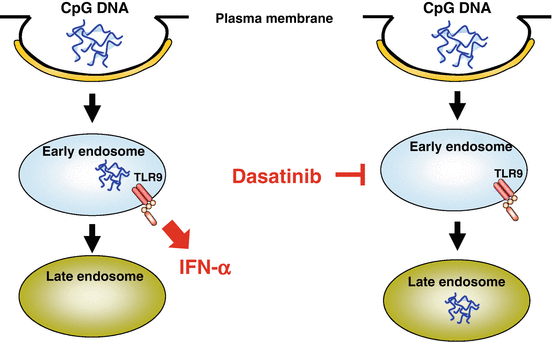
Fig. 12.4
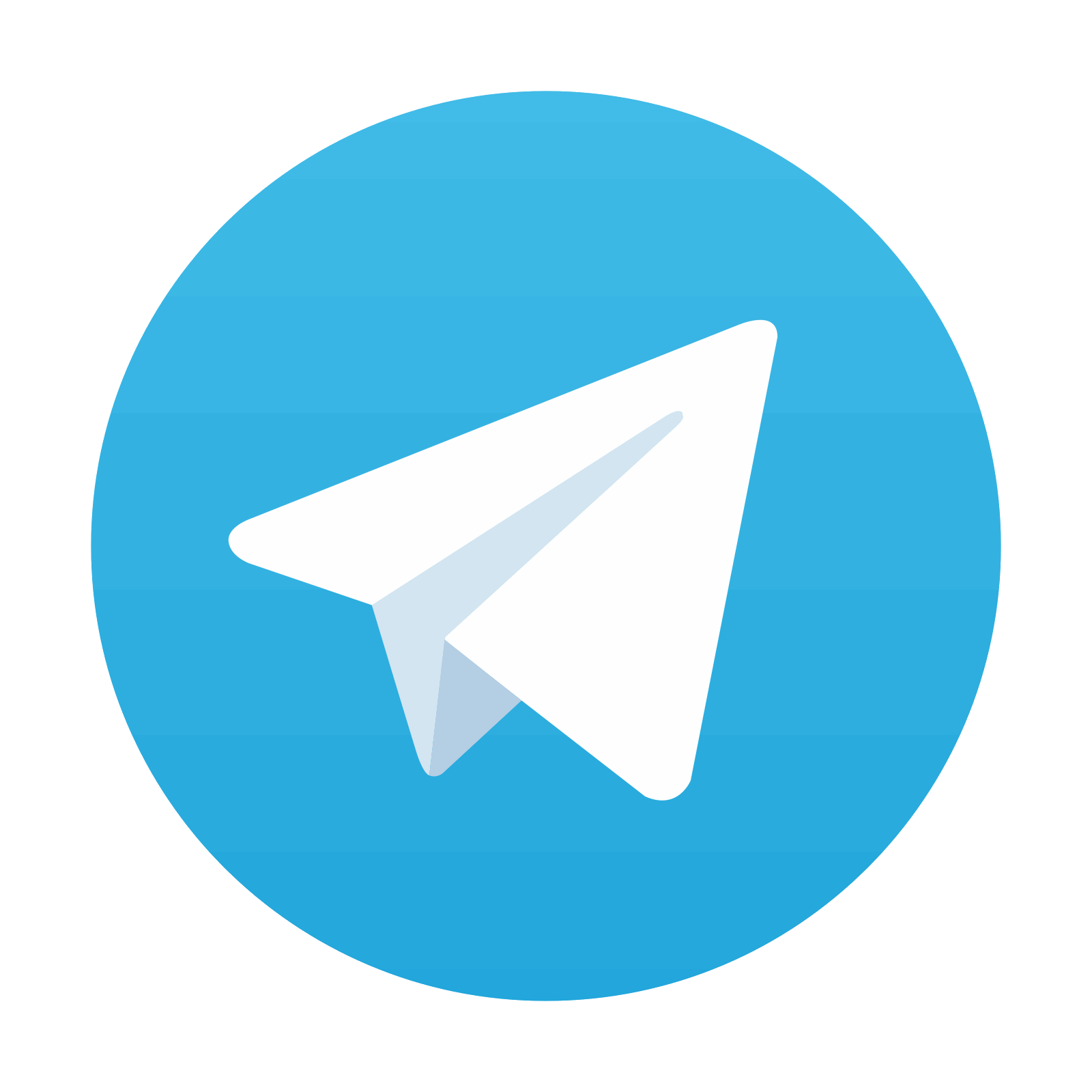
Suppression of plasmacytoid dendritic cells (pDCs) by the tyrosine kinase inhibitor dasatinib. It has been shown that the prodigious production of interferon (IFN)-α depends on prolonged localization of CpG-A in early endosomes. Dasatinib abrogates prolonged localization of CpG-A, which appears to contribute to the suppression of IFN-α production by pDCs
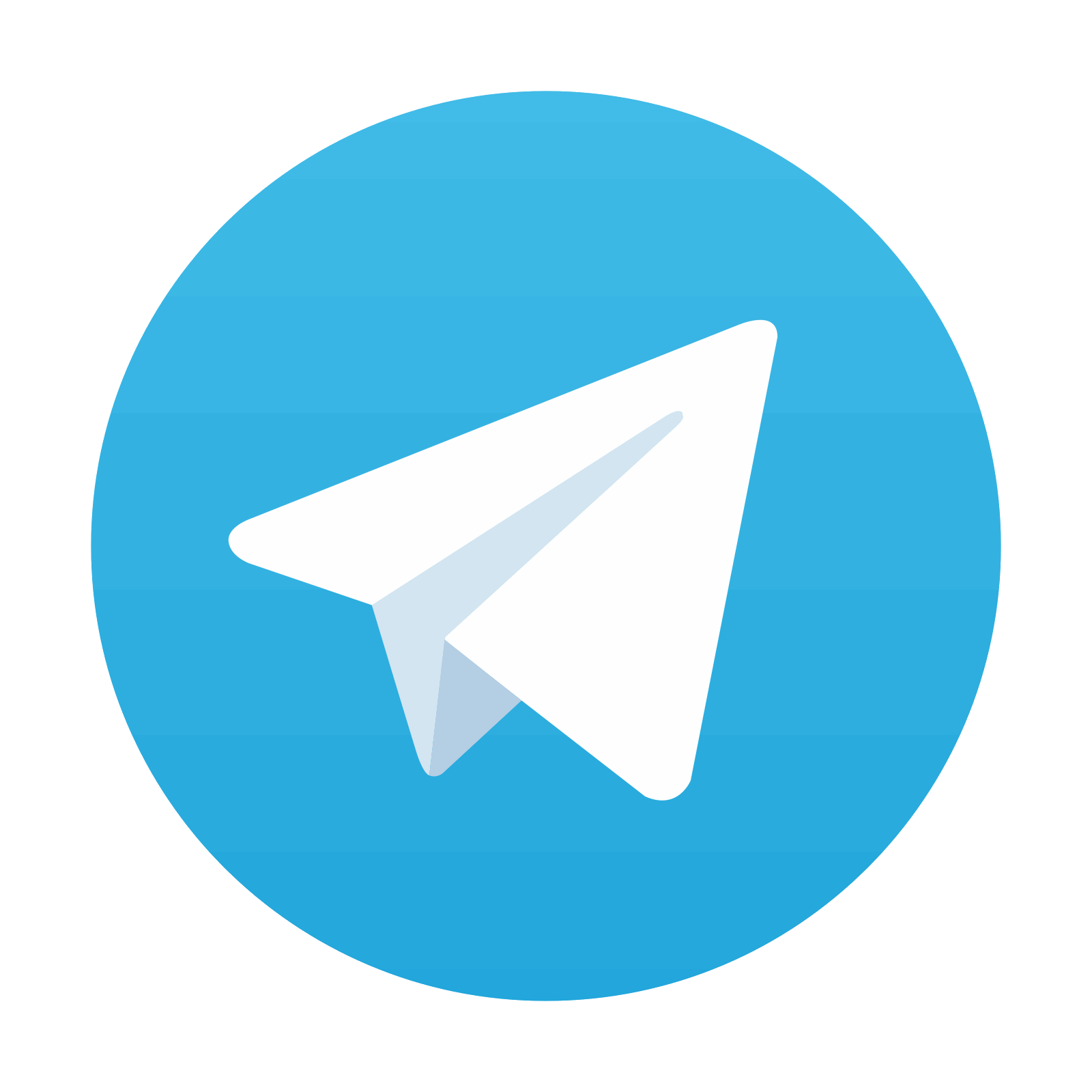
Stay updated, free articles. Join our Telegram channel

Full access? Get Clinical Tree
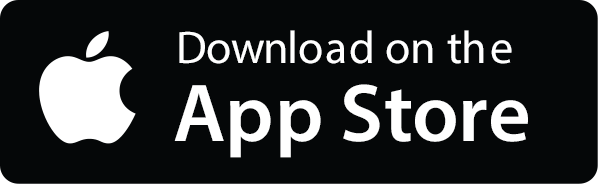
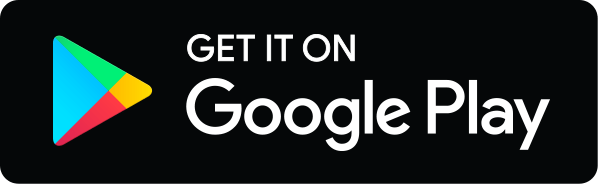
