Fig. 12.1
(a) The locus of enterocyte effacement (LEE) pathogenicity Island found in EHEC, which encodes factors responsible for type III secretion and pedestal formation. LEE1 encodes for ler, the LEE-encoded regulator. LEE1, LEE2, and LEE3 encode for factors involved in type III secretion. LEE4 encodes for EspA, EspB, and EspD. The LEE5/tir operon encodes for intimin and Tir (McDaniel et al. 1995; Kenny et al. 1997a; Mellies et al. 1999)
Chemical signaling through the AI-3/Epinephrine/Norepineprhine signals activates expression of virulence genes in EHEC. Most of these virulence genes are involved in the formation of the AE lesion and are contained within a pathogenicity island named the locus of enterocyte effacement (LEE) (McDaniel et al. 1995) (Fig. 12.2).
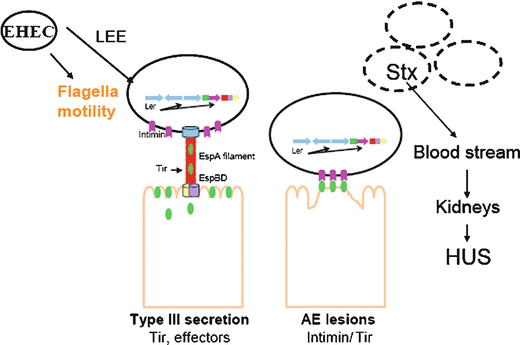
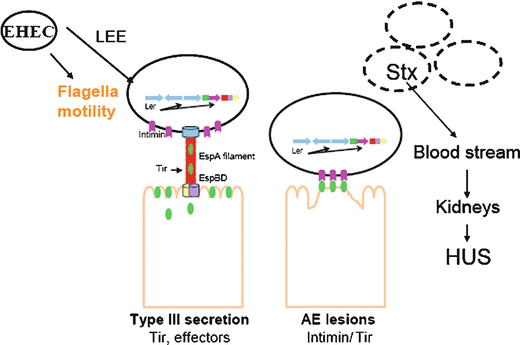
Fig. 12.2
General model for EHEC pathogenesis. LEE region of EHEC encodes most of T3SS effectors, which are essential for EHEC pathogenesis, as well as flagella motility. Ler is master regulator of LEE, intimin is an outer membrane protein, through T3SS EHEC translocates Tir, Intimin-Tir binding culminates in histopathologic lesion called attaching and effacing lesion (or lesion AE). Shiga toxin in EHEC plays late role during infection that can cause hemolictic uremic sindrome (Kaper et al. 2004)
The EHEC LEE region contains 41 genes, most of which are organized into five major operons: LEE1, LEE2, LEE3, LEE5, and LEE4 (Elliott et al. 1998; Elliott et al. 1999; Mellies et al. 1999). The LEE encodes a type III secretion system (TTSS) (Jarvis et al. 1995), an adhesin (intimin) (Jerse et al. 1990), and this adhesin’s receptor, the translocated intimin receptor (Tir) (Kenny et al. 1997b), which is translocated into the epithelial cell through the bacterial TTSS (Elliott et al. 1998; Elliott et al. 1999; Mellies et al. 1999) (Fig. 12.1a).
The TTSS is an apparatus that spans the inner and outer bacterial membranes forming a microscopic “needle.” Several proteins, including EscD, EscR, EscU, EscV, EscS, and EscT span the inner membrane and associate with a cytoplasmic ATPase, EscN, which is required for secretion of proteins (Roe et al. 2003). EscC is predicted to form the main protein ring in the outer membrane to which the EscF “needle” is connected (Wilson et al. 2001). EscF comprises the syringe connected to the filament of the translocon. The translocon consists of EspA, which creates a sheath around the EscF needle. EspB and EspD are located at the distal end of the TTSS and form 3–5 nm pores in the host cell membrane (Ide et al. 2001) through which translocated proteins are secreted.
The eae gene (E. coli attaching and effacing) encodes for intimin, an outer membrane protein that acts as an intestinal adherence factor (Jerse et al. 1990). Mutants of the eae gene are defective in intimate adherence to intestinal epithelial cells, which prevents the concentration of polymerized actin necessary for the development of AE lesions. The translocated intimin receptor (Tir), which is also encoded in the LEE, is translocated from the bacterium through the TTSS into the host cell to serve as a receptor for intimin (DeVinney et al. 1999; Kenny and Finlay 1995; Rosenshine et al. 1996). In the host cell membrane, Tir adopts a hairpin loop conformation and serves as a receptor for the bacterial surface adhesin, intimin (Deibel et al. 1998). Binding of intimin to Tir promotes the clustering of N- and C-terminal cytoplasmic regions and leads to the initiation of localized actin assembly beneath the plasma membrane (Campellone et al. 2004). The EHEC Tir recruits the host protein N-WASP (Goosney et al. 2001) through an interaction with EspFu, another bacterial protein encoded within a prophage, which is also translocated through the TTSS into the host cell (Campellone et al. 2004).
The TTSS encoded by the LEE translocates LEE-encoded and non-LEE encoded effectors. The mitochondrial associated protein, map, affects the integrity of the host mitochondrial membrane (Kenny and Jepson 2000) and is encoded directly upstream of tir. Another effector, EspF, is responsible for the disruption of the intestinal barrier function and induces cell death by an unknown mechanism (McNamara and Donnenberg 1998; McNamara et al. 2001). EspG is responsible for the disruption of microtubule formation and plays a role in virulence in the rabbit enteropathogenic E. coli (REPEC) model (Tomson et al. 2005), while EspH, which is encoded in LEE3, is responsible for the modulation of the host cell cytoskeleton through the inhibition of cell cycle signals (Tu et al. 2003). Although encoded outside the LEE pathogenicity island, several effector proteins have been recently shown to be secreted through the EHEC TTSS (Tobe et al. 2006). These include Cif, which induces host cell cycle arrest and reorganization of host actin cytoskeleton (Charpentier and Oswald 2004), and NleA, which has been shown to localize to the Golgi and play a key role in virulence in an animal model (Gruenheid et al. 2004).
EHEC also produces a powerful Shiga toxin (Stx) that is responsible for the major symptoms of hemorrhagic colitis and HUS. The Stx family contains two subgroups, Stx1 and Stx2. Stx1 shows little sequence variation between strains (Zhang et al. 2002), whereas antigenic divergence has been observed among the Stx2s, including Stx2, Stx2c, Stx2d, and Stx2e (Perera et al. 1988; Schmitt et al. 1991; Zhang et al. 2002). Stx2 has been more associated epidemiologically with severe human disease than Stx1 (Boerlin et al. 1999), with Stx2 and Stx2c being most frequently found in patients with HUS (Ritter et al. 1997).
The genes encoding Stx1 and Stx2 are located within the late genes of a λ-like bacteriophage and are transcribed when the phage enters its lytic cycle (Neely and Friedman 1998). Once the phage replicates, Shiga toxin is produced, and the phage lyse the bacteria, thereby releasing the toxin into the host. The bacteriophage enters its lytic cycle during an SOS response triggered by disturbances in the bacterial membrane, DNA replication, or protein synthesis (Kimmitt et al. 1999; Kimmitt et al. 2000). These triggers are all common targets of conventional antibiotics and may contribute to the controversy surrounding the use of antibiotics to treat EHEC-mediated disease. Shiga toxins consist of a 1A:5B noncovalently associated subunit structure (Donohue-Rolfe et al. 1984). The B subunit of Stx is known to form a pentamer that binds to the eukaryotic glycolipid receptor, globotriaosylceramide (Jacewicz et al. 1986; Lindberg et al. 1987; Waddell et al. 1988). The A subunit is then cleaved by trypsin and reduced, resulting in a polypeptide that causes depurination of a residue in the 28S rRNA of 60S ribosomes (Endo et al. 1988). This leads to the inhibition of protein synthesis, injury of renal glomerular endothelial cells, and the initiation of a pathophysiological cascade that leads to HUS (Fig. 12.2).
12.2 Transcriptional Regulation of the LEE Region
Chemical signaling plays an important role in LEE and flagella expression (Sperandio et al. 1999; Sperandio et al. 2001). The bacterial signal, autoinducer-3 (AI-3), produced by the human intestinal microbial flora, the epinephrine and norepinephrine host stress hormones, as well as the flagella regulon activate expression of the LEE genes (Sperandio et al. 2003). These signals act agonistically to increase LEE gene expression (Walters and Sperandio 2006) Fig. 12.3.
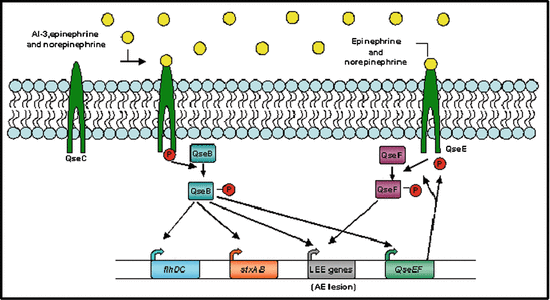
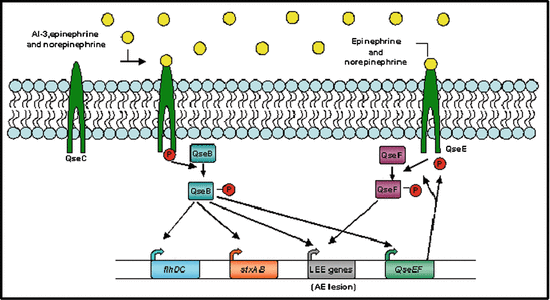
Fig. 12.3
Model for regulation of autoinducer (AI)-3, epinephrine, and norepinephrine (NE) bind the bacterial membrane receptor QseC, which results in its autophosphorylation. QseC then phosphorylates its response regulator QseB and initiates a complex phosphorelay signaling cascade that activates the expression of a second two-component system (QseEF), the locus of enterocyte effacement (LEE) genes, which encode various proteins, including the components of a type III section system that are involved in attaching and effacing (AE) lesion formation, the motility genes (flhDC), and Shiga toxin (stxAB). The QseEF two-component system is also involved in the expression of the LEE genes, and although its activators have not yet been elucidated, it is possible that it senses epinephrine and/or NE (Hughes and Sperandio 2008)
In addition to the regulation via stress hormones, other transcription factors are involved on LEE regulation. Iyoda and Watanabe (2004) have observed that EHEC encodes the genes pchA, pchB, and pchC (PerC homologs) that positively activate the expression of the LEE genes (Iyoda and Watanabe 2004). LEE1 encodes for Ler, the LEE-encoded regulator, which was shown to be required for the expression of all genes within the LEE (Bustamante et al. 2001; Elliott et al. 2000; Kaper et al. 2004; Mellies et al. 1999). Another important factor in the regulation of the LEE is the integration-host factor, or IHF. IHF has been shown to be required for the expression of the entire LEE through the direct activation of ler transcription (Friedberg et al. 1999). Additionally, EtrA and EivF are two negative regulators of the LEE region, acting possibly through ler transcriptional repression. The etrA and eivF genes are found within a second pathogenicity island that encodes a cryptic type III secretion system (ETT2) (Zhang et al. 2004). The histone-like nucleoid-structuring protein, H-NS, is responsible for the repression of LEE2, LEE3, and LEE5 transcription in the absence of Ler (Bustamante et al. 2001; Haack et al. 2003). RpoS, a stationary phase sigma factor, activates the transcription of the LEE3 operon (Sperandio et al. 1999). Finally, Hha has been reported to repress the transcription of the LEE4 operon (Sharma and Zuerner 2004).
Two previously uncharacterized genes in the LEE region, orf10 and orf11, were recently renamed GrlR, global regulator of LEE repressor, and GrlA, global regulator of LEE activator (Barba et al. 2005; Deng et al. 2004). This study suggested that GrlA is responsible for the transcriptional activation of ler, while GrlR represses ler. Additionally, it is known that Ler activates the transcription of grlRA (Barba et al. 2005; Elliott et al. 2000) and that GrlRA activates the expression of LEE2 and LEE4, independently of Ler (Russell et al. 2007).
12.3 Quorum Sensing in EHEC
Surette and Bassler reported in 1998 quorum sensing signaling in E coli K12 and S. enterica Typhimurium (Surette and Bassler 1998) through the production of autoinducer-2 (AI-2) by these bacteria (Surette et al. 1999). A common gene on these bacterial species was cloned and identified as responsible for AI-2 production, and it was named luxS (Surette et al. 1999). Later on, LuxS was characterized as the enzyme involved in the metabolism of S-adenosylmethionine and shown to convert ribose–homocysteine into homocysteine and 4,5-dihydroxy-2,3-pentanedione, which is the precursor of AI-2 (Schauder et al. 2001). Currently, the autoinducer referred to as AI-2 is a furanosylborate-diester in Vibrio harveyi (Chen et al. 2002), and a 2R, 4S-2-methyl-2,3,3,4-tetrahydrofuran (R-THMF) for Salmonella sp.(Miller et al. 2004).
However, at that time, no phenotypes have been shown to be regulated by quorum sensing in E. coli and Salmonella sp. In 1999, Sperandio et al. (1999) described that quorum sensing signaling activated transcription of the LEE genes and type three secretion in pathogenic E. coli, both in EHEC and in enteropathogenic E. coli (EPEC). It was then proposed that signaling through AI-2 might be involved in virulence gene regulation in E. coli. In 2001, quorum sensing was shown to be a global regulatory mechanism in EHEC, involved in AE lesion formation, motility, metabolism, growth, and Shiga toxin expression (Sperandio et al. 2001). The EHEC’s quorum sensing regulatory cascade started to be unraveled with the description of the first three quorum sensing regulators (Sperandio et al. 2002a; Sperandio et al. 2002b), which were named Quorum sensing E. coli, Qse regulators. QseA was reported as a transcription factor from the widespread LysR family, directly involved in activation of LEE1 (ler) transcription and EHEC’s pathogenesis (Russell et al. 2007; Sharp and Sperandio 2007; Sperandio et al. 2002a). The QseBC regulatory system was described as a novel two-component system involved in quorum sensing regulation of flagella expression and motility (Clarke and Sperandio 2005b; Sperandio et al. 2002b).
A year later, it was shown that AI-2 was not the autoinducer involved in EHEC virulence regulation. The signal identified was a yet undescribed autoinducer, which was named autoinducer 3 (AI-3). In addition, it was reported that the eukaryotic hormones epinephrine and/or norepinephrine could substitute for the bacterial AI-3 signal to activate expression of the EHEC virulence genes. Among them, ler, the master regulator of LEE, was shown to be activated in presence of AI-3. Moreover, flagella motility of EHEC regulated via QseBC system was demonstrated to be affected by AI-3. Given that eukaryotic cell-to-cell signaling occurs through hormones, like epinephrine and norepinephrine, this cross talk between bacteria and host seem to happen through cross-signaling between quorum sensing signals and host hormones (Sperandio et al. 2003).
12.4 Infectious Disease and Hormones
Since the 1920s, hormones have been used for treatment of a variety of illnesses (Yamashima 2003). Following studies have shown the role of stress hormones directly on bacterial functions.
Catecholamines, such as epinephrine and norepinephrine, are chemical compounds derived from the amino acid tyrosine containing catechol and amine groups. They are stress hormones involved in the fight or flight response. They are synthesized from l-dopa into dopamine, then norepinephrine, and epinephrine. Both norepinephrine and dopamine containing sympathetic nerve terminals are distributed throughout the body, including the intestinal tract, where they are part of the enteric nervous system. Epinephrine synthesis is restricted to the central nervous system and adrenal glands. However, epinephrine is released into the bloodstream, especially during stress, acting systemically in the whole body (Furness 2000).
Both epinephrine and norepinephrine are recognized by adrenergic receptors in mammalian cells. Adrenergic receptors are a subset of the G-protein coupled receptors (GPCRs) family, which are transmembrane receptors coupled to heterotrimeric guanine-binding proteins (G proteins). Adrenergic receptors are divided in two main subtypes: α and β. Both epinephrine and norepinephrine are recognized by a very similar ligand-binding pocket in all adrenergic receptors (Cherezov et al. 2007; Freddolino et al. 2004).
12.5 QseC: A Bacterial Functional Analog of an Adrenergic Receptor
Unlike mammalian cells, EHEC does not encode a GPCR receptor in its genome; hence, sensing of adrenergic hormones by this bacterium was occurring through another type of receptor. The main signaling transduction systems in bacterial cells are the two-component systems. In these systems, the sensor for environmental cues is a histidine kinase (usually membrane bound), which upon autophosphorylation transfers its phosphate to an aspartate residue in the response regulator. This response regulator is usually a transcription factor that is activated by phosphorylation. QseC is a histidine sensor kinase that augments its phosphorylation specifically in response to the AI-3 bacterial quorum sensing signal and the host hormones epinephrine and/or norepinephrine (Clarke et al. 2006). Importantly, tritiated norepinephrine was shown to specifically bind to QseC, and this binding could be antagonized only by a λ-adrenergic antagonist (phentolamine), while a β-adrenergic antagonist (propranolol) did not have any effect on QseC activity (Clarke et al. 2006). QseC then transfers its phosphate to its cognate response regulator QseB, which only upon phosphorylation binds to its target promoters to regulate gene expression (Clarke et al. 2006; Clarke and Sperandio 2005a; b).
12.6 QseBC Two-Component System
The QseBC system was initially described as a two-component system regulated by quorum sensing, which shares homology with Salmonella enterica serovar Typhimurium PmrAB (Sperandio et al. 2002b). The same study showed that QseBC was involved in regulation of flagella and motility in EHEC.
In a detailed study (Clarke and Sperandio 2005a), it was shown that QseBC constituted a two-component system, and that the qseBC genes were cotranscribed forming an operon. Moreover, it was reported that QseB autoactivated transcription of the qseBC operon (Clarke and Sperandio 2005a). Using primer extension, the start site of the qseBC transcript was mapped, and through nested deletion analysis it was determined the minimal region necessary for QseB transcriptional autoactivation. Also, electrophoretic mobility shift assays, competition experiments, and DNAse I footprints showed that QseB directly binds to 2 sites in its own promoter.
Additionally, Clarke and Sperandio (2005b) described that QseBC regulates the flagella expression and motility through flhDC, the master regulator of flagella. Using electrophoretic mobility shift assays, competition experiments, and DNAse I footprints, it was shown that QseB directly binds to the flhDC promoter both in low and high affinity binding sites. In this study, it was also reported that the promoter of flhDC responsive to QseBC had a σ 28 consensus. In summary, these studies suggested that transcription of the flhDC promoter by QseBC is a complex system and is dependent on the presence of FliA (σ 28).
QseC’s homologs are found at least in 25 species of bacteria, including animals and plant pathogens (Table 12.1), suggesting that this signaling system is not restricted to E. coli (Rasko et al. 2008). A few recent reports have implicated these non-EHEC qseC homologues in virulence gene activation presumably through a combination of AI-3, norepinephrine, and epinephrine activation. The chemical structure of AI-3 was not completely elucidated yet. However, the role of epinephrine and norepinephrine has been extensively reported in different strains of E. coli, Salmonella, Staphylococcus, among others. A qseC homologue was shown to contribute to virulence in a mouse infection model of the class A bioterrorism threat agent Francisella tularensis (Weiss et al. 2007). A qseC homologue was also demonstrated to be involved in norepinephrine dependent enhancement of motility and colonization of juvenile pigs by S. enterica serovar Typhimurium (Bearson and Bearson, 2008) and virulence gene expression in this bacterium (Merighi et al. 2006; Merighi et al. 2009).
Table 12.1
Some of bacterial genera and species where QseBC (Rasko et al. 2008) and QseEF two-components systems are conserved, other than EHEC
QseBC | QseEF |
---|---|
Enteropathogenic E. coli | Uropathogenic E. coli |
Enteroaggregative E. coli | E. coli k12 |
E. coli k12 | S. enterica Typhimurium |
Salmonella | Salmonella enterica Typhi |
Vibrio | Salmonella enterica Paratyphi |
Shigella | Shigella flexneri |
Enterococcus | Shigella boydii |
Campylobacter | Shigella dysenteriae |
Yersinia | Shigella sonnei |
Psychrobacter | Bacillus subtilis |
Fransciella | Bacillus amyloliquetaciens |
Erwinia carotovora | |
Hemophilus influenzae | |
Pasteurella multocida | |
Actinobacillus pleuropneumoniae | |
Chromobacterium violaceium | |
Rubrivivax gelatinosus | |
Thiobacillus denitrificans | |
Ralstonia eutropa | |
Ralstonia metallidurans |
12.7 The QseEF Two-Component System
Recently, a second two-component system in the AI-3/epinephrine/norepinephrine signaling cascade was described by Reading et al. (2007) and named QseEF. The qseE and qseF genes are part of a polycistronic operon that also contain in this cluster the yfhG gene, which encodes for uncharacterized protein, and glnB, which encodes the PII protein involved in nitrogen regulation (Reading et al. 2007). Transcription of qseEF is activated by epinephrine through QseC, suggesting that QseEF comes second to QseBC in the hierarchy of gene expression in this signaling cascade.
In this system, QseE is the sensor kinase, and QseF the response regulator. QseF activates transcription of the recently described gene encoding EspFu (Reading et al. 2007), an effector protein of EHEC, which is encoded outside the LEE region. EspFu is translocated into the host cell by EHEC where it mimics an eukaryotic SH2/SH3 adapter protein to engender actin polymerization during pedestal formation (Campellone et al. 2004). In silico analysis indicates that QseF contains a σ 54 activator domain. However, the espFu gene contains a conserved extended σ 70 consensus promoter region, suggesting that QseF indirectly activates transcription of espFu. In agreement to this hypothesis, eletrophoretic mobility shift experiments demonstrated that QseF does not bind to the espFu promoter region. These data showed that QseF-dependent activation of espFu transcription is indirect and involves an intermediary factor transcribed in a σ 54-dependent fashion.
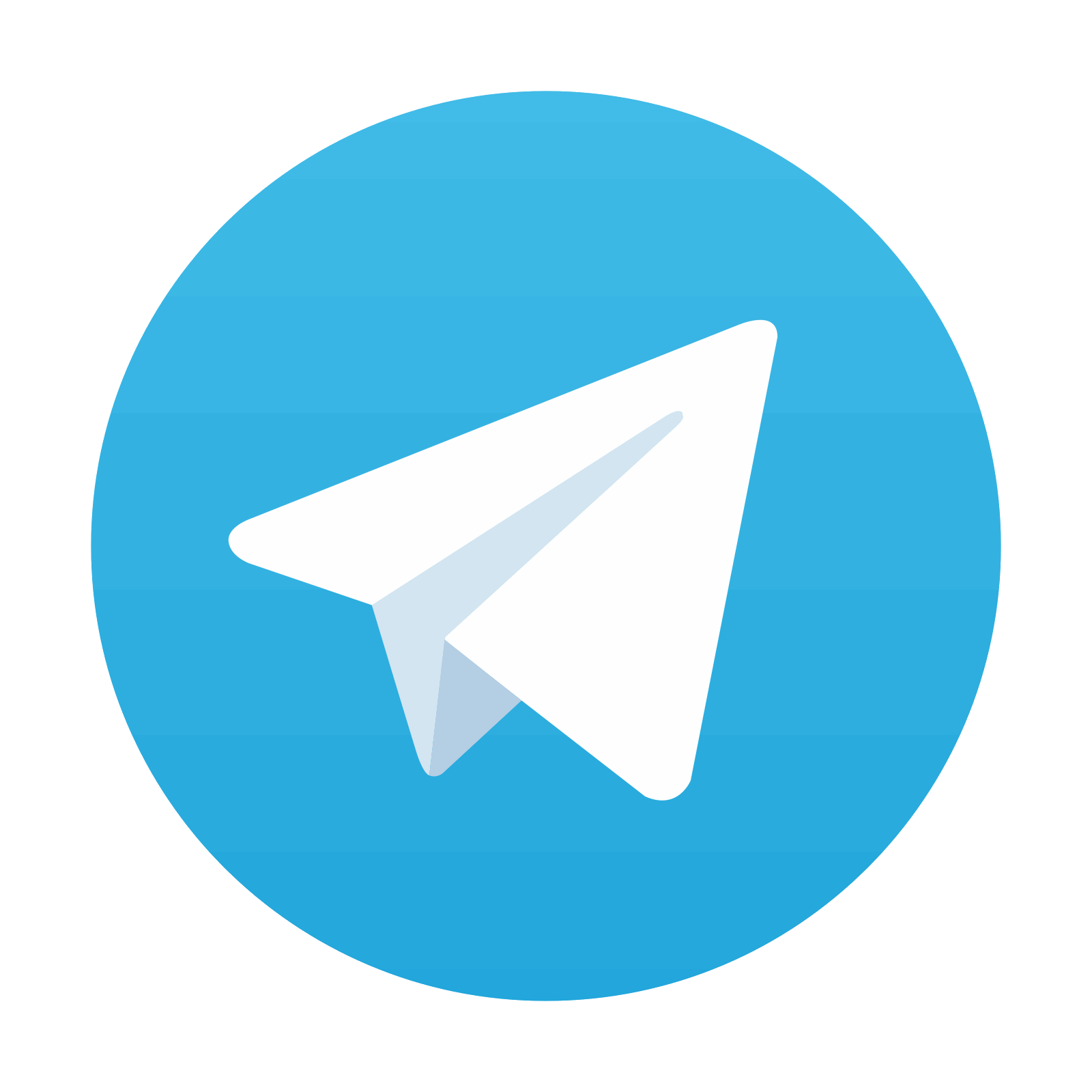
Stay updated, free articles. Join our Telegram channel

Full access? Get Clinical Tree
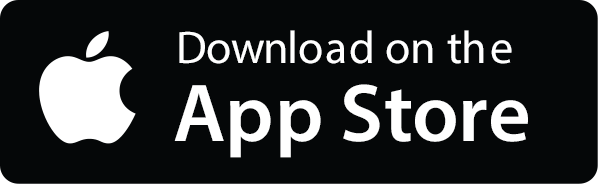
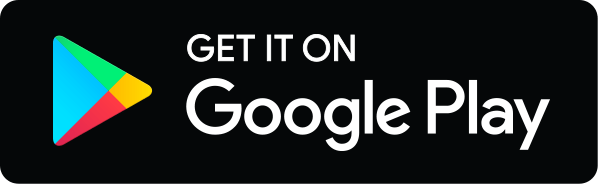