Fig. 11.1
Aerobically grown lactobacilli were enumerated from coprocultures before and for 1 week following maternal separation. Results are mean (S.E.) number of colony forming units (CFU) per gram of fecal matter (wet weight). *p < 0.05 versus preseparation values. Reproduced from Developmental Psychobiology, 1999 with permission from Wiley
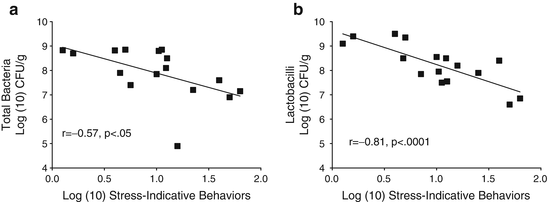
Fig. 11.2
Log transformed stress-indicative behaviors were significantly associated with log(10) CFU/g of intestinal microflora. (a) Total aerobic and facultatively anaerobic microflora. (b) Aerobically grown lactobacilli. Reproduced from Developmental Psychobiology, 1999 with permission from Wiley
Lactic acid bacteria, such as members of the genus Lactobacillus, are thought to be important contributors to microflora-mediated colonization exclusion. Thus, stressor-induced reductions in lactobacilli would be hypothesized to be associated with enhanced susceptibility to enteric infection. In this experiment, none of the monkeys were intentionally infected, but many nonhuman primate colonies have endemic levels of enteric pathogens, notably Shigella flexneri and Campylobacter jejuni. And, 45% (i.e., 9/20) of the infant monkeys became colonized with either S. flexneri or C. jejuni during the week following maternal separation. On the first day that pathogen colonization was observed there was a weak, marginally significant (p = 0.07) inverse association between the number of lactobacilli and pathogens shed from the intestines (Bailey and Coe 1999). These data are consistent with the idea that lactobacilli are important in colonization resistance against enteric pathogens, but further studies are needed to conclude that stressor-induced alterations in microflora result in increased susceptibility to enteric infection.
Stressor-induced reductions in lactobacilli have also been found in college students during stressful periods (Knowles et al. 2008). In this study, lactobacilli levels were determined during a low stress period (i.e., the first week of the semester) and a high stress period (i.e., final exam week). The exam period was associated with significantly higher levels of perceived daily stress and weekly stress, as well as an increase in GI upset. Moreover, when compared to the low stress period, levels of lactic acid bacteria, primarily lactobacilli, shed in the stool were significantly lower for up to 5 days following examination, with differences in bacterial levels reaching one half log unit in magnitude (e.g., baseline values of 6 × 107 CFU/ml vs. 1 × 107 on day 5 post-examination). It should be noted, however, that significant differences in diet did occur across the two time periods; most notable were significant reductions in vegetable consumption and a significant increase in coffee consumption (Knowles et al. 2008). But, given that stressor exposure alters lactobacilli levels in laboratory animals fed a standardized diet, it is likely that stress-associated changes in human microflora reflect an impact of the stressor as well as potential effects of diet.
Healthy adults are somewhat resistant to the impact of stressors on various physiological systems. For example, stressor-induced alterations in the immune response tend to return to baseline upon termination of the stress response. However, the stress response can have a more prolonged effect on immunity in the very old and the very young (Coe and Lubach 2003). And, stressor exposure in the very young, or even during the prenatal period, is thought to set the infant on a significantly different developmental trajectory, resulting in larger stressor induced effects later in life (Coe and Lubach 2003). One of the most consistent findings in regards to exposure to prenatal stressors is that fetal growth and birth weight are reduced after women experience stressful situations during pregnancy (Field et al. 1985; Lederman et al. 1981; Lederman 1986). Rhesus monkeys have been used extensively to investigate the influence of prenatal stress on infant development. And, it has been shown that prenatal stress affects nueromotor development (Schneider and Coe 1993), emotional reactivity to stressors (Clarke and Schneider 1993), brain monoamine levels (Schneider et al. 1998), cell density in the brain (Coe et al. 2002, 2003), and immune reactivity (Coe et al. 1996, 1999, 2007). Our studies focused on the impact of gestational stress on the intestinal microflora across the four phases of microflora development.
Bacteria colonize the GI tract of newborns in a sequential pattern that is tightly related to developmental milestones in the infant (Cooperstock and Zed 1983). The first phase of colonization begins at birth when bacteria from the mother’s reproductive tract colonize the otherwise sterile newborn. These bacteria do not predominate for long and are quickly overcome by maternal aerobic intestinal microflora, which are thought to persist in the intestines for the first few days of life (Tannock et al. 1990). These aerobic species, such as E. coli and Streptococcus spp. consume molecular oxygen as they grow and begin to reduce the oxidation–reduction potential in the intestines creating a more favorable environment for the growth of anaerobic species (Meynell 1963). As a result, high levels of Enterobacteriaceae are evident 1 day after birth, but anaerobes, such as bifidobacteria, predominate by 6 days of age and throughout the period of exclusive breast feeding (Sakata et al. 1985).
Members of the genus Bifidobacterium thrive in breastfed infants and are the predominant bacteria in the intestines due to growth factors found in human milk that bifidobacteria readily use for energy, such as lactose. As bifidobacteria grow, they produce pronounced levels of lactic and acetic acids that can not be buffered by human milk, thus inhibiting the growth of acid sensitive microbes. Breast milk also contains large amounts of immune factors, such as secretory immunoglobulins, lactoferrin, lysozymes, and even leukocytes that can inhibit colonization of certain bacteria (Balmer and Wharton 1991; Wharton et al. 1994a, b). The combination of immune factors and acidic fermentation products gives bifidobacteria a tremendous ecological advantage over other species (Heine et al. 1992; Beerens et al. 1980).
The initiation of weaning from breast milk is associated with a resurgence of aerobic and facultatively anaerobic species, such as E. coli, Streptococci, and Clostridia spp., that are naturally found in newly ingested foods. The concentrations of these newly arrived bacteria fluctuate greatly during this period, but as the diet becomes more consistent, microbial populations in the intestines also stabilize and will remain quite stable throughout the lifespan. This stability is important for maintaining intestinal homeostasis (O’Hara and Shanahan 2006), and if disrupted could contribute to the development of GI infections or cancers (O’Hara and Shanahan 2006).
11.5 Prenatal Stressor-Induced Alterations to Microflora Development
To determine the impact of a prenatal stressor on microflora development, an acoustical startle stressor (i.e., 3 random 110 dB beeps over a 10 min period occurring 5 days per week) was used to evoke a stress response from pregnant rhesus monkeys either early (days 50–92) or late (days 105–147) in the 169 day gestational period. These periods represent crucial time periods in nervous system and GI system development, thus making it likely that disruption of physiological homeostasis at these time points affects fetal development. This stressor resulted in a significant increase in cortisol in the pregnant mothers, but did not appear to significantly affect the number of miscarriages, gestational length, or birth weight (Bailey et al. 2004b). The stressor did, however, significantly affect the development of the intestinal microflora.
During the first 6 months of life, lactobacilli levels in the monkeys born from mothers exposed to the stressor during gestation were significantly lower than levels found in infants from non-stressed control mothers, with the biggest differences in mean levels found at 2 weeks of age (Fig. 11.3). As successful nursing progressed, bifidobacteria began to predominate in the intestines. And, as with the lactobacilli, bifidobacteria levels were significantly lower in the intestines of infant monkeys from mothers that were exposed to the acoustical startle stressor during gestation. This effect, however, was only evident in the offspring from mothers exposed to the stressor late in gestation (Fig. 11.3) (Bailey et al. 2004b). As with the previous study involving maternal separation, none of the monkeys in this study were intentionally infected with enteric pathogens. However, approximately 43% of the infants from mothers stressed early in gestation and 12% of infants from mothers stressed late in gestation became subclinically colonized with Shigella flexneri, an endemic pathogen in the monkey colony. Importantly, Shigella were not detected in any of the infants born from the non-stressed control condition (Bailey et al. 2004b), suggesting that prenatal stress, particularly late in gestation, disrupted the development of natural resistance to the enteric pathogen, S. flexneri.
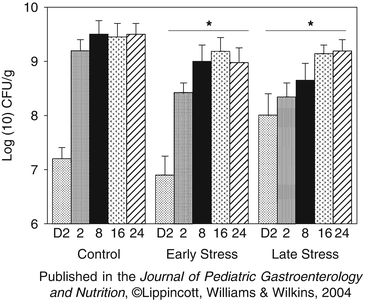
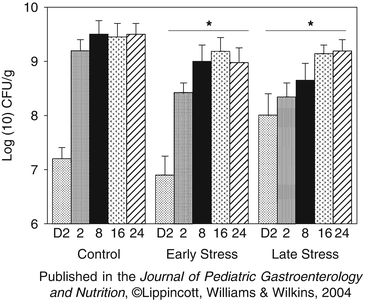
Fig. 11.3
Anaerobically grown Lactobacillus spp. during the first 24 weeks of life. Data are the mean (SE) of log(10) transformed number of colony forming units per gram of fecal matter (CFU/g). Concentrations on day 2 of life were not significantly different between pregnancy conditions. * Both Early Stress and Late Stress infants had significantly fewer anaerobic lactobacilli than did control infants across the first 24 weeks of life (p < 0.05). In addition, there was a developmental trend for increasing titers across the 24 week period in both control and prenatally stressed infants (p < 0.05). Reproduced from Journal of Pediatric Gastroenterology and Nutrition, 2004, with permission from Lipincott, Williams, & Wilkins
11.6 Psychological Stress and the GI Tract: Toward a Mechanism of Stressor-Induced Alterations in Microflora
It is tempting to speculate on the mechanisms through which the intestinal microflora could have been altered by stressful pregnancy conditions. For example, it is known that cortisol can affect many aspects of infant development, and many of the effects of prenatal stress on the immune system can be mimicked by administration of ACTH or the synthetic glucocorticoid, dexamethasone (Coe et al. 1996). And, others have found that giving corticosterone to pregnant rats significantly reduced the concentrations of total and Gram-negative aerobes and facultative anaerobes (Schiffrin et al. 1993). The mechanisms through which glucocorticoids might affect the microflora are not known, but fetal development of the gi tract is thought to be influenced by glucocorticoids. For example, maturation of the intestines occurs concomitantly with the prepartum surge in cortisol in precocial species, such as pigs, sheep, and humans (Trahair and Sangild 1997). Moreover, very high levels of glucocorticoids adversely affect intestinal development, such as the ability to secrete gastric acid and the densitivity of villi and crypts (Sangild et al. 1994), thus changing the microenvironment in the intestines and opening the possibility of shifts in ecological competition.
Altering the microenvironment may also affect established microflora populations in older hosts. The complete set of factors controlling the types of bacteria that can reside as part of the intestinal microflora are not well understood, but it is thought that the host plays a role in “selecting” the microflora. This was elegantly demonstrated by Rawls et al. (2006), who reciprocally transplanted gut bacteria between mice and zebrafish. After transplantation, gut microbial populations shifted to reflect the proportions of bacteria found in the microflora of conventionally reared recipient animals, and no longer reflected the microflora of the original donor animal (Rawls et al. 2006). This may in part be due to the physiology of the host GI tract, since certain aspects of GI physiology are known to influence microbial populations in the GI tract. For example, it is well known that for bacteria to take up residence in the gi tract, they must first survive the low pH of the stomach. Therefore, it is not surprising that reduced production of gastric acid (as occurs with hypochlorhydria) results in overgrowth of bacteria in the gi tract (Drasar et al. 1969). Some species, however, such as members of the genera Lactobacillus and Bifidobacteria are acid tolerant and are able to grow in the low pH (Drasar et al. 1969). This acid tolerance gives the genera an ecological advantage over other species that compete to colonize the gi tract. Therefore, a logical hypothesis is that any stimulus that disrupts gastric acid production will in turn affect intestinal microflora levels.
The influences of emotional states on the secretion and motility of the GI system were documented as early as 1833, when the surgeon William Beaumont noted that the secretion of gastric juice was decreased or abolished during periods of anger or fear in his patient with a gastric fistula (Beaumont 1838). Experimental data has confirmed this observation, and it is now known that secretion of gastric acid can be suppressed by experimental stressors, such as the cold pressure task and mental arithmetic (Badgley et al. 1969; Holtmann et al. 1990). In animals, different stressors have differential effects on acid secretion, with restraint stress reported to significantly increase or decrease gastric acidity depending upon temperature (Murakami et al. 1985; Lenz et al. 1988). These differences are due to different levels of activation of the sympathetic and parasympathetic nervous systems; activating the SNS suppressed whereas activating the PNS enhanced acid secretion (Yang et al. 2000). Research is needed to determine whether gi acidity plays a role in stressor-induced alterations of microflora.
There are, of course, additional secretory products that can affect microflora levels and are themselves influenced by the stress response such as additional digestive products like bile, and immune products like secretory immunoglobulin A (sIgA) and antimicrobial peptides. The use of secretory immunoglobulin deficient mice has shown the importance of this immunoglobulin in influencing microbial populations; sIgA deficient mice have significantly increased populations of anaerobic microflora in the small intestine (Fagarasan et al. 2002). Moreover, antimicrobial peptides, such as the defensins, have been suggested to modify the types and numbers of bacteria colonizing the GI tract (Salzman et al. 2007). Because these molecules can be affected upon exposure to a stressor (Jarillo-Luna et al. 2007; Korneva et al. 1997), an additional plausible hypothesis is that stress-associated alterations of the microflora are dependent upon stressor-induced alterations in sIgA and/or defensins.
Perhaps the most well-studied effects of stress on the gi tract are the effects on GI motility. Animal models have established that stress reduces gastric emptying (Taché et al. 2001; Nakade et al. 2005) and slows transit in the small intestine (Lenz et al. 1988; Kellow et al. 1992) through stressor-induced elevations of corticotrophin releasing hormone (Taché et al. 2001; Nakade et al. 2005). In contrast to the inhibitory effects in the stomach and small intestine, stress tends to enhance motility in the colon due to increased sacral parasympathetic outflow to the large intestine through a CRH dependent circuit (Lenz et al. 1988; Martinez et al. 1997).
Gastrointestinal motility has long been thought to influence microbial populations in the GI tract. For example, slowing peristalsis, and thus motility, by administering high doses of morphine causes significant bacterial overgrowth in the small intestines of rats (MacFarlane et al. 2000; Scott and Cahall 1982). Moreover, data from humans show an association between surgical trauma, stagnation of intestinal motility, and bacterial overgrowth, thus supporting the notion that delayed intestinal motility can result in bacterial overgrowth (Marshall et al. 1988; Nieuwenhuijzen et al. 1996a, b). Interestingly, increased GI motility can also affect microflora levels, with some studies showing a direct correlation between small intestine microflora levels and the rate of peristalsis.
An equally likely explanation is that the intestinal microflora were directly affected by stressor-induced increases in intestinal hormones, such as NE. The primary focus of this book is the exciting finding that bacteria can change their growth characteristics when exposed to hormones. And, the growth of many types of microflora has been shown to be significantly enhanced upon culture with NE (Freestone et al. 2002). Despite the many studies showing bacterial growth enhancement by NE in vitro, demonstrating that these interactions occur in vivo has been challenging. Neuroendocrine–bacterial interactions, however, undoubtedly occur in vivo when NE levels reach high levels. This was evident with the use of the neurotoxin 6-hydroxydopamine, which lyses the nerve terminals of sympathetic neurons resulting in the release of NE that is stored in the nerve terminals (Lyte and Bailey 1997). Thus, even though 6-OHDA is a useful way to chemically sympathectomize laboratory rodents, its initial effect is the release of a large bolus of NE 24 h after injection (Porlier et al. 1977; De Champlain 1971). Interestingly, bacterial levels in the cecums of mice were found to be significantly increased 24 h after administration of 6-OHDA, with E. coli showing the greatest increase (Lyte and Bailey 1997). Since the growth of commensal E. coli is strongly affected by exposure to NE (Freestone et al. 2002), the data suggest that overgrowth of E. coli in the cecums of chemically sympathectomized mice results from direct enhancement of bacterial growth by NE.
Overgrowth of bacteria in the family Enterobacteriaceae is also evident in the intestines of mice exposed to psychological stressors. Our recent studies indicate that restraining mice for prolonged periods (i.e., 16 h per day for 7 days) result in an overgrowth of Enterobacteriaceae in both the small and large intestines (Bailey et al. manuscript under review) as well as in the cecum (Bailey et al. 2006). This overgrowth may have important health implications, since bacterial overgrowth is a precipitating factor in the translocation of bacteria from the gi tract to the rest of body. In fact, the translocation of some species in the family Enterobacteriaceae was found to be directly related to levels in the small intestine and cecum (Steffen and Berg 1983). The finding that exposing mice to psychological stressors can enhance E. coli levels in the intestines prompted the determination of the impact of psychological stressors on bacterial transloction.
11.7 Stressor-Induced Bacterial Translocation
Indigenous microflora are not invasive bacteria, which is one property that allows them to reside with their host. Moreover, the external surfaces of the body, i.e., cutaneous and mucosal surfaces, maintain a barrier to external substances, including microbes. In mucosal tissues, transport of solutes into the body is controlled in part through tight junctions between intestinal epithelial cells that prevent the passive transfer of molecules and microbes. Bacteria from mucosal surfaces, however, are routinely sampled by specialized phagocytic cells, called M cells, which engulf mucosal bacteria and pass them to regional lymph nodes in order to initiate an immune response or to maintain tolerance. Most of these bacteria are killed en route, resulting in low to undetectable levels of culturable bacteria in regional lymph nodes.
The epidermal layer of the skin is also well known to provide a permeability barrier that primarily serves to prevent water loss in a potentially desiccating environment. This barrier, however, is also a potent barrier to the passage of cutaneous microflora into the body. As a result, normal mice rarely have detectable levels of bacteria in lymph nodes that drain cutaneous surfaces. In our studies, less than 15% of non-stressed control mice were found to have bacteria in the inguinal lymph nodes that lie under skin in the lower back (Bailey et al. 2006). This percentage was significantly increased when mice were exposed to prolonged restraint or the social stressor, social disruption (SDR), with 82% of mice in both groups identified as having bacteria in these draining lymph nodes. To try to determine whether this effect could simply be due to mechanical breaches in the skin (such as from biting during SDR, or abrasions from the restraint tube), a separate group of mice received full thickness skin biopsies on the lower back. Interestingly, only 36% of mice in this group were found to have bacteria in the inguinal lymph nodes, which was significantly less than the 82% occurrence in the stressed animals (Bailey et al. 2006). These data indicate that the stress response, rather than mechanical barrier breaches, is responsible for the bacterial translocation of cutaneous microflora.
The percentage of mice with bacteria cultured from mesenteric lymph nodes, which drains the GI tract, was higher than the percentage of mice found to have bacteria in the inguinal lymph nodes. We found that 48% of non-stressed control mice were culture-positive for bacteria in the mesenteric lymph nodes. However, exposure to either restraint or SDR increased the occurrence of bacteria in the mesenteric lymph nodes to over 80% (i.e., 82% of SDR mice were culture positive; 91% of restrained mice were culture positive). Interestingly, depriving the mice of food and water did not significantly affect the translocation of indigenous microflora, indicating that the stress response, rather than other physiological variables significantly enhanced bacterial translocation in the gut (Bailey et al. 2006).
There are now several reports indicating that barrier defenses in both the skin and the GI tract can be disrupted by exposure to psychological stressors. Acute experimental stressors in human participants, such as a public speaking tasks and sleep deprivation, were shown to disrupt the permeability barrier in the skin as determined by measuring transepidermal water loss (TEWL) and by determining the water content of the outermost layer of the skin, i.e., the stratum corneum (Altemus et al. 2001). In mice, TEWL was also found to be affected by exposure to different housing conditions and by immobilization stress (Denda et al. 2000). This effect was later found to be due to the impact of glucocorticoid hormones on the stratum corneum (Choi et al. 2006). In addition to physical barrier properties, the skin also produces many antimicrobial peptides, such as β-defensins and cathelicidins. And, these antimicrobial peptides have been shown to be suppressed during stressor exposure through the actions of stressor-induced glucocorticoids and local production of corticotrophin releasing hormone (Aberg et al. 2007). Thus, stressor-induced alterations in the skin permeability barrier, as well as innate defenses, may explain why bacteria were found in the inguinal lymph nodes of stressed mice.
Exposure to psychological stressors can have similar effects in the GI tract, with stressor-induced changes in gut permeability being well defined (Soderholm and Perdue 2001). These effects have primarily been described in laboratory animals, since studying the impact of psychological stressors on GI permeability in humans has been challenging. Several studies have demonstrated that immobilizing rats in a cold environment significantly increased jejunal permeability to 51Cr-EDTA and mannitol (Saunders et al. 1994) via a cholinergic dependent mechanism (Saunders et al. 1997; Soderholm and Perdue 2001). In the colon, permeability was also increased by immobilization in a cold environment, an effect that could be mimicked by peripheral injection of CRH (Saunders et al. 2002; Soderholm and Perdue 2001).
Enhanced microflora growth and increased permeability of barrier defenses may not be sufficient to result in bacterial translocation. In fact, an additional important component of bacterial translocation is the ability of enteric bacteria to adhere to intestinal tissue. Interestingly, stressor-induced neuroendocrine hormones can also enhance the attachment of enteric bacteria. For example, culturing pathogenic E. coli O157:H7 with NE significantly increased the ability of the bacteria to adhere to colonic tissue (Chen et al. 2003; Green et al. 2004). Moreover, internalization of pathogenic (i.e., Salmonella choleraesuis and E. coli O157:H7), but not necessarily commensal, bacteria was enhanced by treating porcine Peyer’s patch mucosa with NE in an Ussing chamber paradigm (Green et al. 2003).
These studies demonstrate that exposure to psychological stressors affects many aspects of host physiology. And, many of these effects have the capacity to alter the commensal microflora. When considered together, a likely scenario emerges from the data in which exposure to a psychological stressor results in a neuroendocrine response that has the potential to directly or indirectly affect commensal microflora populations, the integrity of barrier defenses, and the internalization of microbes. Delineating whether the effects of stress on the microflora are direct effects, i.e., whether stress hormones themselves affect microflora in vivo, or indirect, i.e., through modulation of the microenvironment in which commensals interact with their host, will be a challenge for future studies. However, as animal models to study the interactions between the microflora and their host continue to be developed, insight into the impact of stress on these interactions will undoubtedly follow.
11.8 An Integrative Hypothesis of Stress, Infection, and Immunity
The importance of stressor-induced alterations in commensal microbial populations and translocation to regional lymph nodes is only beginning to be understood. Although the ability of microbial populations to limit pathogen colonization and invasion has been known for many years, it is now thought that these commensal microbes help to regulate the immune system as well. In GI tissue, for example, inflammation is low despite the enormous antigenic potential of the billions of colonized bacteria. These commensal bacteria, though, may actually be active players in maintaining homeostasis through the suppression of innate pattern recognition receptor signaling. For example, signaling through the Toll-like receptors (TLR), which results in cytokine production, is negatively regulated through Toll-interacting protein (Tollip) and single immunoglobulin IL-1R-related molecule (SIGGR) (O’Hara and Shanahan 2006). Importantly, Tollip expression is directly correlated with microflora levels; the highest levels of Tollip are found in healthy colonic tissue that also has the highest microflora levels (O’Hara and Shanahan 2006; Otte et al. 2004). Thus, as long as healthy levels of microflora are maintained within the GI lumen, inflammation may be actively suppressed.
If the stress response facilitates the passage of bacteria from the lumen into the intestinal tissues, however, the TLRs found on the basolateral surface of enterocytes would then be able to respond to translocating bacteria to initiate an inflammatory response (Abreu et al. 2001; Otte et al. 2004). Moreover, there is an immense network of phagocytes and antigen presenting cells residing below the enterocytes within the GI tissue that can respond to translocating microbes and cause a local or systemic inflammatory response. Thus, it is possible that innate receptors, such as the TLRs, are silenced in the presence of normal luminal levels of microflora, but activated when microflora levels are altered or when they translocate into the tissue.
The ability of stressors to induce and/or enhance the inflammatory response is now well recognized. For example, stressor-induced elevations in circulating inflammatory markers have been found in uninfected humans (Brydon et al. 2005, 2006; Steptoe et al. 2007; Coussons-Read et al. 2007; Bierhaus et al. 2003) as well as in uninfected rodents (Avitsur et al. 2001, 2002; Bailey et al. 2007; Engler et al. 2008; Stark et al. 2001). In rodents, it has been shown that exposure to certain stressors causes leukocytes, especially monocytes/macrophages, to become resistant to the suppressive effects of corticosterone (Avitsur et al. 2001; Bailey et al. 2004a; Engler et al. 2005; Stark et al. 2001). Moreover, these cells have a primed phenotype and show exaggerated inflammatory responses to ex vivo stimulation with LPS or even intact bacteria (Avitsur et al. 2003; Bailey et al. 2007), effects that were associated with the inability of glucocorticoids to suppress the activation of the transcription factor NF-κB (Quan et al. 2003). Human studies employing gene chip technology corroborate findings from murine studies, and have found an underrepresentation of genes containing a glucocorticoid response element and an overrepresentation of genes controlled by the transcription factor NF-κB (which controls the transcriptional expression of inflammatory cytokines) in uninfected individuals reporting high levels of psychological stress (Miller et al. 2008; Cole et al. 2007). These data suggest that periods of psychological stress in humans are associated with an inflammatory profile that is not able to be controlled by endogenous glucocorticoids.
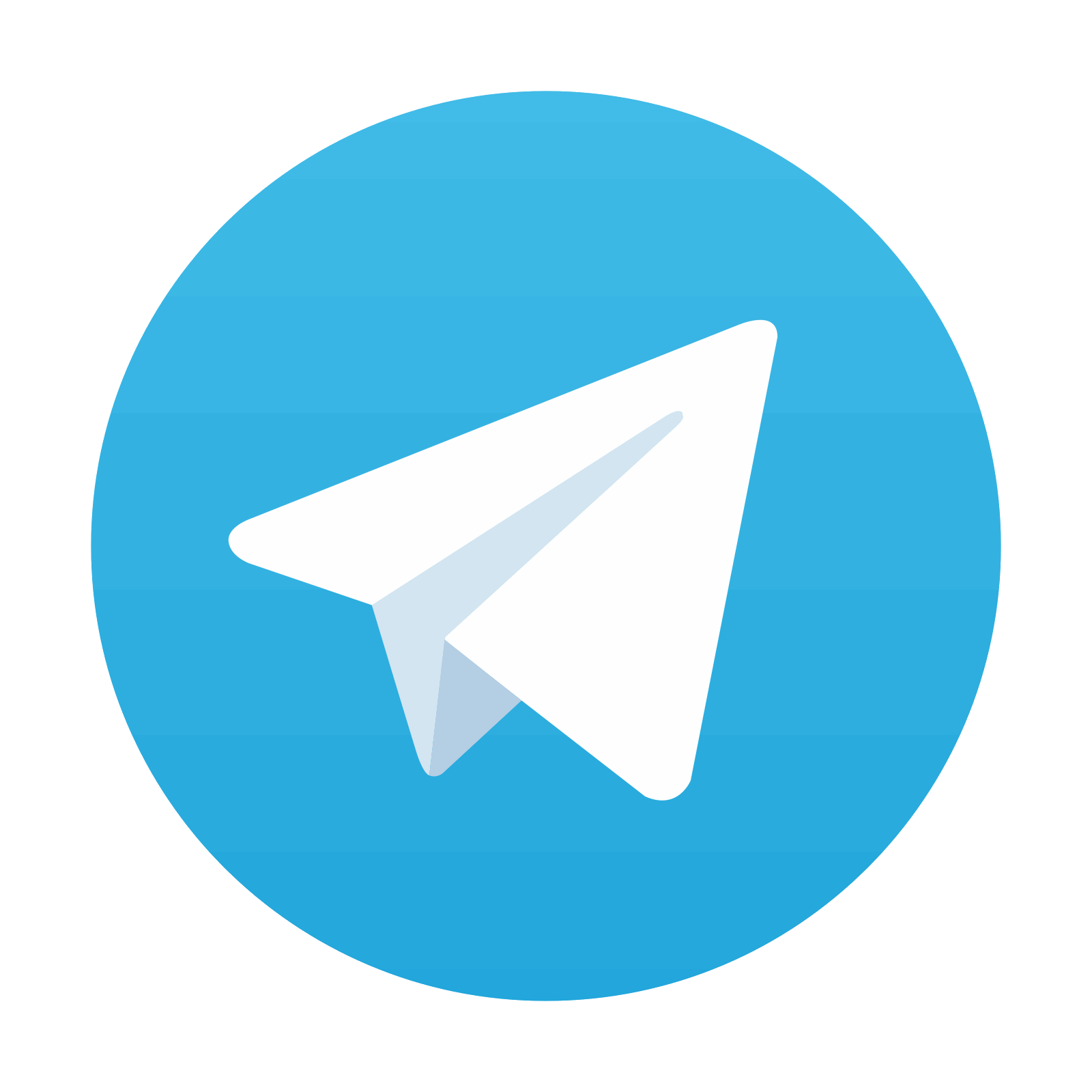
Stay updated, free articles. Join our Telegram channel

Full access? Get Clinical Tree
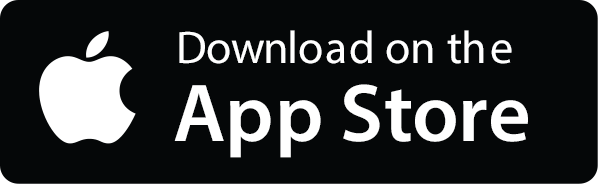
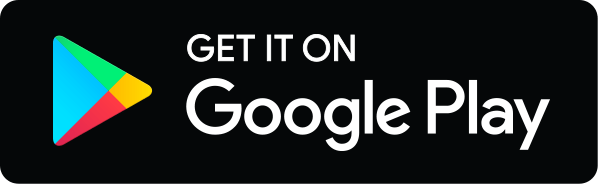