![]() FIGURE 9.1. A. Basophils are “cells with the kinetics and natural history of granulocytes that mature in bone marrow, circulate in the blood, and retain certain characteristic ultrastructural features, even after migrating into the tissues during inflammatory or immunologic processes. The ultrastructure of mature basophils varies according to species but generally includes electron-dense cytoplasmic granules, prominent aggregates of cytoplasmic glycogen, and short, blunt, irregularly distributed plasma membrane processes. There is no convincing evidence that mature basophils, whether in the circulation or in the tissues, retain mitotic capability, or that basophils metamorphose into mast cells upon entering the tissues.” (Galli SJ, Dvorak AM, Dvorak HF. Prog Allergy 1984;34:1-141). As shown in Figure 9.1A, human basophils are round and have irregular, short surface projections, cytoplasmic secretory granules, and aggregates of cytoplasmic glycogen. N, nucleus; bar, 1 µm. (Reproduced with permission from the authors and Blackwell Science Ltd., Dvorak AM, Warner JA, Fox P, et al. Recovery of human basophils after FMLP-stimulated secretion. Clin Exp Allergy 1996; 26:281-294.) B. Mast cells “ordinarily mature outside of the bone marrow or circulation, generally in the connective tissues or serous cavities. Cells in this lineage(s), wherever distributed, apparently retain at least limited or latent proliferative capacity…(I)mmature and mature granules of mast cells and basophils differ distinctively in ultrastructure. Mast cells also differ from basophils in lacking electron-dense aggregates of cytoplasmic glycogen, and in having a plasma membrane surface with uniformly distributed, thin, elongate folds and processes. Mast cell nuclei may appear bilobed in an individual photomicrograph, but they generally lack the pattern of peripherally condensed nuclear chromatin characteristic of basophils and other granulocytes.” (Galli SJ, Dvorak AM, Dvorak HF. Prog Allergy. Basophils and mast cells: morphologic insights into their biology, secretory patterns, and function. 1984;34:1-141). A human skin mast cell is shown with monolobed nucleus with partially condensed chromatin, numerous cytoplasmic granules containing crystalline structures and regularly distributed, narrow, thin surface projections. Bar, 1 µm. (Reproduced with permission from the author and Springer-Verlag. Dvorak AM. Human mast cells. In: Beck F, Hild W, Kriz W, et al., eds. Advances in anatomy, embryology and cell biology. Leicester: Springer-Verlag, 1989;114:1-107.) |
cells,” and the corollary, that mast cells are “tissue basophils,” although imprecise from a strictly developmental standpoint, is still of some value in thinking about the nature of these two cell types. The striking inverse relationship between the numbers of circulating basophils and the numbers of tissue mast cells has been used for decades to infer similarities in function.1
in the brain,33 in psychosocial stress,34 and in acute gastritis,35 and may be a component of responses to Toll-like receptor (TLR) activation.4 In inflammatory reactions in which mast cell and basophil infiltration occurs, such as in cutaneous delayed hypersensitivity, piecemeal degranulation can occur. As with anaphylactic degranulation, piecemeal secretion is associated with the ability of mast cells to recharge their granules and function again.
![]() FIGURE 9.3. Change of lineage model of hematopoiesis. A. In the old view, the presence of mast cell committed progenitors (MCP) was not clear, but mast cells were considered to be the progeny of common myeloid progenitors (CMP) without convincing evidence. Therefore, MCP is put in brackets. B. In the new view proposed by Chen et al.23 the presence of MCP is clear, and MCP is directly derived from multipotential progenitors (MPP). The thick line shows the main differentiation route from MPP to mast cell. CLP, common lymphoid progenitor; GMP, granulocyte/macrophage progenitor; Pro B, progenitor B cell; Pro T, progenitor T cell; B, B cell; NK, natural killer cell; T, T cell; MO, monocyte/macrophage; GR, granulocyte; E, erythrocyte; PL, platelet; MC, mast cell. (Reproduced with permission, Proc Natl Acad Sci U S A 2005;102:11129-11130 © 2005 National Academy of Sciences, U.S.A74). |
interleukin-3 (IL-3) is known to contribute to murine mast cell and to both human and rodent basophil development,55,56,57 it is SCF or c-kit ligand58 which uniquely drives human mast cell differentiation, and not other cytokines that may help promote mast cell development in rodents, such as IL-3, IL-4, IL-9, IL-10,59 and nerve growth factor (NGF).60 SCF is produced by murine and human fibroblasts, epithelial cells, endothelial cells, and tumor cell lines,61 and must bind to c-kit to effect differentiation. Mutations in c-kit can result in mast cell deficiency in vivo and in vitro (“loss-of-function”) or, alternately, in autonomous mast cell growth (“gain of function”), generally leading to autophosphorylation.62 The latter mutations, especially the D-816-V-associated mastocytosis (which can be transferred with similar consequences to the mouse62) have formed the basis of newly designed tyrosine kinase inhibitors in some proliferative mast cell and hypereosinophilic disorders.
TABLE 9.1 CYTOKINES AND OTHER FACTORS INVOLVED IN BASOPHIL AND MAST CELL GROWTH AND DIFFERENTIATION | ||||||||||||||||||||||||||||||||
---|---|---|---|---|---|---|---|---|---|---|---|---|---|---|---|---|---|---|---|---|---|---|---|---|---|---|---|---|---|---|---|---|
|
![]() FIGURE 9.4. Mast cell and basophil ontogeny. An orderly sequence of differentiation is depicted, beginning with a primitive CD34+, FcεRI−, c-kit− hemopoietic stem cell (HSC), and proceeding through various stages of commitment to either mast cell or basophil/eosinophil lineages. In reality, the differentiation process is stochastic, and multiple cytokines binding to their receptors permit specific, end-stage differentiation to proceed. For more detailed phenotypic analyses of basophil and mast cell progenitors, see Wang et al. 37 |
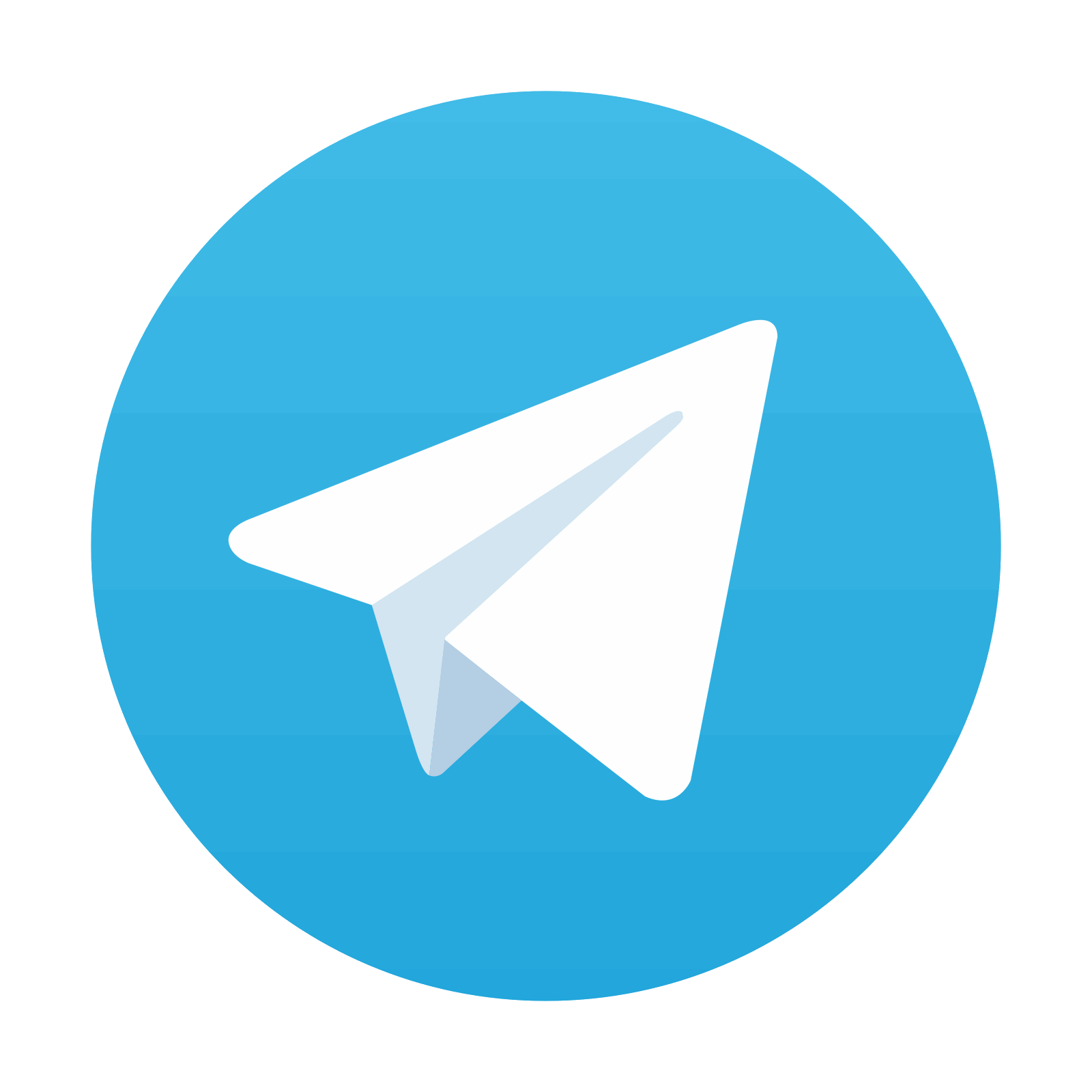
Stay updated, free articles. Join our Telegram channel

Full access? Get Clinical Tree
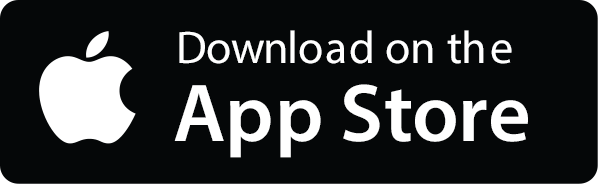
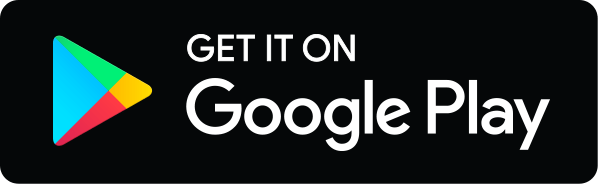
