6
Percutaneous thermal tumor ablation (PTTA) is a minimally invasive treatment alternative for primary and secondary pulmonary malignancies. Thus far, the majority of ablative technologies focus on thermal, chemical, and cell permeability techniques, of which thermal energy has been the most studied and widely used in the thorax and lung. Thermal energy sources can be heat-based (radiofrequency and microwave) or cold-based (cryoablation). To date, radiofrequency ablation (RFA) has been the best developed, although continued advances in microwave and cryoablation technologies have led to their increased utilization and investigation with newly emerging technology-specific benefits.1,2 The emergence of more fully realized thermal ablative technologies, in culmination with image-guided, or minimally invasive, approaches, offers a valuable alternative, or at a minimum, a useful adjunct to conventional or limited surgical resection.
The indications for thermal ablation in the thorax as a standalone technique or in combination with other therapeutic options include stage 1 and 2 non–small cell lung cancer (NSCLC) both medically operable and inoperable candidates, carefully selected stage 3a NSCLC, reduction or debulking of tumor burden in anticipation of limiting vital structure involvement or enhancing eligibility for later resection, control of limited recurrent or metastatic disease, and palliation for pain control.
With refinements in technology, patient selection, clinical applications, and methods for follow-up, PTTA will continue to flourish, as a viable stand-alone or complementary therapy for both primary and secondary lung malignancies in standard and high-risk populations.
♦ Current Treatment Options and the Role of Ablation
The treatment of primary and secondary pulmonary malignancies has evolved into a multidisciplinary approach allowing for combination therapies and the use of novel chemotherapeutic agents, targeted radiotherapy, and thermal ablation. An overview of the current treatment options for primary and secondary pulmonary malignancies in the medically operable and inoperable patient is necessary to understand the role and benefit of percutaneous thermal ablation in relation to other treatment options.
Treatment options for NSCLC are multifactorial but based primarily on the stage and medical fitness of the patient. In stage 1 (tumor, node, metastasis [TNM] stages T1 N0 M0 and T2 N0 M0) and stage 2 disease (T1 N1 M0, T2 N1 M0 and T3 N0 M0), surgery is the treatment of choice with a 5-year survival of approximately 75% and 50%, respectively.3 In stage 3a disease (T1 N2 M0, T2 N2 M0, T3 N1 M0, and T3 N2 M0), radiotherapy, chemotherapy, surgery, and combinations of these modalities are used for treatment, with an overall 5-year survival that does not exceed 10 to 15%.3 In stage 3b disease (any T, N3, M0 and T4, any N, M0), surgery is of no benefit. A combination of chemotherapy and radiotherapy is used with a 5-year survival of less than 5%.3 In stage 4 disease (any T, any N, M1), palliative chemotherapy is used with a median survival at the time of diagnosis of only 7.9 months.4 Although surgery is the preferred method for stage 1 and 2 NSCLC,5 only one third of these patients meet pulmonary physiologic guidelines for lobar or sublobar resection (forced expiratory volume in 1 second [FEV1] >60% predicted and carbon monoxide diffusing capacity of the lungs [DLCO] >60% predicted).6 According to the Surveillance, Epidemiology, and End Results (SEER) database, 14,555 patients, or 15.7%, present with stage 1 and 2 lung cancer but are medically inoperable.7 For these candidates, sublobar resection, specifically wedge resection and segmentectomy, has been proposed, but because of its higher local recurrence rates compared with traditional lobectomy of up to 50%, sublobar resection is considered less favorable and has been viewed by many as a compromised operation.8 Despite comorbid medical illness, however, candidates who receive some form of therapy for early-stage lung cancer demonstrate better outcomes than those who do not receive any form of therapy.9 Therefore, a sizable proportion of patients with stage 1 and 2 lung cancer are medically inoperable and can benefit from less invasive and nonsurgical alternatives, such as percutaneous thermal tumor ablation.
Thermal ablation, in combination with radiation or chemotherapy, for inoperable candidates is being pursued and appears intuitive. Candidates who have been treated with combination therapy include those with limited local recurrence after enjoying a period of remission following chemotherapy and/or radiation and those with large initial tumor bulk, usually more than 3 cm, or tumor within a precarious location to vital structures, especially compelling for the use of combination therapy. Comparatively, conventional radiation alone in inoperable early-stage NSCLC has shown survival rates of approximately 30% at 5 years,10,11 although newer advances in radiation therapy, particularly stereotactic body radiotherapy offering highly focused and precise delivery of a higher biologically effective dose than conventional radiation, have shown promising results but have not yet gained widespread availability.12–14
In patients with NSCLC, radiofrequency ablation and radiotherapy are synergistic. A proposed mechanism is believed to be that RFA has a greater effect in the central portion of the tumor, which often contains areas of diminished oxygenation and therefore is less responsive to radiotherapy, and a lesser effect in the peripheral portion tumor with a surrounding zone of nonlethal heat injury that increases sensitivity to external-beam radiation.15 With the synergism of combined therapy, potential cure may be offered for larger tumors than with RFA alone (Fig. 6.1). Grieco and colleagues15 reported results for patients after thermal ablation (either RFA or microwave ablation) followed by radiation therapy (either external-beam radiation or interstitial brachytherapy) for stage 1 or 2 NSCLC. Overall survival rates were 86.8%, 70.4%, and 57.1% for 1, 2, and 3 years, respectively. Similar to earlier experiences, the reported survival rates for combination therapy were higher than those seen with RFA alone.15–17 Interestingly, the survival rates were similar for stage 1a and 1b, suggesting that radiotherapy may improve the efficacy of thermal ablation for the treatment of larger tumors (Fig. 6.1). However, and perhaps somewhat discouragingly, local recurrence remained higher in tumors larger than 3 cm.15
In regard to adjuvant therapy, cisplatin-based chemotherapy has demonstrated a survival benefit in patients with stage 2 disease and higher.18 However, no significant improvement in survival was seen in stage 1b disease, with a negative effect observed in patients with stage 1a disease, and up to 50% of patients with stage 1b NSCLC eventually develop disease recurrence despite initial treatment with curative intent.18 Chemotherapy alone is not indicated for early-stage lung cancer and should be accompanied with surgical resection or thermal ablation. Similarly, thermal ablation for early-stage lung cancer should be accompanied by adjuvant chemotherapy for better survival.
Fig. 6.1 Combined radiofrequency ablation (RFA) and radiotherapy. (A) Preablation computed tomography (CT) image demonstrates a dominant left lower lobe mass with an adjacent satellite nodule. (B) Preablation CT image shows interval placement of a triple-cluster Covidien Cool-Tip electrode in the dominant mass (arrow) and a single Covidien Cool-Tip electrode in the satellite nodule (arrowhead). (C) Postablation CT image demonstrates placement of a fducial marker in the dominant mass (arrow) for stereotactic body radiotherapy via a 19-gauge coaxial needle. (D) Contrast-enhanced 6-month follow-up CT image demonstrates no enhancement in the ablation zone (*), consistent with complete tumor necrosis with associated atelectasis (arrow) and a small pleural effusion (arrowhead).
In small cell lung cancer (SCLC), treatment is based on the extent of disease, with surgery being of no benefit for extensive disease. Extensive stage SCLC is treated with combination chemotherapy, with radiotherapy alone demonstrating no significant benefit. In limited-stage disease, combination chemotherapy is the cornerstone of treatment and is typically combined with radiotherapy with a median survival of 18 to 24 months and a 2-year survival of 40 to 50%.19,20Surgery plays a limited role in stage 1 SCLC. Currently, the effects of thermal ablation in limited SCLC has not been investigated but, seemingly as surgery, potentially could be used to treat peripheral limited stage 1 SCLC.21
Metastatic disease by solid tumors to the lungs is common, with the lung being the second most commonly affected organ, following the lymphatic system.22,23 Hematogenous metastases from nongastrointestinal tumors to the lungs occur with reasonable frequency, as the lung acts as the first capillary bed for blood-borne and lymphatic-borne dissemination.24 Approximately 20% of candidates with lung metastases, who have already undergone resection of the primary soft tissue tumor, have a limited number of metastases located only within the lung, rendering them potential candidates for surgical resection, or metastasectomy.23 Surgical metastasectomy results in improved disease-free survival in properly selected patients, particularly in those with metastatic colorectal carcinomas and sarcomas.25–27 In contrast to primary lung carcinoma where lobar resections and pneumonectomy confer increased rates of survival, no difference in survival outcome between wedge and lobar resections and pneumonectomy was seen in the metastasectomy population; therefore, resection with the least amount of loss to lung parenchyma has been advocated.28–30 Notably, percutaneous thermal ablation, with its minimal invasiveness and ability to spare lung, is an attractive alternative for those patients with limited metastatic disease who are medically inoperable; have limited recurrent or residual disease following prior surgical resection, chemotherapy, or radiotherapy; or deemed surgically inoperable from decreased lung volumes or pleural adhesions.
In simplistic terms, percutaneous ablation can be reasonably considered in those patients with surgical disease, whether primary or secondary malignancy, who are, for one reason or another, medically or surgically ineligible. The treatment of primary and secondary pulmonary malignancies in the inoperable patient will continue to evolve, with the role of thermal ablation being better defined as increasing long-term data emerges. It is clear that a multidisciplinary, or “team,” approach individualizing therapy to each patient’s disease will be the standard going forward, with thermal ablation being used as a monotherapy, or in combination with surgical resection, radiotherapy, and/or systemic therapy in carefully selected patients.
♦ Patient Selection, Tumor Selection, and Anatomic Considerations
Proper patient selection for PTTA is highly dependent on the anticipated goal to be achieved, with the understanding that thermal ablation provides only local control of cancer. Clinical indications, contraindications, and selection criteria are assessed together when evaluating patients for thermal ablation. Clinical indications are detailed in the previous section and principally include primary or secondary pulmonary or pleural malignancies, in which local control of tumor with and without curative intent as in debulking, reduction of total body tumor burden, or palliation of tumor and its effects is desired and sensible.
- Primary stage 1 or 2 lung cancer in medically inoperable tumors
- Secondary pulmonary malignancies in particular with limited colorectal and sarcoma metastasis
- Secondary pleural malignancies for local control with and without curative intent
There are few absolute and relative contraindications for thermal ablation, and, as a general rule, a patient who can undergo an image-guided biopsy can be considered a candidate for thermal ablation. Absolute contraindications, however, include severe pulmonary arterial hypertension (>40 mm Hg) and acute pneumonia. Relative contraindications include the inability to cooperate (lie flat and breath-hold), although rectified with general anesthesia and deep sedation; coagulation disorders/ medications; pacemakers/automatic implantable cardioverter-defibrillators (AICDs); and pneumonectomy, although some authors believe that thermal ablation can be safely performed even in this population if the proper preparations are made for rapid chest tube insertion.31,32
Although no strict criteria exist for patient selection, the emerging goals for ablation thus far established within the current literature include the potential for cure, prolongation of survival, and palliative relief of symptoms.
Candidates for whom there is a potential for cure include those with stage 1 or 2 NSCLC who are medically inoperable or refuse surgical resection, and those with limited or low-volume and indolent metastatic cancer from an extra-pulmonary primary malignancy, provided that the primary tumor site or extrathoracic sites of tumor are controlled. Candidates for whom there is a potential for prolongation of survival include those with pulmonary malignancy with limited disease outside the lung that can be treated with systemic therapy or locoregional methods, slowly growing pulmonary malignancy with the main bulk of tumor within the lung, and larger tumors where cytoreduction can alter the susceptibility of viable tumor tissue to chemotherapy or radiotherapy. Candidates for whom there is a need for palliation include those with painful peripheral tumors invading the parietal pleura or chest wall, those with tumors adjacent to vital structures as a preemptive strike before invasion, and those requiring cytoreduction of tumor volume to result in reduction of symptoms.
- Absolute: severe pulmonary arterial hypertension and acute pneumonia
- Relative: inability to cooperate (lie flat and breath-hold), coagulation disorders/medications, pacemakers/AICDs and pneumonectomy
- General rule: A patient who can undergo an image-guided biopsy can be considered for thermal ablation.
As with patient selection, strict criteria are also lacking for tumor characteristics favorable for thermal ablation, although clear trends have emerged. The ideal lesion is a solitary tumor that is completely intraparenchymal and ≤3 cm; treating tumors of this size results in higher rates of complete necrosis and ultimately improved survival.17,33 The combination of radiofrequency and targeted radiation has shown improved survival, specifically in lesions >3 cm, and should be pursued.15 For pleural and chest wall lesions, the use of cryoablation instead of heat-based ablative techniques has shown less patient
discomfort and complications.34
For patients with multifocal disease, a finite number of metastases at presentation is desirable, ideally less than six in total, although this is a somewhat arbitrary criterion that is loosely based on metastasectomy data showing diminishing gains with higher numbers of resections.35 Other important considerations regarding the target lesion include confinement within a single ablation zone, higher sphericity, and noncontiguity with the hilum, including large bronchi, pulmonary arteries and veins, or vital mediastinal structures, such as the trachea, esophagus, heart, aorta, and great arteries. If one is considering the use of radiofrequency energy, additional forethought regarding the potential for the heat-sink effect from adjacent pulmonary arteries and veins >3 mm36 or large bronchi37 is necessary to avoid incomplete or partial ablation. However, with advances in microwave and cryoablation technology, the heat-sink effect can be minimized or obviated altogether. The texture of the lesion can also be important, as extremely dense or fibrotic lesions may be mobile or demonstrate a “push-back” phenomenon described by Steinke et al,38 in which the shaft of the electrode is continually pushed back with attempted tine deployment.
- Solitary tumor that is completely intraparenchymal and <3 cm
- In multifocal disease a finite number of metastases, ideally <6
- Confinement to single ablation zone
- Noncontiguity with the hilum and mediastinum
♦ Anatomic Considerations for Thoracic Ablation
Multiple anatomic factors should be taken into account when developing an approach for a percutaneous CT-guided pulmonary ablation. Similar to percutaneous CT-guided lung biopsy, site of entry, adequate pleural anesthesia, and awareness of the bronchovascular planes, the fissures, and the mediastinal structures is critical in maximizing patient comfort and increasing the success of the ablation while minimizing the risk for significant complications.
Initial adequate placement of the coaxial needle is essential for achieving pleural anesthesia and optimal trajectory for tandem placement of ablation electrode. Adequate pleural anesthesia is the cornerstone of successful ablation, as patient comfort and cooperation are of the utmost importance. The parietal pleural is the second most sensitive structure aside from the skin in the path of the ablation electrode. The costal and diaphragmatic parietal pleura is innervated by the overlying intercostal nerve, where inadequate anesthesia is characterized by significant pain and discomfort along the dermatome or referred pain to the ipsilateral shoulder. Effective anesthesia of the parietal pleura requires the precise placement of the anesthesia needle tip in an extrapleural location immediately adjacent to and superficial to the parietal pleura, while avoiding both the underlying parietal and visceral pleura, which if violated increases the risk of pneumothorax (Fig. 6.2). Proper needle tip placement requires the combination of exact measurements obtained from localization scans and operator needle control. As the needle penetrates the different layers of the chest wall, the operator will feel many “pops,” representing the needle traversing the different chest wall structures, the most important of which is the endothoracic fascia as its passages signifies the exact location of the extrapleural fat space immediately superficial to the parietal pleura, where a few millimeters can mean the difference between a straightforward ablation versus one complicated by a premature pneumothorax (Fig. 6.3).
An understanding of the central bronchovascular computed tomography (CT) anatomy provides a safe transthoracic route to a central lesion. The pulmonary hila are composed of the major bronchi with accompanying pulmonary arteries and pulmonary veins that extend radially into the lobes, segments, and subsegments of the lungs. It is often difficult to avoid the vessels and airways in the periphery of the lung, but fortunately, such disturbances result in minimal to no significant complications. For central lesions, when developing a percutaneous transthoracic route, the radial nature of the bronchovascular structures from the hila to the periphery of the lung typically provides an abronchovascular route to the central portions of the lung and thus allowing safe access to a centrally located lesion (Fig. 6.4). By exploiting this abronchovascular plane, the risk of a threatening pulmonary hemorrhage with or without hemoptysis, bronchovascular, bronchopleural fistulas, and, in a worst-case scenario, air embolism can be significantly reduced. In addition to conventional axial images, sagittal and coronal reformations can better delineate the relationship of the target lesion with the surrounding bronchovascular structures and may be beneficial in developing a safe transthoracic route. Of particular interest is the right upper lobe vein tributary (posterior vein) lying in the angle between the anterior and posterior segmental branches, which is present in almost all patients. The clinician should note the possibility of an anomalous pulmonary vein branch seen posterior to the bronchus, which requires special attention so as to avoid injuring it.
Fig. 6.2 Pleural anesthesia: two different patients undergoing percutaneous thermal tumor ablation (PTTA). (A,B) Preanesthesia: a 19-gauge coaxial needle with the tip (arrow) superficial to the extrapleural fat (arrowheads). (C,D) Postanesthesia: a 19-gauge coaxial needle has been advanced with the tip in the extrapleural fat (arrows) status post-administration of 1% lidocaine in the extrapleural space (arrowheads).
As in percutaneous CT-guided lung biopsy, awareness of the interlobar and accessory fissures is important, as these fissures should be avoided to reduce the risk of a pneumothorax, which may result in a problematic or persistent air leak. The fissures are composed of the major, minor, and accessory fissures. The fissures are best visualized on thin, less than 3 mm, CT sections; they appear as a white line that typically can be avoided when developing a path to the target lesion. However, if thin sections are not available, fissural position can be inferred from the location of a 1- to 2-cm-thick avascular plane of lung. The minor fissure is usually difficult to visualize, as it parallels the plane of the scan, but it can be readily visualized on coronal and sagittal reconstructions. The fissures are not always complete, and are often partial. Aziz et al39 catalogued in a large study population the incidence of partial or absent fissures, and found centrally absent right major, left major, and right minor fissures in 48%, 43% and 63% of patients, respectively. Once recognized, incomplete fissure anatomic variation can be taken advantage of while planning a translobar approach to a central lung target (Fig. 6.5). Accessory fissures, seen in up to 30% of individuals,40 should also be considered while planning an approach; common accessory fissures include the inferior accessory fissure that separates the medial basal segment from the remaining basal segments, the azygos fissure (composed of four layers of pleura: two visceral and two parietal) that represents an invagination of the right apical pleura by the azygos vein, the superior accessory fissure that separates the superior segment from the basal segments of the lower lobe, and the left minor fissure that separates the lingula from the remaining portions of the upper lobe.
Fig. 6.3 Early pneumothorax. (A) Preablation CT image demonstrates 8-mm nodule (arrow) within the left upper lobe with a 19-gauge coaxial needle in the chest wall (arrowhead). (B) Following advancement of the coaxial needle, the tip (arrow) is seen 3 mm into the thoracic cavity, resulting in a premature pneumothorax. (C) Repeat imaging demonstrates a persistent air leak requiring chest tube placement prior to ablation.
Fig. 6.4 Abronchovascular plane. (A) A 51-year-old man with colorectal carcinoma with a 9-mm right lower lobe centrally located metastasis (arrow). (B) Preablation CT image demonstrates a 19-gauge coaxial needle tip in the extrapleural space post–lidocaine administration (arrow). (C) Using the 19-gauge coaxial needle as an approximate guide a single Covidien Cool-Tip electrode is advanced between the bronchovascular bundle medially (arrow) and pulmonary vein (arrowhead) laterally. (D) Utilizing the abronchovascular plane, the electrode is advanced into the centrally located metastasis (arrow).
Lesions adjacent to or within the mediastinum must be approached and ablated with caution, as they pose a risk of injury to mediastinal structures and incomplete ablation from the heat-sink effect. Structures that should be noted when planning a percutaneous thermal ablation include the trachea, aorta, great vessels, superior and inferior vena cavae, heart and pericardium, and esophagus. In addition, depending on the approach and location of the lesion, note should be made of possible injury to neural structures such as the brachial plexus (apical), the left recurrent laryngeal nerve (mediastinal), and the phrenic nerves (juxtamediastinal). Patients and clinicians must be made aware of the potential for injury and the possibility of long-term sequelae following ablation.
- Proper placement of coaxial needle is essential for achieving pleural anesthesia and optimal trajectory including tandem placement of the ablation needle.
- Abronchovascular route and incomplete fissures provide safe access to central pulmonary lesions.
- Ablation near mediastinal structures must be approached and performed cautiously, with great care taken around neural structures.
Fig. 6.5 Incomplete fissure. (A) Baseline CT image shows a centrally located 1.5 cm nodule (*) in the left upper lobe with an incomplete oblique fissure (arrow) seen posterior and lateral to the pulmonary nodule. (B) The incomplete fissure is again seen (arrow) allowing the RFA electrode to approach the left upper lobe nodule (*) via the preferred posterior approach through the left lower lobe. Coronal (C) and sagittal (D) multiplanar reconstructions were performed to confrm that the oblique fissure is incomplete (arrows) prior to electrode placement.
♦ Technique: A Step-by-Step Approach
Preprocedure Evaluation
Evaluation of a potential candidate for PTTA is preferably performed in an outpatient setting. Directed patient history and physical examination are necessary, with special attention given to the patient’s cardiopulmonary status and ability to cooperate, including the ability to perform adequate breath-hold and the ability to lie still for an extended period of time. A thorough evaluation of the patient’s medication history is important, with attention paid to anticoagulant and antiplatelet medications, as these need to be discontinued prior to the procedure. Patients on warfarin require conversion to subcutaneous or low molecular weight heparin, which is then stopped at least 24 hours before the procedure. Review of a recent electrocardiogram and pulmonary function tests is particularly important in patients with comorbid heart or lung disease or prior resection. Many patients with lung cancer are or have been smokers, and pulmonary function tests are required to establish adequacy of oxygenation, pulmonary reserve, and flow volume spirometry. Recent, ideally less than 4 weeks, CT scans should be available to ensure amenability of tumor size, and to determine the number of tumors requiring ablation. CT also helps map out a safe access route, and demonstrates comorbid disease in the lung and the location and relationship of the tumor to vital structures. In cases of primary lung cancer, current staging with whole-body positron emission tomography (PET) or mediastinoscopy, if the patient is able, is essential.
Histopathologic diagnosis of the lesion should be obtained before the ablation. Biopsy can be performed preferentially as a separate session prior to ablation or on the same day. Concurrent sessions are less preferred because of prolongation of procedure time and the possibility of complications from the biopsy that can delay or ultimately postpone the ablation. In addition postbiopsy hemorrhage along the needle tract and around the targeted tumor may obscure tumor margins for accurate and adequate electrode placement and make the detection of the immediate postablation zone, as demonstrated by surrounding ground-glass opacity, less accurate.
The presence of a pacemaker or AICD must be ascertained, as radiofrequency and, to a lesser extent, microwave current can cause potential device malfunction. The possibility of coagulation necrosis at the myocardial implantation site seems invalid, likely from a massive heat-sink effect of cardiac ventricular blood flow. Patients dependent on a pacemaker for a small percentage of time can have temporary deactivation with temporary cardiac pacing available on standby. However, patients with constant dependence on the pacemaker may benefit from alternative modalities, specifically cryoablation and microwave ablation, depending on the tumor location.
- Outpatient evaluation with directed history and physical focusing on:
- Cardiopulmonary status
- Ability to perform adequate breath-hold and to lie still for extended periods
- Medication history, including anticoagulant and antiplatelet medications
- Cardiopulmonary status
- Recent CT with or without PET to radiographically stage, ensure amenability, and develop route
- Histopathologic diagnosis should be obtained preferably as a separate session.
Anesthesia
Thermal ablation has been performed primarily as an outpatient procedure under conscious sedation, with a typical conscious sedation protocol that includes midazolam, morphine sulfate, and fentanyl citrate. Operators have at times favored deep conscious sedation and even general anesthesia,41 particularly in patients undergoing radiofrequency, and less so with microwave ablation, for targeted lesions involving the pleura or chest wall, which can be extremely painful both during and after the ablation. No consensus, for the most part, exists among authors for sedation; each operator’s decision is dependent on many variables, including comorbid disease, tumor location, and risk for potential complications.38,42–44 The advantages of general anesthesia include greater degree of intraprocedural patient comfort, better control of the airway, and the presence of an expert in cardiopulmonary management in case of a complication. The disadvantages of general anesthesia include higher cost, logistical challenges requiring a second participating service, longer procedure times related to anesthesia setup and availability, higher potential for pneumothorax due to positive pressure ventilation, and the risks specific to general anesthesia. As an alternative to general anesthesia, deep conscious sedation offers the advantages of lower cost and shorter procedure times, and the disadvantages of potentially less patient comfort and procedural pain control during the procedure. In addition, special attention must be paid to patients with severe chronic obstructive pulmonary disease who require supplemental oxygen and are at increased risk for respiratory depression when placed on higher percentages of oxygen during conscious sedation.31
Day of the Procedure
An informed consent for the procedure and other specific interventions should be obtained, detailing the benefits, risks, and alternatives. Laboratory data, including prothrombin time, partial thromboplastin time, international normalized ratio, and occasionally bleeding time are required the day of the procedure or at least within the past 7 days, especially if the patient is on anticoagulation. A complete blood count, including hemoglobin and hematocrit, white count, and, most importantly, platelets, is recommended. Creatinine should be obtained if the administration of iodinated contrast is required to delineate vascular structures and tumor margins. Prophylactic antibiotics are given routinely by some interventionists 1 hour before the procedure and in the postprocedural period, the argument being that devitalized tissue at the end of ablation is a potential nidus for infection. However, the benefits of prophylaxis have yet to be proven.
For the lung, CT is the most widely used modality for directed and precise needle placement. Volumetric acquisition of imaging data allows for computer reconstruction of three-dimensional volume data sets for pre-, intra-, and postprocedure analysis. Multiplanar reformatted (MPR) display of images both parallel to (long axis views) and perpendicular to (short axis views) the axis of the needle can provide valuable information regarding depth of device penetration; the exact position of the electrode relative to the target lesion and its margins, especially in the z-axis; the configuration of multiple electrodes; and the relationship to essential and nontargeted structures (Fig. 6.6).45 The principal limitation of CT is the lack of real-time imaging feedback for advancement of devices into the targeted tumor, although some operators have found CT-fluoroscopy to be a useful adjunct for device placement.
- MPR display of images both parallel to and perpendicular to the axis of the needle can provide valuable information regarding:
- Depth of device penetration
- Exact position of the electrode relative to the target lesion and its margins
- Relation to essential and nontargeted structures
- Depth of device penetration
Patient positioning on the CT table warrants special attention. In general, the shortest distance from skin to the tumor is optimal, although a lateral approach is less favorable due to increased and opposite motion of ribs to the lungs during breathing, making needle placement difficult and raising the potential for air leak. When equivocal, a posterior approach with the patient in the prone position is preferable to an anterior approach with the patient in the supine position, because the posterior ribs are less subject to respiratory variation, maintaining a more consistent intercostal window. In general, access should pass over the superior margin of the rib to minimize the probability of intercostal artery or vein injury, should avoid traversing bullae or interlobar fissures, and should avoid injury of essential nontarget structures. Care should be taken when positioning the upper extremities to avoid injury to the brachial plexus. Off-center positioning of the patient on the CT table may be required to provide sufficient clearance between the chest wall and the inner surface of the CT gantry to accommodate the RF electrode, microwave antenna, or cryoablation probe. The CT table should be as low as possible to maximize gantry clearance.
Fig. 6.6 Cryoablation: axial, coronal, and sagittal multiplanar reformats. (A) A 48-year-old woman with a 7-cm right apical mass consistent with mesothelioma recurrence (*), who presents with brachial plexus symptoms. Axial (B), coronal (C), and sagittal (D) multiplanar reformats allow for precise placement of four cryoprobes with development of an ice ball that completely encompasses the target lesion.
- Posterior approach provides a more consistent intercostal window.
- Lateral approach should be avoided due to increased rib motion with breathing.
- When equivocal the posterior approach with the patient in the prone position is preferred over the anterior approach with the patient in the supine position.
For radiofrequency ablation, dispersive grounding pads with large surface areas must be applied to the patient’s body, preferably perpendicular to the RF electrode path and not located over bony prominences. The grounding pads should be in good contact to the skin, and, if necessary, the skin should be shaved. Grounding pads are usually placed over the thighs (Fig. 6.7). When more than one electrode or cluster electrodes are used, at least two extra grounding pads are required to prevent cutaneous pad burns and falsified increased impedance. The upper extremities and fingers lying close to grounding pads should be insulated to avoid alternate circuit and finger burns.
- Pads are placed on thighs, perpendicular to RF electrode path while avoiding bony prominences.
- For each additional electrode, at least two extra grounding pads are required.
- Use towels or blankets to insulate the upper extremities from the adjacent grounding pads to avoid alternate circuit and finger burns.
- During ablation, the operator must ensure that perspiration has not caused the pads to peel away from the skin, as skin burns can occur.
Fig. 6.7 Radiofrequency ablation grounding pads placed over the thighs status post-shaving.
Intraprocedural Technique
Initial CT is performed, and the site of entry marked, using a radiopaque grid or radiopaque marker like a lead ball (Fig. 6.8A). Once the skin entry site is confirmed, it is prepped with antiseptic solution and draped with sterile towels. Consider a wider area of preparation to allow for possible chest tube placement and additional localization needles or electrodes. After cutaneous anesthesia is achieved, a coaxial or Chiba needle is directed to the pleural surface (Fig. 6.8B), where liberal local anesthetic is deposited to achieve pleural anesthesia, as previously described (see Anatomic Considerations for Thoracic Ablation, above).
Two techniques have been used successfully to place the RF electrode into the targeted tumor. The first option requires placement of a 21- or 22-gauge localizing needle, 10 to 15 cm in length, into the tumor (Fig. 6.9). The thinner localizing needle is thought to be associated with a lower risk of pneumothorax and hemorrhage than the larger gauge electrode, should multiple attempts be needed to obtain optimal device position. It can also be used to obtain biopsy samples if already not obtained or documented. Once the small gauge localizing needle is situated satisfactorily within the tumor, it can be used as a guide for advancement of the larger gauge electrode through the tandem needle technique, whereby the electrode is simply passed along the same tract as the initially placed localizing needle into the tumor target. Because the localizing needle is not electrically insulated, it must be removed prior to starting radiofrequency, microwave, or cryoablation (Fig. 6.9).42 Alternatively, as a second option, some operators advance a 19- or 20-gauge coaxial biopsy cannula to the pleural surface; this larger needle enables better delivery for pleural anesthesia and also serves as an approximate, although not definitive, guide to the targeted tumor. Subsequently, the electrode is placed, and once it is into the pulmonary parenchyma, it is directed into the lesion under CT guidance with or without fluoroscopic assistance (Fig. 6.8C,D).
Fig. 6.8 Radiofrequency ablation technique. (A) A 2.7-cm left lower lobe lung adenocarcinoma (arrow) with entry site localization with a radiopaque ball (arrowhead). (B) After cutaneous anesthesia is achieved, a coaxial needle is directed to the pleural surface for pleural anesthesia. (C) Utilizing the coaxial needle as an approximate guide, a triple-cluster Covidien Cool-Tip electrode (arrow) is placed into the pulmonary nodule. (D) Postablation CT image demonstrates perinodule air space and ground-glass opacity enveloping the target lesion (arrows) with a cauterization tract seen (arrowhead).
Fig. 6.9 Radiofrequency ablation technique in a 73-year-old man with a 3.2-cm left upper lobe squamous cell lung carcinoma. (A) A 22-gauge Chiba needle is placed for initial localization. (B) Via tandem needle technique, a RITA StarBurst XL Semi-Flex expandable electrode is placed at the leading edge of the cancer. (C) The electrode is expanded to 2 cm at the start of staged deployment and ablation. (D) After reaching target temperature, the electrode is further deployed to 3 cm. (E) After reaching target temperature, the electrode is finally deployed to 4 cm until ablation is completed, with the ablation zone demonstrating a vertical oval relative to the shaft of the electrode. (F) Postprocedural image demonstrates ground-glass parenchymal attenuation encompassing the target tumor in its entirety, the lateral ablation zone abutting the adjacent costal pleura.
- Two techniques:
- 21- or 22-gauge localizing needle directly into the tumor: exact guide to the tumor; used to obtained biopsy
- 19- or 20-gauge coaxial biopsy cannula to the pleural surface: better delivery of pleural anesthesia; approximate guide to the tumor
- 21- or 22-gauge localizing needle directly into the tumor: exact guide to the tumor; used to obtained biopsy
- Adequate placement ensures homogeneous coagulation necrosis of the entire tumor as well as 5 to 10 mm of surrounding nontumorous lung.
For radiofrequency ablation, electrode selection depends on operator preference. Three electrodes have been most widely used, each electrode unique in its design and ablation zone geometry. The LeVeen electrode (Boston Scientific, Natick, MA) deploys 10 tines concealed within the shaft of the electrode. Once the tip of electrode is in position, the tines are deployed initially expanding laterally, then arcing backward forming an umbrella-like array (Fig. 6.10A). The LeVeen electrode is optimally deployed when its tines arise from the center of the target tumor and extend immediately beyond the peripheral edges of the tumor. The resultant ablation zone appears as a horizontal oval relative to the shaft of the electrode (Fig. 6.11). The tines of the Starburst and Side-Deployment (SDE and Talon) type electrodes (AngioDynamics Inc., Queensbury, NY) emanate forward from either the tip (Starburst) or the side of the electrode (SDE), laterally similar to the stems in a bouquet of flowers (Fig. 6.10B). With this design, the tines are deployed from the electrode shaft at the near aspect of the tumor and then advanced until they extend beyond the edges of the tumor. The ablation zone achieved is a vertical oval, oriented to the shaft of the electrode (Fig. 6.9). The straight internally cooled single-electrode or triple-cluster electrodes (Covidien, Boulder, CO; Fig. 6.10C) are placed with the active exposed tip situated in the deep portion of the tumor and extending 0.5 to 1.0 cm into nontumorous lung beyond the far edge of the tumor. The resultant ablation zone is a vertical oval but more spherical than the ablation zones created by the AngioDynamics electrodes (Fig. 6.12). Single-needle electrodes can be used alone or simultaneously, with up to two additional needles through the addition of the “switch box” to create a larger ablation zone. If multiple electrodes are used, each electrode should be placed no further than 2 cm apart within the tumor and no further than 1 cm from the margin of the tumor to ensure creation of a proper surgical margin (Fig. 6.13). The ablation zone for each of the electrodes currently available on the market varies based on the duration of ablation and the size of the live portion of the electrodes, and can be obtained from the vendors directly; this information is also usually available in the electrode packaging (Fig. 6.14).
Fig. 6.10 Radiofrequency ablation electrodes. (A) The LeVeen electrode (Boston Scientific, Natick, MA) employs an expandable multiarray, with all tines initially contained within the electrode shaft. Once the tip of the shaft is in position, the tines are deployed, arcing horizontally and backward forming its umbrella-like array. (B) The Starburst XL electrode (AngioDynamics Inc., Queensbury, NY) also employs an expandable multiarray, with its tines coursing forward from the shaft tip. Once deployed, the completed array resembles the stems in a bouquet of flowers. (C) The internally cooled single-needle electrode or triple-cluster electrode (Covidien, Boulder, CO) utilizes an active tip of varying length that determines the size of the ablation zone.
Once the electrode(s) is in position, radiofrequency ablation is started. The goal is to achieve homogeneous coagulation necrosis of the entire tumor as well as an adjacent 5 mm, but ideally greater than 10 mm, surrounding margin of nontumorous lung (Fig. 6.12). Each device vendor has provided end-point parameters that assume adequate ablation. Repeat ablations can be performed if these end points are not achieved. The Boston Scientific system increases the electrical power (in watts) delivered in a stepwise fashion at prescribed time intervals until the impedance (ohms) increases, precipitously shutting off power delivery, a phenomenon affectionately termed “roll-off.”41 The AngioDynamics generator provides full power at 250 W. When the average tissue temperature exceeds target temperature, usually 90°C, as detected by thermocouples located in alternate tines, the tines are advanced as a staged deployment until the desired array diameter is achieved. Following manufacturer recommendations, ablation is continued at target temperature until specified ablation end times are achieved. The Covidien system gradually increases electrical power until an impedance rise of 20 Ω over baseline is detected, at which time power delivery is reduced. The heating cycle is terminated after an ablation time of 12 minutes. The tissue temperature, as measured by an embedded thermocouple, should exceed 70°C immediately after power termination and remain above 60°C for at least 3 minutes. If sufficient end temperature is not reached, additional overlapping ablations are performed.
Irrespective of the electrode used, imaging end points can be helpful to determine adequate ablation, specifically the appearance of ground-glass opacity on CT encompassing the treated tumor in its entirety and extending at least 5 mm, or a more confdence-inspiring >10 mm, beyond the periphery of the lesion (Fig. 6.12). Larger ground-glass margins have been associated with better local control rates.17,46 With experience, authors have favored the concept of “overablating,” in some cases achieving an immediate postprocedure ablation zone at least four times larger than the initial tumor to better predict complete ablation.46
- Ground-glass opacity on CT encompassing the tumor and extending 5 to 10 mm beyond the periphery of the lesion
- Overablation favored as larger ground-glass margins are associated with better control rates
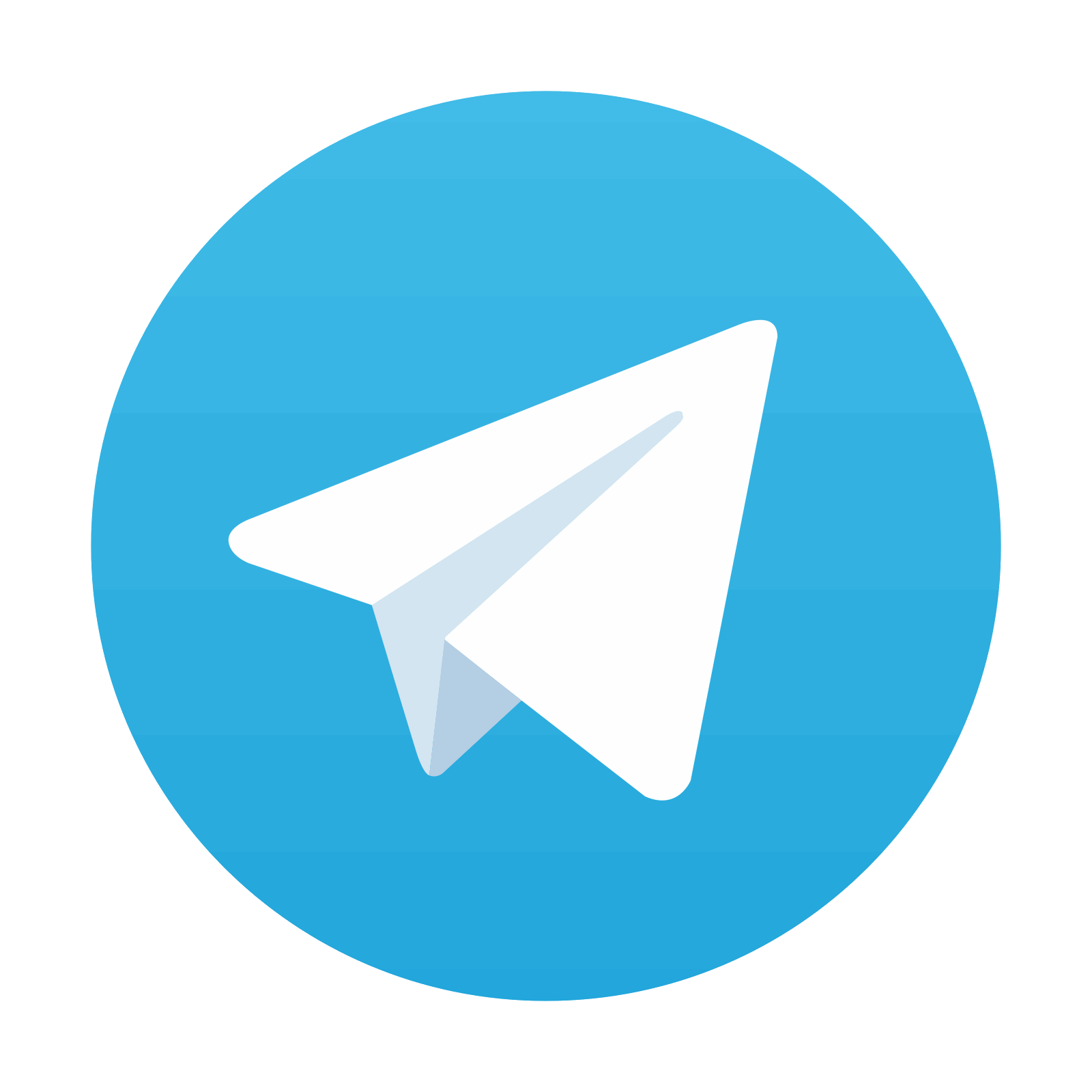