3
Microwave Ablation:Mechanism of Action and Devices
Microwave ablation (MWA) is a new treatment modality within the field of thermal ablation. Similar to radiofrequency ablation (RFA) and cryoablation, MWA may be performed under image guidance in the operating room during open or laparoscopic procedures, or percutaneously as an outpatient therapy. Compared with surgical resection, percutaneous image-guided ablation provides a minimally invasive treatment option ideal for nonoperative candidates. With the advent of these technologies comes a reduction in procedure-related morbidity and mortality, decreased cost due to the elimination of operating room time and staffing, significantly fewer hospital admissions, and faster recovery times. The synergistic effect of ablation therapy combined with conventional treatment options of chemotherapy and external beam radiation may provide improved long-term treatment success and survival.1–7 As a nascent treatment modality, MWA is clinically applicable to a broad spectrum of cellular pathology and malignancies that are susceptible to its cytotoxic effects.
Compared with other thermal technologies, including RFA and cryoablation, microwave energy offers unique advantages including an improved convection profile, the generation of consistently higher intratumoral temperatures, larger ablation volumes due to the synergistic effects of using multiple antennae simultaneously, and reduced treatment times. MWA has been most commonly utilized in the treatment of hepatic, pulmonary, and renal malignancies. The highly localized nature of this applied energy and percutaneous technique is ideal for patients with impaired hepatic, pulmonary, or renal function, as neoplastic tissue is ablated and remaining functional parenchyma is preserved.8–12 Technically, microwave is the modality of choice when treating lesions that are greater than 4 cm in size, are located in close proximity to a sizable vessel larger than 3 mm in diameter due to minimal “heat-sink” effects, or when treating a lesion that has recurred following prior thermal ablation.13
♦ The Physics of Microwave Ablation
Microwave energy is a form of electromagnetic radiation with frequencies ranging from 900 to 2450 MHz, and it lies on the spectrum between radio waves and infrared radiation. The waveform used to represent electromagnetic radiation demonstrates that this energy carries an electric charge that oscillates between positive and negative values, creating a rapidly alternating electric field. For instance, microwave energy oscillating at a frequency of 920 MHz changes signs approximately two billion times a second.14 Water molecules (H2O) within biologic tissues also carry electrical charge. The portion of the molecule containing two hydrogen molecules is positively charged, and the oxygen-containing portion is negatively charged (Fig. 3.1A). This asymmetric distribution of charge subsequently creates polar water molecules.
When water molecules are exposed to the oscillating electric charge from a microwave source, the molecules function as small electrical dipoles that flip back and forth in spatial orientation, rapidly spinning in an effort to align with electromagnetic charges of opposite polarity (Fig. 3.1B). Thus, microwave energy is able to cause water molecules to spin and change orientation 2 to 5 billion times a second.14 The microwave probes that are strategically placed within the ablation target in biologically active tissues function as antennae that transmit this high-frequency microwave energy. Given that temperature is a measure of molecular movement within matter or molecular kinetic energy, this rapid flipping and molecular agitation against frictional forces results in a significant rise in water temperature and heating of the target tissue surrounding the microwave applicator.
At temperatures up to approximately 40°C, cellular homeostasis is able to be maintained in living tissues. When temperatures of 42° to 45°C are reached, cells become increasingly vulnerable to damaging agents such as radiation and chemotherapy, but remain resistant to cell death despite prolonged exposures to these temperatures. However, if the temperature is increased slightly to 46°C and exposure time exceeds 60 minutes, irreversible cellular injury ensues, and at 50°C the exposure threshold is lowered to only 4 to 5 minutes.15,16 Temperature end points of 50° to 100°C have been shown to be optimal for the induction of protein and enzymatic degradation and the denaturation of histone complexes that are required to maintain the tertiary structure of DNA.15,17,18 Following exposure to these cytotoxic temperatures, in time, cellular death ensues by means of coagulation necrosis.14
Fig. 3.1 (A) Schematic illustration of a polar water molecule. (B) Schematic illustration of the interaction between an oscillating electromagnetic microwave waveform and water molecules.
Clinically, RFA creates a zone of active tissue heating emanating only a few millimeters from the electrode active tip, and the remainder of the ablation zone is heated passively via thermal conduction.19 In vivo, microwave antennae are able to generate a relatively larger zone of active tissue heating by creating a broad elliptical field of high-power density extending up to 2 cm in radius from the antennae itself. This improved convection profile of active heating allows for more uniform induction of cell death within the ablation zone or “zone of kill.”20 The electromagnetic nature of microwave-generated energy also eliminates the increase in impedance caused by RFA. Radiofrequency (RF)-induced tissue charring and boiling responsible for this increased impedance provide electrical insulation, thus limiting the spread of energy and the size of the RF-induced ablation zone.17,20 Free of these restricting properties, microwave energy creates significantly higher intratumoral temperatures, aiding in the creation of more sizable ablation zones in a shorter period of time.
♦ Ablation Systems
Equipment and Setup
At our institution, ablations are performed on a computed tomography (CT) scanner under fluoroscopic guidance. Microwave energy is delivered to a target lesion by means of an antenna that is electrically coupled to a microwave generator by means of a coaxial cable. Each antenna is individually powered by a single microwave generator capable of producing up to 45 W of power at a frequency of 915 MHz (Fig. 3.2). Antennae emit electromagnetic microwave energy from the noninsulated, 0.25-cm-wide gray stripe approximately 1.5 cm from the distal tip. Treatment parameters are determined in accordance with the manufacturer’s recommendations for the antenna being used, most commonly consisting of 10-minute treatment times at 45 W and 915 MHz.
Fig. 3.2 Microwave generator. (Evident™ Microwave Ablation System; Valley Laboratory/Covidien, Boulder, CO)
Microwave antennae, all 14.5-gauge with 3.7-cm active tips, have total shaft lengths of 12, 17, or 22 cm (Fig. 3.3). To minimize the risk of burn injury, antennae are equipped with sensors that monitor shaft temperature. By means of automatic feedback regulation, the generator will shut off if the proximal shaft in contact with the patient’s skin exceeds a temperature of 43°C. Additionally, a remote thermocouple sensor may be placed to accurately monitor the temperature within the tumor and developing ablation zone intraprocedurally to indicate treatment progress (Fig. 3.4).
Currently, in the United States, there is only one commercially available percutaneous microwave ablation system, the Evident™ Microwave Ablation System (Valley Laboratory/Covidien, Boulder, CO). Additional systems in development, designed to function at 915 to 2450 MHz, have not yet either obtained U.S. Food and Drug Administration clearance or become commercially available. The pMTA (percutaneous Microwave Tissue Ablation) System (Acculis; Denmead, Hampshire, UK), the Certus 140 percutaneous microwave device (NeuWave Medical, Madison, WI), and systems from MedWaves, Inc. (San Diego, CA) and BSD Medical (Salt Lake City, UT) are currently in development.
Fig. 3.3 Microwave antennae (Evident™ Microwave Ablation System; Valley Laboratory/Covidien, Boulder, CO). From left to right: 3.7-cm active tip intraoperative antenna with a noncooled shaft, and 14.5-gauge percutaneous antennae with shaft lengths of 22, 17, and 12 cm, each with a 3.7-cm active tip. All three antennae designed for percutaneous use have cooled shafts. Clear plastic tubing (arrow) with two lumina side by side runs in parallel to the coaxial power cable (arrowhead) and provides a continuous normal saline flush circuit (blue stripe indicates input, no stripe indicates output). All cables are encased within a three-dimensional zigzag array of white plastic to prevent the blue coaxial cable from coming in direct contact with the patient’s skin, thus minimizing the risk of burn injury.
Multiple microwave generators are contained within the Valley Laboratory system currently being used at our institution. Also included, is a specialized pump that provides the outer shaft of each antenna designed for percutaneous use with a continuous infusion of normal saline at 60 cc/min for internal cooling to prevent thermal injury to the tissues along the length of the antenna shaft. This coolant is contained within the antenna, never directly immersing the tissues being treated, and is delivered by means of clear tubing running parallel to the coaxial cable connecting the antenna to the microwave system (Fig. 3.3). Each microwave generator is equipped with one output channel, making it possible to power a single antenna.
Multiple antennae may be used per treatment as long as an equal number of generators are used simultaneously, in a 1:1 ratio. This flexibility in selecting antennae number and three-dimensional array for each lesion to be treated allows for the creation of substantially larger ablation zones, making it possible to ablate more sizable lesions. When using multiple antennae simultaneously, each should be placed 1.5 to 2.0 cm apart to fully take advantage of the ablation zone generated by each, and to minimize excessive heating due to overlapping. Additionally, unlike RFA systems, grounding pads are not required when using microwave technology.
Ablation Protocols
Preprocedural Workup and Patient Preparation
Prior to undergoing a thermal ablation procedure, patients are routinely seen in a standard outpatient consultation. At this time, all relevant imaging studies are reviewed, a detailed medical history is obtained, and a focused physical examination is performed. The most suitable thermal ablation modality is then selected based on tumor location, biology, and treatment goals. All risks, benefits, potential complications and side effects, and alternative treatment options are fully explained to the patient during this visit. Any additional preprocedural imaging studies needed to ensure sufficient planning of the percutaneous approach to the target lesion and any laboratory testing deemed necessary are ordered by the treating physician or nurse practitioner at this time. Coagulation profiles including prothrombin time, international normalized ratio, and partial thromboplastin time laboratory studies are routinely evaluated. Patients with internal cardiac defibrillators or pacemakers are advised to consult with the treating electrophysiologist pre-and postablation.21–23
All patients are to fast after midnight on the eve of their scheduled ablation procedure in an effort to minimize any risk of sedation-induced aspiration. Cardiac or antihypertensive medications should be taken as prescribed with a sip of water on the morning of the procedure. For patients with insulin-dependent diabetes, only half of their morning insulin dose should be administered in standard fashion to prevent hypoglycemia secondary to overnight fasting. Aspirin and antiplatelet agents should be held for the appropriate number of days prior to ablation, ranging from 2 to 7.
On the day of the planned ablation, patients arrive at the radiology suite preprocedural holding area. Here, a focused history is obtained, and physical examination is performed to assess all relevant systems prior to the procedure. Informed consent is obtained at this time, the treatment site and side are marked, and any residual questions and concerns are addressed. A peripheral intravenous line is then placed by the nursing staff for fluid and sedative administration during the perioperative period. Prophylactic antibiotics are not routinely administered.
Planning of Computed Tomography–Guided Approach and Microwave Ablation
The patient is transported to the CT suite and positioned on the gantry table in a supine, prone, or lateral decubitus position as determined by the anticipated location of the percutaneous access site. An initial baseline CT scan is obtained, confirming the size and location of the target index lesion. These preliminary images are analyzed on the workstation monitor with a superimposed software-generated mapping grid. This allows for on-screen measurements to be made that indicate the precise three-dimensional location of the lesion within that slice or image, and subsequently determine the percutaneous access site and antenna trajectory. Similarly, horizontally and vertically aligned laser lights are generated by the CT gantry, and correspond to the software-generated mapping grid previously visualized on the workstation monitor. A handheld ruler is then placed upon the patient and used to measure out the distances as determined on the mapping software, ensuring accurate access site position and antenna trajectory angulation.
Fig. 3.4 A 72-year-old man with bladder cancer metastatic to the right hip. (A,B) A circular hypodense osteolytic lesion is found within the anterior aspect of the right acetabulum on preablation axial CT images. Intraprocedurally, a 3.7-cm active tip microwave antenna was percutaneously placed (arrowhead in C) in addition to a remote thermocouple sensor (arrows in D,E) to accurately detect the temperature within the ablation zone. A single 10-minute treatment at 45 W was performed to a temperature of 60°C. (F,G) Fifteen-month postablation follow-up axial CT images show a robust periosteal reaction with sclerosis (asterisk) and no evidence of residual or recurrent disease in the ablation zone.
3 Microwave Ablation: Mechanism of Action and Devices 31
The skin overlying the access site is then marked, prepped, and draped in the standard sterile fashion. With a 25-gauge skin needle, 1% lidocaine buffered with bicarbonate is routinely injected at the access site intradermally, and then more deeply with a 22-gauge spinal needle to provide additional local anesthesia. A small nick is then made in the skin at the access site with a No. 11 scalpel blade. The spinal needle is reintroduced into the access site and CT-fluoroscopy is engaged Fig. 3.5A,B). The spinal needle is visualized, confirming table positioning and needle angle, as this will dictate the trajectory of the microwave antenna(e) soon to be inserted. This is an opportune time for patient repositioning or to make adjustments in the spinal needle trajectory. The spinal needle is then switched out for the microwave antenna of choice.
Fig. 3.5 (A) Sterile tray of percutaneous access supplies to the left of the patient, who is placed on the gantry table in a supine position.(B) The access site (arrowhead), localized by means of the horizontally and vertically aligned laser array (arrow), has been marked, prepped, and draped in standard sterile fashion and the spinal needle is in place (arrowhead). The selected microwave antenna (C) is then percutaneously placed under CT-fluoroscopic guidance (D).
When selecting the appropriate antenna, it is important to understand that the desired outcome of percutaneous microwave ablation is twofold in nature: (1) to achieve complete eradication of all viable malignant cells within a designated area, and (2) to minimize destruction of surrounding normal tissue within the treated region. In compliance with resection principles of surgical oncology, malignant lesions require a 1.0-cm disease-free margin. Thus, when ablating malignant neoplasms for the purpose of curing disease, a 1.0-cm margin of normal cell death around the target lesion is desired.
This has been shown to significantly reduce the presence of residual disease and risk of subsequent recurrence due to ineffective destruction of malignant cells at the periphery of the lesion.18,24 However, when treating benign lesions or when striving to provide only palliation, sufficient ablative margins are not required; rather, damage to surrounding structures and adjacent tissues should be minimized.
In addition to the treatment goal, there remain several criteria to be considered when selecting an antenna. Index tumor size, distance to the target lesion from the percutaneous access site, and proximity of nearby vital structures or great vessels are all relevant factors that allow microwave antenna(e) active tip, overall length, gauge, and multiplicity to be determined. One to six microwave antennae may be used simultaneously, allowing for the treatment of larger lesions. A 4.5- or 6.0-cm zone of kill emanating in an elliptical array centered approximately 1.5 cm from the distal tip of the antenna may be created during a single 7- to 10-minute treatment depending on the active tip length of 1.6 or 3.7 cm.
The chosen microwave antenna is positioned within the tract previously made by the spinal needle, and then advanced along the trajectory approximately halfway to the target lesion. An image is then obtained under CT-fluoroscopy providing an opportunity for the trajectory of the antenna to be adjusted in any of three dimensions (x, y, or z plane) before further advancement. This process of obtaining and reviewing images and advancing the antenna along a desired trajectory is continued until the antenna is positioned as desired within the target lesion (Fig. 3.5C,D). Technically, the “sweet spot” or hottest point on the antenna shaft, indicated by a ¼-cm wide gray stripe approximately 1.5 cm from the distal tip, should be centered within the lesion to ensure even and adequate coverage of the target. This stripe is the point at which microwave energy is generated (Fig. 3.6), and placing it in the center of the target lesion ensures optimum ablation geometry.
Fig. 3.6 (A) Axial CT-fluoroscopic image showing the “sweet spot” (arrow) of the percutaneously placed microwave antenna approximately 1.5 cm from its distal tip. Microwave energy, in the form of electromagnetic waveforms, is generated at this precise point (B), from which it emanates into surrounding tissues in an elliptical shape (C).
Additional antennae may be percutaneously placed in the manner described above. Prior to turning on the generator, midazolam (0.5–1 mg/dose) and fentanyl (25–50 μg/dose) are administered intravenously to provide moderate sedation. Vital signs and electrocardiogram tracings are continuously monitored and charted by a dedicated nursing staff member to ensure that a sufficient but safe degree of moderate sedation is maintained. Lesion size and location as well as patient positioning and pain tolerance greatly influence the amount of sedation required, and general anesthesia is rarely necessary. Depending on the chosen antenna(e) and generators used, each ablation should be performed according to the manufacturer’s specifications for wattage and treatment duration. Commonly used treatment parameters for a 3.7-cm active tip microwave antenna are a power of 45 W and a duration of 10 minutes, per treatment. The number of treatments per ablation session is determined intraprocedurally.
Index lesions greater than 2.0 cm in maximum diameter require the use of multiple microwave antennae simultaneously or consecutive single antenna treatments to ensure creation of a sufficiently sized thermocoagulation zone. Completeness of ablation is determined by the treating physician, who is actively viewing the CT-fluoroscopic images and tracking immediate postablation imaging changes within the ablation zone. When treating pulmonary lesions, commonly observed findings include ground-glass opacification, gas bubbles, and a surrounding hypodense halo-like rim. Upon completion, each microwave antenna is withdrawn, the access site is cleaned with first a damp and then a dry cloth, and a dry sterile dressing is placed on the skin.
Postprocedural Recovery
Upon completion of the procedure, the patient is transported to the postprocedure recovery room within the radiology suite. Here the patient remains for 1 to 3 hours while the effects of sedation, moderate or general, resolve. Vital signs are recorded as the patient is observed and monitored by the nursing staff. When fully awake, the patient is allowed to gradually resume oral intake as tolerated. The treating physician is notified if there are any signs of early complications. As the patient was given sedating medications, driving a motor vehicle is prohibited postprocedurally, and thus the patient may be discharged home only under the care of a friend or relative who has agreed to provide transportation.
At this time, discharge instructions are explained to the patient both verbally and in writing. It is recommended that acetaminophen or acetaminophen/hydrocodone be used for the initial treatment of postprocedural discomfort, and that the use of aspirin and nonsteroidal antiinflammatory drugs be resumed several days later due to an increased risk of bleeding in the immediate postprocedure period. Discharge documentation includes the department and on-call radiologist’s phone numbers, and patients are instructed to call if they are experiencing increasing pain, redness, swelling, or bleeding at the ablation site, fever greater than 101.5°F, or increasing chest pain or shortness of breath. A follow-up outpatient visit at the ablation clinic is routinely scheduled within 1 week. At this visit, plans for follow-up care and imaging are arranged at anticipated 3-, 6-, and 12-month intervals, or as clinically indicated.
♦ Clinical Practice
There are numerous well-documented advantages to the use of MWA that have become evident as clinical research studies come to define its niche within the field of percutaneous thermal ablation. When compared with RFA, MWA has been shown to provide a minimally invasive treatment option that offers an improved convection profile, enabling the generation of consistently higher intratumoral temperatures. With a decreased vulnerability to the heat-sink effect demonstrated by RFA, sizable vessels in close proximity to target lesions are not contraindications to MWA. With the unique flexibility of using multiple applicators simultaneously, synergistic energy deposition results in the creation of larger ablation zones, making microwave the modality of choice for treating larger lesions. Total MWA treatment times are also shown to be significantly shorter compared with RFA, as the required number of applications are fewer, and each ablation faster. Lastly, when using microwave technology, it is not necessary to place grounding pads on the patient.
Microwave Ablation of Hepatic Lesions
Hepatocellular carcinoma (HCC), a primary malignancy of hepatic origin, is one of the most prevalent and most lethal cancers, causing 662,000 deaths worldwide each year.25 Most cases are secondary to alcoholic cirrhosis or chronic infection with hepatitis B and C in endemic regions.26 In the United States, HCC incidence continues to escalate, correlating with the observed rise in chronic hepatitis C–induced cirrhosis.27Prognostic outcomes remain poor, as only 10 to 20% of HCCs are able to be surgically resected at the time of presentation due to the extent of disease or poor liver function.26 Resection has been shown to increase both 5-year survival rates among these patients, as well as disease-free survival.28–30 Without successful surgical intervention and removal, average life expectancy is only 3 to 6 months.25 As such a small number of patients are surgical candidates, minimally invasive thermal ablation has emerged as a treatment option increasing in popularity.
Despite its worldwide popularity, there are disadvantages to the use of RFA for the treatment of HCC. There remains a high rate of local recurrence, especially when ablating lesions greater than 3.0 cm in diameter, a significant heat-sink effect due to local blood flow when the lesions are in close proximity to blood vessels, and pathologic evidence of surviving tumor cells within the treated lesion.31,32 Thus, microwave ablation has been proven by several groups of investigators to be a superior thermal modality in this setting.33–36
In the United States, colorectal carcinoma, superseded only by lung cancer, is the second most lethal cancer in both men and women, accounting for 9% of all cancer-related deaths.37Mortality rates are approximately 50,000 per year, with 49,960 deaths in 2008, of which the majority were attributable to metastatic disease.38 Untreated hepatic metastases carry a particularly poor prognosis, with a 5-year survival of less than 2% and a median survival of less than 12 months.39Despite the popularity of RFA, high local recurrence rates remain a concern when ablating hepatic colorectal metastases greater than 3.0 cm in diameter.40–43 Additionally, procedural times for RFA become lengthy as multiple sequential overlapping applications are necessary to ensure sufficient coverage of larger lesions.40,41 Thus, microwave ablation emerges as the minimally invasive thermoablative technique of choice in this clinical setting as well.
The ablate and resect study by Simon et al44 was a phase 1, two-center clinical trial of 10 patients with hepatic masses, either HCC or colorectal carcinoma metastases, who underwent synchronous triple antenna MWA prior to elective hepatic resection. Intraoperatively, three straight microwave antennae (13-gauge diameter, 15-cm length, 3.6-cm active tip) were arranged in a triangular configuration spaced 1.5 cm (n = 3), 2.0 cm (n = 6), or 2.5 cm (n = 1) apart with a manufacturer-produced rigid spacer. Each of the three antennae was powered simultaneously such that each lesion was synchronously ablated by three antennae in a single treatment at 45 W for 10 minutes under real-time ultrasonography. The mean tumor diameter was 4.4 cm, with a resultant mean maximum ablation diameter of 5.5 cm and average ablation zone volume of 50.8 cubic centimeters.44
On pathologic examination, ablation zones were found to contain a pale central area of coagulation necrosis encompassed by a red hyperemic zone. Both were devoid of viable cells, creating a sharp border demarcating ablated from viable regions. Thus, sizable ablation zones with uniform cellular death were created in only 10 minutes, compared with RFA sessions requiring 12 to 25 minutes per application. Also included in these resected specimens were blood vessels greater than 3 mm in diameter.44 Due to the minimal heat-sink effect observed with MWA, there was complete cellular death surrounding these vessels, with the typical ablation zone distortion observed with RF energy not seen. RFA may remain the appropriate modality for small lesions ≤3 cm, with MWA most suitable and efficient in the percutaneous treatment of lesions >3 cm and in close proximity to sizable vessels (Fig. 3.7).44–46
Fig. 3.7 A 63-year-old man with colorectal carcinoma, status post–left colectomy 3 years prior, presents for MWA of hepatic metastases. (A) Preablation positron emission tomogram shows two fluorodeoxyglucoseavid hepatic lesions corresponding to the heterogeneously enhancing foci seen on CT. (B) A contrast-enhanced axial CT image depicts two lesions in the right hepatic lobe: 3.8-cm lesion in segment VII (white arrow) and a 2.6-cm lesion in segment VIII (black arrow). At the discretion of the treating surgeon, MWA was chosen over surgical resection, as the lesion abutting the middle hepatic vein would have necessitated trisegmentectomy. (C) One month after intraoperative MWA of both lesions (three antennae with 3.7-cm active tips at 45 W for 10 minutes—one treatment for segment VII lesion, two treatments for segment VIII lesion), contrast-enhanced portal-venous phase CT shows corresponding postablation cavities (white and black arrows) without any areas of enhancement to suggest the presence of residual or recurrent disease. Note that the zones of induced thermal scarring are larger than the treated index lesions. Also, there is a sharp margin demarcating the ablation cavity in segment VIII (tip of black arrow) as the incurred heat-sink effect was minimal and the ablation zone abuts the middle and right hepatic veins. (D,E) The 27-month postablation follow-up PET-CT reveals contraction of the thermal scar with no evidence of FDG-avid disease, as both axial (D) and coronal (E) images show a photopenic defect (white arrows) corresponding to the sites of prior ablation.
Also highlighting the minimal heat-sink effect observed with MWA (Fig. 3.8) is the study by Wright et al47 that makes direct comparisons between microwave and RFA in a hepatic porcine model. Data show that ablation zones induced by both RF and microwave energy become larger and more uniform secondary to occlusion of hepatic vascular inflow.48–51 However, the heat-sink effect caused a 3.5 ± 5.3% deviation of the ablation zone in microwave ablated tissue compared with 26.2 ± 27.9% in RF ablated tissue (p <.05).47 In biologically active tissues, RF electrodes create only a small zone of active heating on the scale of several millimeters induced by ionic agitation. The majority of tissue heating is passive, secondary to thermal conduction, and is known to decrease exponentially with increasing distance from the electrode source. Finite-element computer modeling theorizes that consequently there is an inefficient conversion of electrical energy into heat, most notably at tissue–vessel interfaces where perivascular tumor cells and tissue are thermally protected by the dissipatory effects of flowing blood.52
Conversely, the area of active heating induced by microwave technology is a function of the wavelength of the applied energy resulting in a comparatively larger zone of active heating that is less susceptible to this vessel-mediated cooling effect. Histologically, the liver is so well vascularized that any sizable lesion will be in close proximity to at least one large blood vessel, making this heat-sink effect a contributory factor in the higher rates of local recurrence reported in clinical RF liver ablation studies.53 The thermal protection of malignant cells provided by this perfusion-mediated cooling (heat-sink effect) is partly responsible for the greater recurrence rates seen after RFA compared with MWA, with the former ranging from 2.4 to 39%.53–57
Phase two of the aforementioned hepatic ablation trial was performed by Iannitti et al,58 who studied 87 patients with primary and metastatic lesions averaging 3.6 cm in diameter. Single-antenna or clustered arrays were used in these ablations at 45 W for 10 minutes, with procedures performed open, laparoscopically, or percutaneously under ultrasound or CT guidance, with proportions of 45%, 7%, and 45%, respectively. Single-antenna ablations were found to result in ablation volumes of 10 mL, and clustered arrays in volumes of 50.5 mL. Lesions >3.0 cm in diameter were also successfully treated at our institution as shown by a retrospective review examining the safety and efficacy of MWA of hepatic malignancies in 70 patients.59 This study by Wolf et al59 details the treatment of both primary and metastatic lesions ranging in size from 0.3 to 12.0 cm (mean 4.2 ± 2.0 cm) in maximum diameter. Lesions were ablated intraoperatively and percutaneously under ultrasound and CT guidance, respectively, with a technical success rate of 96%.59
Iannitti et al58 demonstrated that clustered arrays of antennae create significantly larger ablation volumes. Similarly, up to four straight microwave antennae, with lengths of 12, 17, or 22 cm and active tips of 1.6 or 3.7 cm were used in lesion-specific customized three-dimensional arrays at our institution.59 In the initial 6 months following ablation, 12% of treated lesions (14/118) were found to have residual disease at the ablation site, not predicted by index tumor size. This illustrates that residual disease (remaining viable tumor cells within the ablation zone) was not due to inadequate induction of coagulation necrosis within and surrounding the index tumor. In contrast to the usual 3.0 cm cutoff, the lesion size for which RFA becomes inefficient, Wolf et al59 showed that index size did not become significantly predictive of outcomes until lesions reached sizes >4.5 cm. For example, index tumor size >4.5 cm was shown to predict recurrent disease at the ablation site (p = .02) with a mean 13 ± 1 months to first recurrence, as well as cancer-specific mortality.59
The practice of using multiple microwave antennae simultaneously in three-dimensional arrays to create more sizable ablation zones, allowing for the treatment of larger lesions, has emerged from much clinical experimental evidence (Fig. 3.9). The efficacy of triangular and spherical arrays was investigated by Yu et al60 in nine patients who underwent intraoperative, ultrasound-guided MWA of HCCs ranging from 2.9 to 6.0 cm in diameter (mean 4.2 cm) prior to surgical resection. A single-straight (13-gauge, 15 cm, 3.6-cm active tip) antenna was used in two patients, a triangular triple-straight (rigid spacer, separated by 1.5 cm, in one patient, and by 2.0 cm in three patients) antennae configuration in four, and a spherical triple-loop “cage” antennae configuration in three patients, determined based on lesion of size, location, and accessibility. The triple-loop antenna was composed of three 13-gauge shafts each with a deployable circular, wirelike tip or “loop.” Once positioned in the target tissue and deployed, a three-dimensional spherical cage took shape, designed to ablate the centrally contained tissue and a 3-cm external margin immediately adjacent to the loops.
The theoretical advantages of using a symmetric spherical cage to encircle the target lesion and ablate it “from the outside in” are proposed in a porcine study by Shock et al.61In nine hepatic porcine ablations, dual single-loop microwave antennae were used in an orthogonal configuration, and created significantly higher intratumoral temperatures (97.2°C) in the shortest amount of time (93.3 seconds) when compared with single and dual single-loop parallel antenna arrays. Higher temperatures were reached in a shorter period of time due to heating the tumor “from the outside in.” By first depositing energy peripherally, the blood supply to the target lesion is immediately eliminated, thus minimizing any heat-sink effect caused by ablation zone vascularity for the duration of the ablation. The dual single-loop orthogonal array also created ablation zones that were comparatively more spherical in shape, with no viable tissue found within the zone bounded by the loops.61
Clinically, the benefits of this caged-in theory are demonstrated in the work by Yu et al.60 Postablation coagulation volumes of 16.7, 51.7, and 54.3 cubic centimeters were reported for the single-straight, triangular triple-straight, and triple-loop antenna arrays, respectively. The triple-loop cage configuration also yielded the most uniformly round resultant ablation zone. Tissue heating progressing from the outer circumference inward created uniform coagulation of all enclosed tissue and a 0.5- to 1.0-cm rim immediately external to the cage. Additionally, when placing loop antennae, the tissues adjacent to the malignant lesion are targeted so that the lesion itself is not entered or punctured, eliminating the risk of tract seeding.61 Thus, arrays of loop antennae and three-dimensional straight antennae, with each component powered simultaneously, allow for more efficient and effective treatment of larger lesions when coupled with microwave technology. However, it remains unfortunate that the loop antennae used in these studies are not presently available for clinical use.
Fig. 3.8 A 65-year-old woman with a remote history of breast cancer and metastatic colorectal carcinoma status postcolectomy underwent intraoperative MWA of lesions in hepatic segments IVa and VII. The IVa lesion was ablated with a 3.7-cm active tip antenna at 45 W for 10 minutes. (A,B) One-month postablation follow-up CT portal venous phase axial images show a 1.4-cm hypoenhancing lesion in segment IVa (arrow) abutting the left hepatic vein and prior ablation zone (arrowhead), consistent with recurrent disease. (C–E) PET-CT images show intense FDG uptake within segment IVa. During planned surgical resection, intraoperative examination revealed a complex, irregularly shaped tumor encasing the left and middle hepatic veins, and adherent to the left border of the right hepatic vein, making the mass unresectable. Intraoperative MWA was performed using three antennae with 3.7-cm active tips at 45 W for 10 minutes, and then two antennae at 45 W for an additional 10 minutes. (F,G) One month after reablation, portal venous phase CT images show ablation defects in both segments VII and IVa with no evidence of residual or recurrent disease. As minimal heat-sink effect was incurred, the ablation zone in IVa extends up to the left hepatic vein (asterisk).
Fig. 3.9 An 88-year-old man with T3N0M0 non–small cell lung cancer presents with a 6-cm lesion in the left lower lobe abutting the medial visceral pleura and aorta. Under CT-fluoroscopic guidance four microwave antennae with 3.7-cm active tips were percutaneously placed in a diamond-shaped configuration: one superiorly (A), two in the middle (B),and one inferiorly (C), depicted by intraprocedural three-dimensional volume rendering (D). A single 10-minute treatment was performed at 45 W. The 1-month postablation contrast-enhanced axial (E,F) and coronal (G,H) CT images show scarring with associated pleural thickening and a walled-off ablation zone measuring 6.2 × 4.9 cm without any evidence of residual or recurrent disease.
In all clinical MWA studies performed at our institution, as well as in the work by Yu et al, microwave energy was delivered at a frequency of 915 MHz generated by the Evident™ Microwave Ablation System (Valley Laboratory/Covidien) and VivaWave Microwave Coagulation System (Vivant Medical), respectively.44,59,60 Research shows that these systems are tuned to the dielectric properties of hepatic tissue, promoting optimal absorption and distribution of energy.62 Treatment parameters routinely included the delivery of energy at a power of 40 to 60 W for 5 to 10 minutes.59,60 The results and implications of the work at our institution and in the aforementioned studies44,47,58–61 are contrary to the data recently reported by Ohmoto et al.63 In one study from Japan, 34 patients underwent RFA and 49 patients underwent MWA for HCCs each ≤2 cm in diameter in an effort to compare the two thermal modalities. Larger areas of postablation necrosis, decreased local recurrence rates, increased survival rates, and fewer complications were observed in the RFA cohort.63
A possible explanation of this discrepancy lies in the different MWA systems used and the applied treatment parameters. As mentioned previously, MW energy at a frequency of 915 MHz is thought to better match the electrochemical nature of biologic tissues, allowing for maximum penetration with minimal applied power. Of note, the Microtaze system used by Ohmoto et al63 delivered microwave energy at a frequency of 2450 MHz, and lesions were treated at 60 W for an average of 2.5 minutes. Likely, this higher frequency necessitated the application of additional power to generate sufficient tissue penetration. Thus, the effectiveness of MWA was limited in this context, causing less favorable outcomes and higher complication rates.
The safety of percutaneous image-guided MWA in patients with malignant hepatic lesions was examined in depth by Liang et al.64 Recent data from a cohort of 1136 patients with 1928 lesions collectively, who underwent 3697 MWA sessions, outlines the most commonly encountered complications. Treatment parameters were such that microwave energy was delivered at a frequency of 2450 MHz initially, and then at 915 MHz in subsequent treatments. Liang et al report that two deaths occurred in this study population, neither of which was directly attributable to MWA. This is congruent with the 0% mortality rates reported by both Iannitti et al58 and Wolf et al.59 Major complications occurred in 2.6% of patients (n = 30) and included the following: liver abscess and empyema formation (n = 5), bile duct injury (n = 2), colonic perforation (n = 2), tumor seeding (n = 5), pleural effusion requiring thoracentesis (n = 12), hemorrhage necessitating arterial embolization (n = 1), and skin burn requiring resection (n = 3).64
The most commonly encountered additional minor complications included the following: fever, pain, asymptomatic pleural effusion, gallbladder wall thickening causing minimal right upper quadrant abdominal pain, and skin burn not requiring therapeutic intervention.64 The most common postprocedural complaint was that of fever, ranging from 37.2° to 39.7°C, occurring within 24 to 48 hours following ablation and lasting 1 to 5 days. Localized pain at the site of percutaneous entry and along the antenna tract was also common, and reported in 80% of patients, with the majority, 66.5%, graded as 1 to 2 by National Cancer Institute common terminology criteria for adverse events (CTCAE) and lasting 1 to 3 days.64
The work of Wolf et al59 presents an intraprocedural minor complication of bradycardia requiring treatment with atropine (CTCAE grade 2) in two of 84 ablations, as well as postprocedural pneumothoraces in two ablations (one of which required chest tube placement; CTCAE grade 2). No major complications were reported by Wolf et al,59 Martin et al,65 and Iannitti et al.58 There was no correlation detected between increasing tumor size and complication rate. Rather, an increase in the number of MWA treatments per session and the use of noncooled antennae shafts were associated with a higher rate of major complications (p <.05).64,66 All percutaneous and laparoscopic ablations performed at our institution utilized cooled antennae, with the outer shafts continuously perfused with normal saline at a rate of 60 cc/ min to minimize thermal injury to superficial tissues and skin, preventing the known risk of increased complications resulting from the use of noncooled shafts.59
Microwave Ablation of Pulmonary Lesions
In the United States, lung cancer is the second most common malignancy and the most lethal, resulting in approximately 160,000 mortalities per year.67 Surgical resection remains the gold standard for the treatment of localized non–small cell lung cancer (NSCLC), offering the greatest promise of local control and survival. However, more than 15% of all patients who are diagnosed with stage I or II NSCLC have tumors deemed surgically unresectable, increasing to 30% for those who are 75 years of age or older.68 This may be due to tumor staging or medical comorbidities resulting in poor cardiopulmonary function and medically inoperability. Pulmonary tissue also remains a common site for metastatic lesions from various distant primaries throughout the body. Data show that some patients with oligonodular metastatic disease to the lungs may have increased survival with local treatment, such as surgical resection.69 However, cardiorespiratory comorbidities are also present within this population, creating a niche for percutaneous thermal ablation of pulmonary malignancies, both metastatic and primary, in nonsurgical candidates.
A recent study by Brace et al70 compares the effectiveness of both RFA and MWA in a normal porcine lung model. When RF current is applied to aerated lung parenchyma, the resultant impedance is shown to be several times greater than that encountered in both normal liver tissue and solid pulmonary tumors.71–73 At higher impedance limits, the amount of energy that may be locally deposited decreases, resulting in lower temperature rise in the targeted tissue and potentially increasing the risk of treatment failure.74 This limitation of RF energy is exacerbated by the aforementioned obstacles of insulation due to tissue charring and heat sink due to vessels greater than 3 mm in diameter that limit passive heat conduction.
Conversely, the penetrating ability of microwave energy and active heating is not limited by the lower electrical permittivity and conductivity of aerated lung and charred or desiccated tissues.71 The clinical significance of these electrophysical limitations lies in the following concept. When ablating lung tissue, the difficulty in achieving local control is due to the challenge of penetrating deeply enough into the normal aerated lung encasing the tumor in a way that a sufficient and predictable margin of normal lung parenchyma is destroyed, thus decreasing the chance that microscopic invasion or residual satellite nodules remain Fig. 3.10).75 In this swine lung model, microwave ablation (compared with RFA) resulted in larger and significantly more uniformly circular zones of thermal necrosis as well as faster growth of the ablation zone.70 A reduced amount of measurement variability also implied that MW energy may be consistently more effective.
Fig. 3.10 Gross pathology specimen showing viable, dark red, unablated lung parenchyma (zone I), an intermediate transition zone (zone II) of ablated but viable noncancerous lung tissue, and pale nonviable ablated tissue (zone III).
When compared with RFA in porcine lung with an equivalent sized applicator, microwave energy created ablation zones that were statistically 25% larger in mean diameter, 50% larger in cross-sectional area, and 133% larger in volume.70The ability of microwave technology to permit continuous heating at maximal power levels may be most advantageous in this setting. This is in contrast to the on/off pulsations of RF-delivered energy triggered when impedance thresholds are exceeded, allowing the tissue to rehydrate and cool, so that treatment may be completed.76 These results support the percutaneous use of MWA for the treatment of lung neoplasms, and its increased effectiveness compared with that of RFA.
Our group used MWA under CT guidance to treat 82 intraparenchymal pulmonary lesions, both NSCLCs and metastatic lesions, in 50 patients.77 These patients (mean age of 70) underwent a total of 66 MWA sessions, with multiple 7- to 10-minute treatments each performed at 40 to 60 W. The mean diameter of index lesions was 3.5 ± 1.6 cm, double that of tumors previously treated with RFA (all lesions <4 cm, mean diameter <1.7 ± 1.0 cm).78 Results showed that 1-, 2-, and 3-year cancer-specific mortality rates were not significantly affected by index tumor size >3 cm or ≤3 cm.77 This proves contrary to prior results obtained at our institution detailing the outcomes and survival in 153 patients with 189 primary or metastatic inoperable lung cancers who underwent percutaneous CT-guided RFA.79 This RFA study by Simon et al79 demonstrates a statistically significant improved survival rate in patients with index tumors 33 cm in diameter, compared with those patients with larger tumors >3 cm (p <.002). This comparison suggests that MWA is efficacious and should be the modality of choice when treating larger lesions.
Wolf et al77 report a 0% overall 30-day postablation mortality rate. The most common minor complication was pneumothorax, occurring after 39% of ablation sessions (69% of which did not require chest tube placement; CTCAE grade 1). A total of two skin burns were reported in these 50 patients, one of which required surgical intervention. Postablation syndrome, a constellation of productive cough with or without associated hemoptysis, residual postprocedural discomfort, and fever occurring in the few days immediately following ablation, was only shown to occur in one patient.
On initial postablation imaging, a CT scan obtained 1 to 30 days after ablation, the following imaging features were evaluated with intent to be followed on future scans: postablation diameter (ablation zone), cavitation, pleural thickening and retraction, pleural effusion, adenopathy (short axis, >1 cm), and lesion enhancement. Interestingly, of these features seen on follow-up imaging, the presence of cavitation at the ablation site proved to be significantly associated with a reduced cancer-specific mortality rate in patients who underwent pulmonary MWA (p = .02).77
Microwave Ablation of Renal Lesions
The incidence of renal cell carcinoma (RCC), a primary malignancy of the kidney arising from the lining of the proximal convoluted tubule, has been steadily increasing, with approximately 51,190 new cases diagnosed in the United States in 2007.37 Radical nephrectomy, once considered the only curative option for localized RCC, has recently been replaced by more conservative surgical options. Select patients may now be deemed suitable for nephron-sparing surgical resection.80,81 Despite being less invasive, partial nephrectomy remains a complex surgical procedure, with reported complication rates up to 30.1%.82
Percutaneous thermal ablation techniques have thus emerged as minimally invasive treatment options for patients with early-stage RCC, including those deemed nonsurgical candidates due to medical comorbidities. Studies have demonstrated efficacious tumor control with significantly lower complication rates subsequent to image-guided RFA and cryoablation of RCC.83–87 With resultant short- and intermediate-term results on a par with those seen following surgical intervention, RFA and cryoablation are increasingly used in the treatment of small RCC lesions.83–85 The practice of using MWA, a thermal ablation modality offering long-term survival rates comparable to surgical resection for HCC, remains relatively unexploited in the treatment of RCC.88,89
Fig. 3.11 An 82-year-old man with a history of chronic lymphocytic leukemia, Rai stage I and Binet stage A. (A) He presents with a complex exophytic mass at the lower pole of the left kidney measuring 4.0 × 4.5 × 4.8 cm on CT. As a poor surgical candidate, the patient was scheduled to undergo percutaneous biopsy and MWA of this solid renal mass. (B) Under CT-fluoroscopic guidance, a total of three microwave antennae, each with 3.7-cm active tips, were sequentially placed 2.0 cm apart within the epicenter of the lesion to ensure adequate coverage. A single 10-minute treatment of this biopsy-proven renal cell carcinoma was performed at 45 W. Three-year postablation follow-up axial (C) and coronal (D) CT images demonstrate stable, nonenhancing postablation changes at the lower pole of the left kidney, with no evidence of residual or recurrent disease. The ablation zone measures 5.7 × 5.1 cm and is encased by a hypodense rim of fat necrosis due to radial extension of the induced thermal gradient, and a circumferential rim of scar tissue.
In China, 12 patients with localized, clear cell RCC, underwent MWA of peripherally located single lesions ≤4 cm in diameter, with sizes ranging from 1.3 to 3.8 cm (mean 2.5 cm).90 Lesions were exophytic or intraparenchymal in growth pattern, with no invasion of the renal pelvis/calyx or adjacent structures such as the bowel or ureter. Liang et al90used a centrally positioned single antenna to ablate lesions ≤2 cm and two antennae (no more than 1.8 cm apart) for the ablation of lesions >2 cm. No major intra- or postprocedural complications were reported, and associated minor complications included pain not requiring pharmacologic intervention and self-limited asymptomatic microscopic hematuria. Although not reported in this series, RFA and MWA may result in thermally induced ureteral stricture. During a median follow-up period of 11 months, Liang et al found no evidence of recurrence on follow-up imaging, and ablation zones remained well defined on contrast-enhanced CT, suggesting that MWA may be an effective therapeutic option in the treatment of small, localized RCC lesions.90
This patient population may benefit from the numerous well-documented advantages of MWA, such as shorter procedural times and flexibility in using multiple applicators simultaneously (Fig. 3.11). For example, in a phase I study by Clark et al,91 10 patients with RCC lesions ranging in size from 3.9 to 13 cm were ablated with microwave energy prior to surgical resection. Results demonstrated that reproducible and sizable ablation zones can be created in single 10-minute treatments. Comparatively, to achieve similar effects with RF, multiple sequential treatments would be required.91 This would result in significant lengthening of the ablation session. Additionally, with a triple antenna array, Clark et al were able to produce a relatively large mean ablation zone measuring 5.7 × 4.7 × 3.8 cm. Within this zone, there was uniform tissue necrosis and no evidence of viable remaining renal neoplasm.
Fig. 3.12 A 78-year-old man with a spiculated right upper lobe lung mass presents for biopsy with on-site pathology and percutaneous MWA. (A) Preablation contrast-enhanced CT lung windowed image shows a 0.9-cm spiculated mass in the right upper lobe consistent with (biopsy-proven) small cell carcinoma. (B) With the patient in a prone position, a single 1.6-cm active tip microwave antenna was percutaneously placed under CT-fluoroscopic guidance, and two consecutive treatments for 10 minutes and then 7 minutes, each at 60 W, were performed. (C) One-year postablation follow-up contrast-enhanced CT shows thermal scar formation with associated pleural thickening and ground-glass opacification at the site of prior ablation. (D) Two-year postablation PET shows no evidence of FDG-avid disease within or adjacent to the RUL ablation site. Activity in this zone is less intense than the mediastinal background, a finding consistent with resolving postablation changes.
There are several disadvantages to the use of MWA in clinical practice. To date, there remains a need to place percutaneously a remote thermocouple device once all antennae have been properly inserted, just prior to turning on the generators. This device allows for the detection and monitoring of intratumoral temperatures throughout the ablation treatment, ensuring that cytotoxic temperatures are reached. It is expected that, in time, intralesional temperature-sensing technology will be incorporated into the microwave antennae themselves, as in the MedWaves, Inc. (San Diego, CA) microwave platform in development, eliminating this additional procedural step.
The second disadvantage to using microwave energy results from the confines imposed upon the treating radiologist by the limited selection of percutaneous antennae that are now available. The use of a single antenna, with a 3.7-cm active tip, results in the formation of an elliptical ablation zone centered 1.5 cm proximal to the distal tip of the antenna. A previously manufactured shorter active tip antenna, 1.6 cm, (Valley Laboratory/Covidien) allowed for the creation of a smaller, sphere-shaped zone of kill centered around the target lesion (Fig. 3.12). As this product is no longer commercially available, radiologists are limited in their strategic approach. For example, when treating a small pulmonary metastasis that abuts the pleura, accuracy becomes important. Thus, there remains a need for a greater variety of percutaneous antennae that are designed for the ablation of smaller lesions, and still offer the advantageous and improved conduction profile of microwave energy.
- Microwave radiation is part of the electromagnetic spectrum (along with radio waves, infrared waves, visible light, ultraviolet and x-radiation, among others), which exhibits a frequency of oscillation between 0.9 and 2.45 GHz.
- Microwave ablation is associated with larger ablation zone, faster ablation time, and less susceptibility to the heat-sink effect, compared with RFA.
- The direct ablation zone for MWA is approximately 2 cm from the probe compared with only a few millimeters for RFA. Beyond these margins, ablation depends on the frictional transfer of energy (conduction) between adjacent molecules.
- MWA, contrary to RFA, does not require the placement of grounding pads.
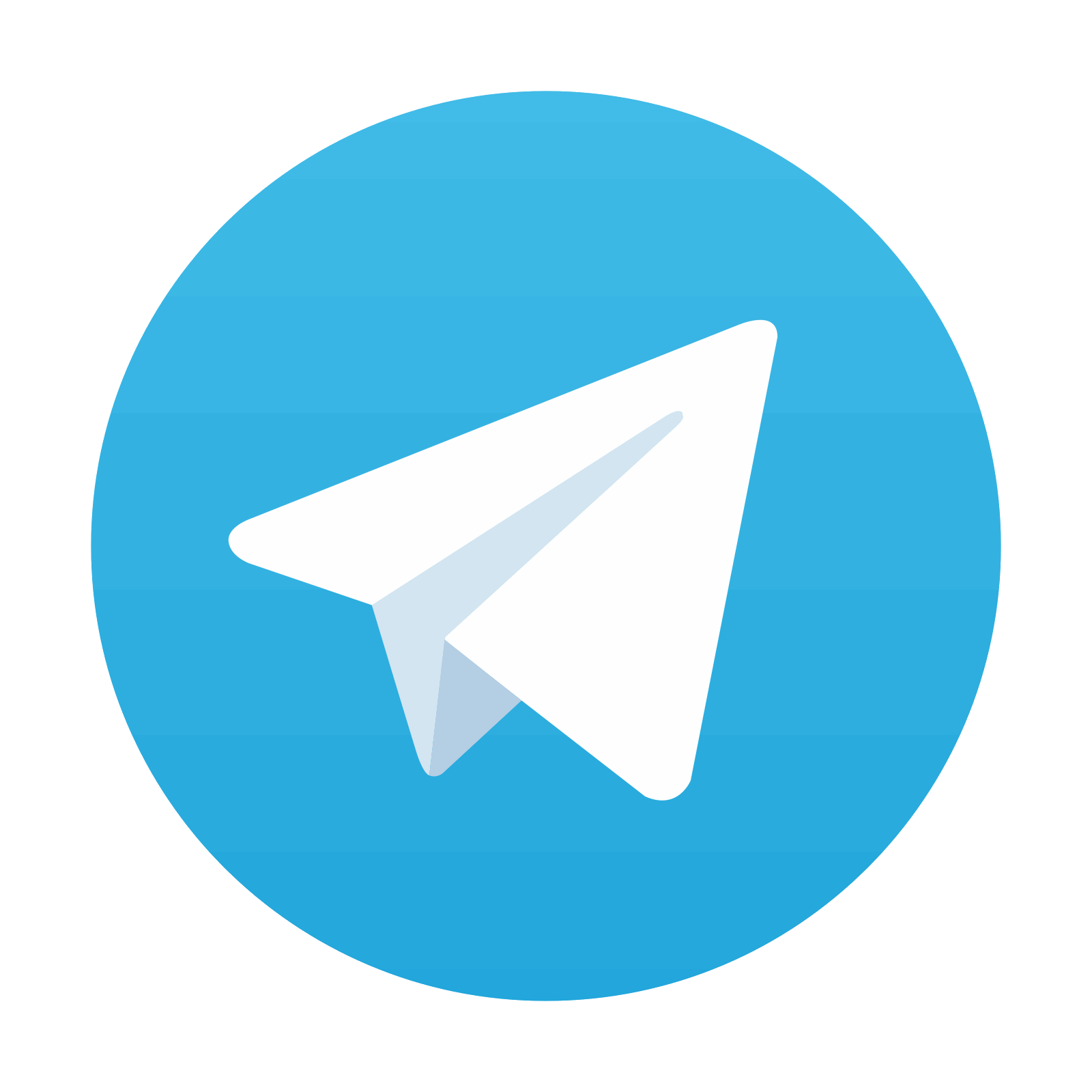