Lymphocytes and Lymphatic Organs
Frixos Paraskevas
LYMPHOCYTES: HISTORICAL PERSPECTIVE
In the early part of the 20th century, the main debate about the nature and function of the lymphocyte centered around its relationship to cells of the connective tissue and cells of inflammatory exudate. Lymphocytes were considered capable of transforming into granulocytes, monocytes, macrophages, fibroblasts, and other cells. Although Marschalko,1 Downey,2 Maximow,3 and Bloom4 believed that lymphocytes gave rise to plasma cells, the scientific evidence for these assumptions was lacking; and Rich (1936) suggested that “The complete ignorance of the function of this cell is one of the most humiliating and disgraceful gaps in all medical knowledge.”5 The first conclusive evidence that antibodies are formed in the lymph nodes (LNs) was presented in 1935 by McMaster and Hudack,6 but it took another decade to prove that lymphoid cells in LNs were the cells that contained antibody.7,8 This discovery tipped the balance in favor of the lymphocytic theory of antibody production.9
In parallel with these studies, the classic work of Fagraeus brought strong indirect evidence of the relationship of plasma cells to antibody production.10 The demonstration with immunofluorescence techniques by Coons et al. of the presence of antibodies in plasma cells is a landmark in the long debate on the origin of antibodies and the function of plasma cells.11,12 The question whether plasma cells arise from lymphocytes was answered later by the elegant ultrastructural studies of Harris et al., who by means of electron microscopy demonstrated the gradual change of a lymphocyte to an antibody-forming plasma cell.13,14,15
Lymphocytes are a heterogeneous population of cells which differ greatly in terms of origin, life span, preferred areas of settlement within the lymphoid organs, surface structure, molecular markers, and function.16
Certain morphologic characteristics, such as size, granularity, and nucleocytoplasmic ratio, distinguish a variety of lymphocyte populations, but provide no clues to identification of their lineage and function. A precise and quantitative method used in clinical laboratories is based on detection of certain glycoproteins displayed on the membrane of lymphocytes known as markers. Two discoveries have helped in the dissemination of routine application of lymphocyte marker analysis in clinical medicine, the development of monoclonal antibodies and the invention of flow cytometry.
LYMPHOCYTES
Light Microscopy
Most blood lymphocytes are small (10 µm or smaller) but larger forms are common (Fig. 11.1A). Some of the large lymphocytes are known as large granular lymphocytes and contain azurophilic granules in their cytoplasm (Fig. 11.1B).17,18 The dimensions of the cells vary according to the method of preparation. In blood smears stained with Romanovsky dyes, the nucleus is deep purple-blue, usually round or slightly indented, composed of dense aggregates of chromatin. Nucleoli are not visible in Giemsa stained smears with ordinary techniques, although a nucleolus may be seen in wet smears and histologic sections. The cytoplasm forms a narrow rim in small lymphocytes, but it may be abundant in larger cells. The cytoplasm is moderately basophilic and usually is devoid of granules, but larger cells may contain several bright reddish-violet (azurophilic) granules that differ from the granules of myeloid cells because they are not positive in oxidase or peroxidase reactions. By phase contrast microscopy, a well-defined centrosome can be observed adjacent to the nucleus and because it is somewhat rigid, it may cause an indentation in the nucleus. Transitional forms between lymphocytes and plasma cells are often seen in the blood of patients with viral infections (Fig. 11.1C-E). These cells are variously known as lymphocytoid plasma cells or plasmacytoid lymphocytes and represent morphologic stages of differentiation of antigenically stimulated lymphocytes.
Flow Cytometry
Flow cytometry, invented in the 1960s, has been used for diagnosis, staging, and evaluation of patients with hematologic malignancies and has contributed to identification and characterization
of cell populations. With the advent of one- and two-color flow cytometers, two major lymphocyte lineages were identified, comprising the humoral arm (B cell) and the cellular arm (T cell) of immunity. The characterization of the cells on the basis of their phenotype provided a more reliable platform than morphology for accurate diagnosis and evaluation of residual disease. The new technology, using 12- to 18-color analysis of the patient’s blood or other body fluids, brought an unprecedented resolution, which identified multiple subpopulations of T cells, i.e., follicular helper T cells, Th-17 cells and regulatory T cells. Flow cytometry helped our understanding of certain functions of the immune system such as cell cycle analysis, immune deficiencies, the nature of leukemia/lymphoma, and detection of apoptosis induced by various concentrations of gamma secretase inhibitor, N-(3,5-Difluorophenacetyl)-1-Alanyl-Sphenylglycine T-butyl ester (DAPT), and signaling by Notch 1, recognized as the cell fate signaling molecule.
of cell populations. With the advent of one- and two-color flow cytometers, two major lymphocyte lineages were identified, comprising the humoral arm (B cell) and the cellular arm (T cell) of immunity. The characterization of the cells on the basis of their phenotype provided a more reliable platform than morphology for accurate diagnosis and evaluation of residual disease. The new technology, using 12- to 18-color analysis of the patient’s blood or other body fluids, brought an unprecedented resolution, which identified multiple subpopulations of T cells, i.e., follicular helper T cells, Th-17 cells and regulatory T cells. Flow cytometry helped our understanding of certain functions of the immune system such as cell cycle analysis, immune deficiencies, the nature of leukemia/lymphoma, and detection of apoptosis induced by various concentrations of gamma secretase inhibitor, N-(3,5-Difluorophenacetyl)-1-Alanyl-Sphenylglycine T-butyl ester (DAPT), and signaling by Notch 1, recognized as the cell fate signaling molecule.
Electron Microscopy
The small lymphocyte is 6 to 9 µm in size, with a smooth bilaminar cytoplasmic membrane that contains only a few microvilli, except in the area of the uropod in motile cells (Fig. 11.2).19 The scanty cytoplasm of small lymphocytes shows a remarkable absence of organelles. The Golgi apparatus is small, usually found near the nuclear notch, and one or two centrioles are often seen. No organized endoplasmic reticulum is observed, although careful scrutiny may reveal one or two profiles. Many ribosomes are evident, occasionally in clusters, and typical mitochondria are common, but lysosomes containing enzymes characteristic of these organelles are sparse. Dense bodies of unknown significance may also be seen occasionally. The cytoskeleton consists of occasional microtubules in the cytoplasm and microfilaments adjacent to the cell membrane. The nucleus is enveloped by a membrane consisting of two layers which fuse at the site of nuclear pores, while abundant dense heterochromatin forms aggregates close to the membrane, but less often in the center of the body of the nucleus. These aggregates are separated by interchromatinic spaces which contain smaller bits of chromatin aggregates, particles resembling ribosomes, fibrils, and usually a nucleolus.
The medium lymphocyte is larger (6 to 8 µm) because of an increase in the amount of cytoplasm and a Golgi apparatus which is more developed than in the small lymphocyte. The cytoplasm contains numerous polyribosomes and a few strands of endoplasmic reticulum parallel to the nuclear membrane. Lymphoblasts are usually larger cells (8 to 12 µm) with scarce cisternae of endoplasmic reticulum, a nucleus with loose chromatin, and a giant nucleolus that has a reticulated appearance and occupies as much as one third of the nuclear area. Lymphocytes carry the normal diploid number of 44 autosomes and two sex chromosomes.
Scanning Electron Microscopy
By scanning electron microscopy, the human peripheral blood lymphocytes can be seen to be of two broad categories on the basis of their cell surface 20; one population has a fairly smooth surface, whereas the other is covered by numerous microvilli (described as “hairy”) (Fig. 11.3). The smooth cells correspond to thymusderived, or T lymphocytes, whereas the one with the microvilli is bone marrow-derived or B lymphocyte. The surface features of the lymphocyte depend on the functional state of the cells as well as the methods used for preparation of the cells.21 All lymphocytes develop villi if they are stimulated by mitogens or after crossing the high endothelial cells of LN venules, but recover their smooth surface when they reach their respective home microenvironment. Therefore the smooth cell surface is likely associated with resting lymphocytes, whereas microvilli appear following environmental stimuli that interact with cell-surface receptors and may help lymphocytes to interact with target substrates.
B cells with characteristic thin cytoplasmatic projections, i.e., hairy, are positive for tartrate-resistant acid phosphatase (TRAP), and by immunophenotyping they are shown to be positive for CD-103, CD-25, and CD-11c.
Malignancy of this B cell subpopulation has a typical clonal pattern and is known as hairy cell leukemia (HCL), an indolent B cell lymphoproliferative disease, characterized by splenomegaly and pancytopenia.22
Lymphocyte Locomotion
The motile lymphocyte may extend cytoplasmic projections, 12.5 to 200 nm wide and up to 0.8 µm in length, and moves with an average speed of 20 µm/minute. Its motility increases with the state of activation; thus lymphoblasts demonstrate greater locomotion compared to small lymphocytes. Locomotion is enhanced by stimuli inducing blast transformation, and the trailing edge of the mobile lymphocyte, the “uropod,” is associated not only with locomotion, but with a variety of interactions with lymphocytes, macrophages, and other cells.23 (Fig. 11.4)
The T lymphocytes adhere to macrophages, forming clusters or rosettes, and their mobility is regulated by their lamellipodia and filopodia.24 A stable cluster forms only when macrophages and lymphocytes are exposed to antigen and the T lymphocytes within the clusters are stimulated into proliferative activity. In general, the uropod formation is a morphologic expression of lymphocyte activation as measured by DNA synthesis. The locomotion of lymphocytes is strikingly different from that of other cells, since they move forward in a steady manner, maintaining a hand mirror shape, whereas myeloblasts express a wriggling worm-like locomotion, and the cells of the monocytic series change shape and direction continuously. Locomotion of the lymphocytes is an important function regulated by the Krupel-like transcription factors which regulate other important functions, such as trafficking and differentiation.25 Although the uropod is characteristic of T lymphocytes, the B lymphocytes also form uropods after stimulation with anti-immunoglobulin antibodies26 and the formation by surface immunoglobulin (Ig) of caps, which eventually are endocytosed. The uropod therefore serves not only in cell mobility but also as a site for endocytosis of foreign substances, and ultrastructurally contains practically all cytoplasmic organelles, including the Golgi apparatus, mitochondria, microfilaments, and microtubules. It has been shown that an important protein in T cell motility, known as Kidins-220, is expressed in high concentration in the T cell uropod associated with intercellular adhesion molecule-3 (ICAM-3).27 The enzyme kinase-D, which interacts with Kidin-220, regulates the motility and migration of the T cell, essential for its response to foreign antigens.
PLASMA CELLS
Plasma cells are the progeny of lymphocytes and morphologically are easily distinguished from other cell types (Fig. 11.5) by their spherical or ellipsoid shape and their size, which ranges from 5 to 30 µm. The cytoplasm is abundant and basophilic, usually deep blue, and may have a granular character. There is a well-defined perinuclear clear zone that contains the Golgi apparatus. The nucleus is small in relation to the cell size, round or oval, eccentrically placed, and contains dense masses of chromatin.
By electron microscopy, the plasma cell membrane and the nucleus appear to be similar to those of the lymphocyte, but their cytoplasm is characterized by a well-developed rough endoplasmic reticulum (Fig. 11.6A) that fills most of the cytoplasmic space, except for a clear area in the perinuclear zone which
contains the Golgi apparatus. The endoplasmic reticulum consists of lamellae arranged in a variety of patterns but usually as parallel convolutions. Their inner surfaces are smooth and form the walls of spaces (cisternae) that are filled with amorphous products of varying density. The outer aspects of the lamellae are rough because of attached ribosomes, and a few mitochondria may be seen.
contains the Golgi apparatus. The endoplasmic reticulum consists of lamellae arranged in a variety of patterns but usually as parallel convolutions. Their inner surfaces are smooth and form the walls of spaces (cisternae) that are filled with amorphous products of varying density. The outer aspects of the lamellae are rough because of attached ribosomes, and a few mitochondria may be seen.
The morphology of plasma cells shown by electron microscopy as described above is the most typical, but intermediate forms exist which resemble small lymphocytes,14 with a scant amount of cytoplasm and an unusually well-developed rough endoplasmic reticulum (Fig. 11.6B). Such intermediate cells (Fig. 11.1C-E) are common in the blood of patients with plasma cell dyscrasias or immunologic diseases characterized by hypergammaglobulinemia. Similar cells have been observed in the blood of patients with viral infections (Turk cells),19 including infectious mononucleosis, as well as in the blood of apparently healthy individuals. Alternatively, immature plasma cells may have an appearance more akin to that of phytohemagglutinin (PHA)-transformed lymphocytes, with large and leptochromatic nuclei and cytoplasm containing a simple endoplasmic reticulum, but many ribosomes and polyribosomes, demonstrating that it is often difficult to draw sharp cytologic dividing lines. Other plasma cells may contain vacuoles or needle-type inclusions in the cytoplasm (Fig. 11.5C).
PRIMARY LYMPHOID ORGANS
The cells of the immune system originate in the primary lymphoid organs, which in humans are the bone marrow and the thymus, and during their life span settle in the secondary lymphoid organs, i.e., spleen, LNs, and Peyer patches (PPs) of the gut and the Waldeyer ring (tonsils and adenoids). This division provides the anatomic basis for the two fundamental stages of lymphocyte differentiation, i.e., the antigen-independent stage in primary lymphoid organs and the antigen-dependent stage in the secondary.
The primary lymphoid organs develop first in ontogeny, while the secondary organs provide the proper microenvironment for antigen-dependent differentiation of lymphocytes from immature precursors. Immunocompetent lymphocytes are released from the primary organs and home to specific areas of the secondary lymphoid organs.
The secondary lymphoid organs provide an optimal microenvironment for attracting antigen-specific lymphocytes directing the terminal stages of lymphocyte differentiation activated by antigens.
Bone Marrow
A detailed account of the histologic features and function of bone marrow is given in Chapter 5. Here we concentrate on the contribution of bone marrow in lymphopoiesis as a “primary lymphoid organ.” The bone marrow occupies the medullary cavities of bones throughout the skeleton. It is the major hematopoietic organ in humans and supports differentiation of all blood cells,28 although in some cases this differentiation is not always complete. T lymphocytes and monocytes, for example, reach their final stages of maturation in locations outside the bone marrow.
The bone marrow is divided into an extravascular compartment, which is the site of hematopoiesis, and a vascular compartment, composed of wide venous blood vessels known as sinuses. These vessels receive blood from the nutrient artery and the periosteal capillary network. The sinuses are radially disposed in the bone marrow and eventually open into larger, centrally located sinuses that exit through the same foramina used by the nutrient arteries. The walls of the venous sinuses consist of an endothelial layer, a basement membrane, and the adventitia. The endothelial cells are flat, with tapering ends, and contain the usual organelles and therefore are endowed with endocytic activity.
The adventitial cells have broad sheet-like processes that form a reticulum, the interstices of which are occupied by the hematopoietic cells. Under certain circumstances, the adventitial cells become swollen because of an increased fat content, and the gross appearance of the marrow turns from red to yellow. In conditions of increased demand on the hematopoietic process, the fatty adventitial cells decrease in size, allowing an expansion of the hematopoietic compartment. The adventitial cells are related to the reticular cells found in the splenic cords and are therefore called adventitial reticular cells.
The hematopoietic compartment displays specific cellular arrangements of hematopoietic cells. Megakaryocytes lie close to the adventitial cells and deliver platelets directly into the sinuses through apertures in the sinus walls. Erythrocytes are produced near the sinuses, forming erythroblastic islets, while granulocytopoiesis takes place at a distance from the sinus wall in a diffuse pattern or sometimes in distinct clusters or sheets.
Available evidence obtained through labeling techniques suggests that new lymphocytes are formed at the periphery of the bone marrow and move toward the center in a centripetal fashion.
The lymphocyte progenitors are enriched with a prolymphocyte population which contains progenitors for B cells and natural killer (NK) cells, which are CD122+ and under defined culture conditions can mature toward B or NK lineage. The B cells are generated from hematopoietic stem cells (HSCs), which migrate from the subendosteal region toward the center of the cavity.
These stem cells differentiate toward several cell lines as a result of signals received from bone marrow-adherent cells that create microenvironments known as niches that support hematopoiesis. The bone marrow stromal cells can be identified by their phenotype, i.e., CD105, CD90, CD184, and class MHC II.29 HSCs interact with the osteoblasts of the bone, which may contribute to the creation of the hematopoietic niche30 and support the development of hematopoietic colonies as a result of secretion of a variety of cytokines.31
The development of an in vitro culture system32 facilitated the identification of the growth and differentiation factors necessary for B cell lymphopoiesis, while animal studies defined five stages of B cell differentiation downstream from the common lymphocyte progenitor (CLP) cell: (a) pre-pro-B cell, (b) pro-B cell, (c) pre-B cell, (d) immature B cell and (e) mature B cell. The CLP is the first cell which is irreversibly committed to B or T cell differentiation, but the terminal stages of B cell differentiation, within bone marrow niches, require the availability of certain growth and differentiation factors such as Ikaros,33 which acts as a factor of lineage competency and facilitates both myeloid and lymphoid development programs.34
Immediately after commitment to the B lineage, precursors become dependent on SDF1 chemokine and its receptor CXCR4, as shown with the earliest identifiable B cell precursor populations in the bone marrow, where their development in CXCL12 (SDF1)−/−, but not in IL7−/−, embryos is severely disrupted.35
The SDF-1 and its receptor are the first example of a chemokine and its receptor responsible for specific biologic activities of the B cell precursors in the earliest B lineage stages.35 Other important molecules for the function of B cells are the early B cell factor (EBF) and the FLT3 ligand, essential at the pre-pro-B and pro-B stages and the chemokine ligand CXCL12 (known as stromal cell-derived factor-1, SDF1).36
The B cell growth after pro-B cell is regulated by interleukin-7 (IL7) (which is essential for the development of B cells.37 The chemokine CXCL12, which binds to its receptor CXCR4 of stromal cell, guides and retains B cells to appropriate secondary lymphoid organs.38 Newly synthesized lymphocytes are discharged into the circulation and have been found singly or in small groups near the sinusoidal walls. Some of them are in transit through the wall of the sinus, which suggests that B lymphocytes are not stored in the bone marrow except for brief periods of time before they are released into the circulation. Histologically, no lymphoid follicles are distinguishable in normal bone marrow, which resembles the red pulp of the spleen with vascular sinuses and reticular meshwork formed by the adventitial cells or the cordal reticular cells, respectively. The bone marrow circulation, however, is closed while the circulation of the red pulp is partially open, and there is no endothelial continuity between the arteries and the veins.
Thymus
Ontogeny and Histology
The thymus is a lymphoepithelial organ situated in the superior mediastinum39,40 which consists of two lobes that are divided into lobules forming the basic anatomic units of the thymus. The thymus is covered by a fibrous capsule from which fibrous bands (trabeculae) penetrate the parenchyma, dividing it into lobules. Histologically, the lobules have two distinct regions (Fig. 11.7), (1) a peripheral region named the cortex which is divided into the outermost or subcapsular cortex, and the inner or deep cortex, and (2) a central region named the medulla. In hematoxylin-eosin-stained sections, the cortex appears dark blue to purple because of the predominance of lymphocytes (80% to 85%), whereas the medulla appears eosinophilic because of the predominance of the epithelial cells. These anatomic divisions correspond to functionally distinct microenvironments that support specific phases of thymocyte differentiation. The subcapsular or superficial cortex contains large, actively dividing blasts with many mitotic figures, while the deep cortex is composed principally of nondividing small thymocytes.
The medulla contains predominantly medium-sized thymocytes. The relationships between the thymocytes in each compartment and the mechanisms underlying thymocyte differentiation are discussed in detail in Chapter 13.
The embryologic origin of the thymus has been under dispute for more than 30 years41. According to one view, the thymic primordium arises from the third pharyngeal pouch (endoderm) as well as the pharyngeal crest (ectoderm) 42; while another study supported exclusively an endodermal origin,43 which alone contributes to the formation of the thymus.44
The pharyngeal pouches are transient embryonic structures and their formation requires the paired box gene 1 (PAX 1) and 9 (PAX 9) as well as the fibroblastic growth factor-8 (FGF8). They develop in an anterior to posterior sequence and are separated from each other by pharyngeal arches. Each endodermal primordium contains precursors for one thymic lobe and one parathyroid gland. It is surrounded by a capsule of mesenchymal cells derived from cells of the neural crest, which supports the growth
and development of the primordium, and under their guidance it separates into a thymus and pharynx.
and development of the primordium, and under their guidance it separates into a thymus and pharynx.
![]() FIGURE 11.7. Histology of thymus shows lobules (L) consisting of cortex (C) and medulla (M). H, Hassall corpuscles; S, septa (trabeculae). |
The development of the thymus proceeds through several stages: (a) positioning, i.e., determination of the place where the organ rudiment will develop; (b) growth and patterning, i.e., generation of distinct regions in the growing rudiment; and (c) differentiation, i.e., separation of distinct cell types that have been developed. The function of the homeobox A3 (HOXA3) is necessary to identify the third pouch, which forms the thymus and may control the position of the mitral rudiment.44 Mice lacking Hoxa3 are athymic and have no parathyroids, since the third pouch endoderm cannot differentiate appropriately. The expression of PAX1 in all pharyngeal pouches requires two other genes such as the eyes absent gene (EYA1) and the sine oculus-related homeobox1 homologue (SIX1).45 Functional evidence that the endoderm alone forms a fully functional thymus was obtained through ectopic transplantation of isolated endoderm tissue grafted under the kidney capsule.46 Normally, the structures of the original tubular epithelium, one on each side, descend into the anterior superior mediastinum where they fuse, forming the mature organ with two lobes. At this stage, the thymus is triangular, with the base resting on the pericardium and the apex pointing toward the neck.
Once the anlage is formed, the function of the WHN (winged helix nude; now known as FOXN1) gene is required for the completion of thymic structure and function.47,48 The nude locus gene encodes the FOXN1 transcription factor, of the forked/winged/helix family. It contains a DNA-binding domain and a strongly acidic transcriptional activation domain, which is functionally indispensable. Mutations affect the DNA-binding domain or the C-terminal transcriptional activation domain and are associated with athymia and hairlessness because the gene regulates the keratinization of hair.
The neural crest contributes to the formation of several glands, including the perivascular mesenchyme of the thymus.49 The neural crest cells migrate into the thymus early in development, surrounding the epithelial rudiments and are closely associated with the developing thymocytes. They form the connective tissue capsule and trabecular septa that penetrate the parenchyma, dividing it into lobules, and likely provide extracellular matrix into the cortex and medulla, such as hyaluronan, collagen, and fibronectin, which may be important for the presentation of growth factors to the thymocytes. Hyaluronan is the ligand of CD44, and their interactions are important in the early stages of human thymus development.50 The undifferentiated epithelial cells are large, with dispersed chromatin, short, blunt cytoplasmic processes, and sparse tonofilaments. As the cell matures, the amount of cytoplasm decreases and long dendritic processes form, which permit tight junctions between adjacent cells and the formation of the epithelial framework, within the interstices of which T-lymphocyte differentiation takes place.
Migrating lymphoid progenitors initiate a symbiotic relationship with the epithelial cells and contribute to the organization of the thymic microenvironments. Whereas prothymocytes (i.e., immature T cells) regulate induction of the cortical microenvironment,51 the mature thymocytes, i.e., T cell receptors (TCR)+ organize thymic medullary epithelial cells.52 E-cadherin plays an important role at this stage because it mediates interactions that shape the cortical and medullary thymic microenvironments which are architecturally distinct.53
Heterotypic interactions important for thymocyte proliferation occur between epithelial cells, which express the E-cadherin, and the double-negative T lymphocytes, which express the ligand of E-cadherin, i.e., CD103 or αEβ7 integrin.54
The thymic anlage is permeated by blood vessels, which also contribute to a well-organized medullary epithelial compartment, even in the absence of CD3+ mature thymocytes (i.e., in Rag2−/−mice). Medullary epithelial cells arranged as cuffs around vessels of intermediate size affect the development of the thymic microenvironment.55
Addition of keratinocyte growth factor, a member of the fibroblast growth factor family secreted from mesenchymal cells, results in the expansion of the medullary epithelial compartment in the Rag2−/−mice.56 These data emphasize the fact that the growth and maintenance of a functional thymic microenvironment is a highly complex process and is maintained by stimuli from all structural components. The RELB gene encodes a subunit of the nuclear factor-κB complex, critical for the coordination of several aspects in the development and organization of medullary epithelial cells and dendritic cells.
Overexpression of CD40L on thymocytes disrupts the thymic architecture and the epithelial differentiation, with loss of cortical epithelial cells, expansion of the medullary compartment, and infiltration of the capsule with a mixture of CD3+ cells, B cells, and macrophages.57 These findings point out that regulation of normal development of the thymus is a highly complex process, delicately regulated by multiple cellular interactions and soluble factors released as a result of these interactions.
The medullary epithelium expresses several molecules, considered tissue specific such as parathyroid hormone, thyroglobulin, insulin, and respiratory epithelium. This may represent a “promiscuous” gene expression or a “mosaic” of epithelial differentiation,58 which may be the basis for the establishment of self-tolerance. This view is supported by the demonstration that the AIRE gene, which is defective in autoimmune polyendocrinopathy candidiasis ectodermal dystrophy (APECED), is expressed by medullary thymic epithelial cells (TECs).59
AIRE (auto immune regulator) is a DNA-binding nuclear protein with a putative DNA-binding domain named SAND (Sp100, Aire-1, NucP41/75, DEAF-1) and four LXXLL nuclear receptor-binding motifs. The structure of AIRE suggests that its function is that of a transcription regulator60,61 which interacts with CREB-binding protein (CBP), a property that mediates its transcriptional regulation property.62 It also contains a proline-rich region, known to be expressed by several transcription factors which mediate interactions with SH3 domains.
The LXXLL motif binds coactivators to nuclear receptors and activates transcription of target genes by nuclear receptors. AIRE plays a vital role in preventing autoimmunity; and mutations in this molecule cause a recessive inherited disease known as APECED or autoimmune polyendocrinopathy syndrome type-1 (APS-1). APECED at the beginning is presented as mucocutaneous candidiasis early in childhood with eventual destruction of endocrine organs and the appearance of Addison disease, hypoparathyroidism, and type I diabetes.63 AIRE is expressed in medullary
epithelial cells, testes, and cells of dendritic lineage.64 It has been suggested that AIRE may induce transcription of genes specific to other tissues that are promiscuously expressed in the TECs.65
epithelial cells, testes, and cells of dendritic lineage.64 It has been suggested that AIRE may induce transcription of genes specific to other tissues that are promiscuously expressed in the TECs.65
It promotes the expression by TECs of self antigens from other tissues facilitating the removal of thymocytes with self-reactive T cell receptors.66,67
Recently, a second thymus has been identified in the neck of mice with typical cortical and medullary structures.68 The cervical thymus is structurally the same as the thoracic thymus, consisting of cortex and medulla and containing double-positive (CD4+/CD8+) and single-positive (CD4+/CD8− and CD4−/CD8+) thymocytes expressing TCR/CD3 complexes. The cervical thymus is fully functional, because it could supply T cells to athymic (nude) mice, correcting their immune deficiency.
This unexpected discovery poses a number of questions for experimental work done with thymectomized animals over the past 45 years.69 Perhaps the cervical thymus is too small to be relevant and its cellularity is only 1/500th of the thoracic thymus.70
Work done with thymectomized animals suggest that the role of the thymus in autoimmune disease may have to be reconsidered.71
Nonlymphoid Cells
Thymic Epithelial Cells
The thymic rudiment is populated by epithelial cells which are distinguished anatomically as cortical and medullary. Lack of Hoxa3 has an impact on the early phases of thymic organogenesis from the third pharyngeal pouch.72 Foxn1 is expressed in epithelial progenitors of cortical and medullary compartments and regulates the differentiation of TECs during embryonic and adult life. In Pax9 null mice, the thymic anlage develops as an ectopic polyp-like structure, and expression of the Foxn1, a marker of thymic epithelium, is markedly reduced. Expression, however, of the Tcrb genes is readily detectable in the mutant thymus, but the TCR gamma chain is not detectable.73 The forkhead transcription factor Foxn1 is indispensable for TEC development and function, acting as a master regulator of the epithelial programs of the thymus.74
Overexpression of Foxn1 attenuates the decline of thymocyte numbers and frequency of early thymic progenitors, and histologic studies reveal that structural alterations associated with thymic involution are diminished in aged Foxn1 transgenic mice.75
Deletion of Pax1 results in thymic hypoplasia as a result of defective organogenesis of the thymus, while Pax9 causes marked thymic hypoplasia because of defective cellular migration to thymic rudiments. In Pax9 null mice the thymic anlage develops as an ectopic polyp-like structure in the larynx, expresses Whn/Foxn1, the markers of thymic epithelium, but fails to perform the normal caudo-ventral movement to the upper mediastinum, and the TCR-γ chain is not detectable.73 Loss of Foxn1 function, on the other hand, results in a more complex phenotype known collectively as the “nude” mouse.
The “nude” mouse has been an important “experiment” of nature that has provided important insights of the function of the thymus.
Abnormal thymic development is associated with defective keratinization, as seen in the nude mouse,76 and although the early stages of development proceed normally, including normal mediastinal migration, the subsequent rudiment of the thymus does not develop as a result of failure of its colonization by lymphoid progenitors.77
It is generally accepted that organogenesis of the thymus is completed in two distinct steps. During the first stage, endodermal elements of the third pharyngeal pouch migrate to the mediastinum and form the thymic rudiment. In the second stage the Foxn1 transcription factor drives differentiation of multipotential progenitors to the thymic cell lineage. In the absence of Foxn1 function, the cells of the rudiment resemble respiratory epithelium in their phenotype and organizational features.74 As shown in nude mice, T cell development and selection require expression of the forkhead transcription factor Foxn1 regulated by Wnt glycoproteins secreted by epithelial cells and thymocytes. In the absence of their function, epithelial cell morphogenesis is defective and lymphoid precursors are not attracted to the thymic primordium. The Wnt molecules therefore provide regulatory signals critical for thymic function.78
The glycoproteins Wnt bind to glycosaminoglycans of the extracellular matrix, cell-surface receptors of the Frizzled (Fz) family, and members of receptors related to low density-lipoprotein receptors. Through regulation of Foxn1 function the Wnt proteins contribute importantly in the development of TECs.
The epithelial cells in the cortex and medulla have a common progenitor as shown by clonal analysis,79 and these progenitors still exist in the thymus after birth.80
Ultrastructurally, the epithelial cells are highly heterogeneous, probably reflecting their divergent origins (see Chapter 13). In the past, the epithelial cells have been referred to by a variety of designations, such as reticular, syncytial, epithelioid, and squamoid (Fig. 11.8). The characteristic features that distinguish them from other cells in the thymic parenchyma are the presence of tonofilaments and desmosomes. The tonofilaments are filamentous structures approximately 3.0 nm in diameter and 0.1 to 3.0 µm in length which often form bundles and are located close to the nucleus. Desmosomes are detected at the junctions of the dendritic processes of adjacent epithelial cells. Their oval nuclei have evenly distributed chromatin and prominent nucleoli. The epithelial cells joined by desmosomes form a continuous meshwork that surrounds the trabeculae, the blood vessels and the inner surface of the capsule. Between the epithelial sheath and the supporting scaffolding of the trabeculae and blood vessels there forms a kind of a continuous basement membrane or meshwork, which is distinct from the basement membrane of the capillaries and separates the epithelial meshwork from the cavernous spaces filled with thymocytes.
The cortical epithelial cells do not have secretory granules and probably do not participate in the secretion of thymic hormones. Such interactions are regulated by products of genes of the major histocompatibility complex. Indeed, the presence on epithelial cells of class I and II molecules in mice and humans has been demonstrated.
The distribution of class II antigens is typically dendritic in the cortex, but is confluent in the medulla. MHC II levels, as well as the percentages and cycling status of TEC populations expressing MHC II, were not static during postnatal development, suggesting
quantitative flexibility in presenting signals to the developing thymocytes.81
quantitative flexibility in presenting signals to the developing thymocytes.81
The class II antigens are of higher density than the class I antigens in cortical epithelial cells, but they are of the same high density in the medullary epithelium. Using immunohistochemical techniques and electron microscopy, investigators have shown that these molecules are of epithelial origin because they are located on cells containing desmosomes and tonofilaments.
The thymocyte-epithelial cell interaction in the outer cortex results in the formation of lymphoepithelial complexes known as nurse cells (TNC) (Fig. 11.9). TNCs are located within the corticomedullary junction to express cytokeratins five and eight (K5 and K8) and the transcription factor Trp-63. The phenotype suggests that the TNCs play an important role in thymocyte selection and may also be involved in the maintenance of thymic epithelia.82 They are composed of large clusters of lymphocytes surrounded by cell membrane and appear to reside within the cell body of an epithelial cell. Their significance in T cell differentiation is discussed in Chapter 13.
The epithelial cells of the medulla do not have long dendritic processes and are known as epithelioid or spatulate epithelial cells. They are more pleomorphic and contain dark granular inclusions that may have secretory functions. Another variety of epithelial cells, known as squamoid, is found exclusively in the medulla. These cells probably give rise to Hassall corpuscles and contain dense bundles of tonofilaments and masses of keratohyalin.
The Hassall corpuscles, first described more than 150 years ago,83 are solid or cystic, and their origin is highly disputed. Hammar suggested that Hassall corpuscles arise as solid structures through the tight apposition of as many as 20 to 50 epithelial cells in a concentric fashion, but degenerative changes in the centrally located cells produce the cystic forms. The cavity of the cystic forms of these corpuscles is lined by epithelial cells with villous projections, and sometimes it is filled with debris and degenerated cells. In some solid corpuscles, keratinization is prominent and is similar to that of the skin.
Monoclonal antibodies specific for high-molecular-weight keratins that react with terminally differentiated epithelial cells of the skin also bind to Hassall corpuscles.39,84 Epithelial cells of the deep cortex are phenotypically different from those in the medulla, which undergo terminal keratinocyte differentiation. Hassall corpuscles are not the epithelial cell graveyard as previously thought, but contribute actively to important immunoregulatory functions. They secrete thymic stromal lymphopoietin (TSLP), which regulates CD4+ T cell homeostasis through activation of thymic dendritic cells (TDCs) (see below) and induces proliferation and differentiation of CD4+/CD25+/Foxp3+ regulatory T cells from CD4+/CD25− thymocytes.85
Macrophages
Macrophages are found throughout the thymus: among thymocytes, in capsular and septal connective tissues, and in the perivascular space. Some macrophages are surrounded by thymocytes and form rosette-like structures. Thymic macrophages direct thymocyte maturation, but appear to be involved also in the disposal of many thymocytes dying within the thymus. Macrophages are more prominent in involuted thymuses after stress or steroid hormone treatment, rendering the histologic picture of a starry sky.
Lymphocyte phagocytosis is found in the germinal center (GC), a site that also is characterized by high proliferative activity.
Interdigitating Dendritic Cells
A third population of nonlymphoid cells in the corticomedullary junction is known as interdigitating dendritic cells or thymic dendritic cells (TDCs). They contain an irregularly shaped nucleus and clear cytoplasm, but they lack the Birbeck granules characteristic of Langerhans cells (LCs) and their phenotype and origin apparently varies.
Specialized dendritic cells (DCs) within the thymus are crucial for deletion of autoreactive T cells. The question of whether these cells arise from intrathymic precursors with T cell potential has been hotly debated, and the regulatory pathways as well as the signals that direct their development remain unclear.86 Some are CD8a+-derived from a common precursor with T cells and therefore are lymphoid-related and the granulocyte-macrophage colony-stimulating factor is not required for their development (in contrast to the myeloid DCs), and they need functional Ikaros transcription factors for their maturation.87 In humans, pluripotent stem cells, which leave the bone marrow to migrate to the thymus, have the potential to develop into T cells and DCs.88 The question whether these cells arise from intrathymic precursors with T cell potential has been hotly debated, and the regulatory pathways and signals that direct their development remain unclear.89
Other thymic DCs express the CD116/CD8 phenotype (i.e., the Langerhans cells in other tissues) and originate from DC precursors.90 Their differentiation is driven by factors produced by TECs as well as Hassall corpuscles.91 Another pathway of TDC differentiation is from double-negative thymocytes (i.e., CD4−/CD8−) stimulated, in the presence of IL7, by IL18 produced by TECs.92
Thymic stromal cells produce the IL7-like lymphopoietin, TSLP, that acts through appropriate receptors on dendritic cells carrying self-peptide-MHC complexes inducing expansion of CD4+ T cells. TSLP is also produced by epithelial cells in tonsillar crypts and other mucosal tissues, and thus may exert a homeostatic mechanism on CD4+ T cells, through dendritic cell activation not only within the thymus, but in other mucosal lymphoid tissues as well.
Myoid Cells
Myoid cells are striated muscle cells seen in many lower vertebrates and are found in the medulla of the thymus. Their origin has not been documented but it is believed to originate from mesenchymal or epithelial cells to which they are attached by desmosomes.
They contain muscle proteins, including myosin, desmin, acetylcholine receptors, and numerous intracytoplasmic filaments arranged in haphazard fashion.
Their presence in human fetal thymuses has been documented and plays a role in T cell differentiation, maturation, and protection of thymocytes from apoptosis.93
Lymphoid Cells
Lymphoid cells constitute 80% to 85% of the cortical and 15% of the medullary cells. The outer cortex lymphocytes are large blast cells with dark blue cytoplasm that proliferate actively. They are the immediate descendants of bone marrow-derived prothymocytes, which enter the thymus and migrate to the subcapsular cortex. As they mature, they move into the deeper cortex, which is occupied by small nondividing thymocytes. The medullary thymocytes are of medium size and are considered emigrant cells, although this fact has not been proven conclusively. The recruitment, migration, and differentiation of lymphocytes and the relationship between subcapsular, deep cortical, and medullary thymocytes are discussed in Chapter 13.
Thymic Vasculature
The thymic anlage initially is avascular and is penetrated by vessels at approximately 12 to 14 weeks of gestation. The thymic arteries are branches of the inferior thyroid, internal mammary, and pericardial phrenic arteries; they enter the thymus and pass down to the medulla, branching into arterioles which penetrate into the deep cortex.
Capillaries that arise from the arterioles run toward the subcapsular cortex, where they anastomose and turn inward toward the medulla, eventually forming venules.94 The vessels are ensheathed with epithelial cells and connective tissue that is continuous with the capsule.
Histologically, a number of layers can be distinguished from the lumen of the blood vessels outward which constitute the blood-thymus barrier: (a) endothelium; (b) vascular basement membrane; (c) mesenchymal perivascular connective tissue space occupied by collagen fibers; (d) fibroblasts, macrophages, and other cells; (e) epithelial basement membrane; and (f) epithelial cell syncytium. The mesenchymal interstitial space is probably formed in the early stages of development when the TECs envelop the blood vessels; it is considered extraparenchymal. The tightness of the barrier has been tested with particulate and protein tracers. It is tight in the cortex, mainly because of the impermeability of the endothelial junctions. Traces of protein transported by plasmalemmal vesicles of the endothelial cells which are released on the parenchymal front are removed rapidly by macrophages along the interstitial spaces of the vessels and are prevented from coming into contact with the thymocytes. This barrier, however, is incomplete in the medulla, especially along the site of thymocyte migration through the wall of venules.95 The fact that antigens are detected in the thymus indicates that the “barrier” has leaks, although experimental tracers have never reached the cortical parenchyma. This arrangement of the vasculature and the epithelial sheaths separates the thymus into the intraparenchymal compartment, composed of the lymphoepithelial complex and the extraparenchymal compartment composed of the blood vessels and the surrounding interstitial space.
Involution
Beginning at puberty, the thymus undergoes a gradual process of involution, characterized by loss of the cortical lymphocytes and atrophy of epithelial cells and their replacement by fat originating from mesenchymal cells present in the connective tissues along the vasculature and capsule. More than 50% of the thymus is replaced by adipose tissue by the age of 40 to 45 years, but the fat is still contained in the extraparenchymal compartment, separated from the remaining lymphoepithelial complex by the epithelial basement membrane and the sheet of epithelial cells. The age-related involution may be influenced by stress and other factors.
Thymic involution starting at puberty has been associated with use of sex hormones. This hypothesis, however, has been challenged, since morphometric studies have shown that thymic involution actually starts early in life,96 as the potential of intrathymic progenitors to maintain the level of mature thymocytes starts to decrease at that time.97
With a rise of sex hormone concentration there is a decline in the production of growth hormone and many of its effects in the periphery are mediated by insulin growth factor-I induction.98 A slow rate of thymic activity is maintained throughout life with complete but slow renewal of T cells,99 but the changes of the thymic tissue are quantitative rather than qualitative. At the molecular level thymic involution is linked with the expression of JAGGED-1 protein in T cells100 together with Delta protein. The gene of this protein is a member of the Delta/Serrate/Lag2 (DSL) genes which regulate Notch signaling implicated in regulation of T cell activation and differentiation. Expression of JAGGED-1 on T cells interferes with Notch signaling and induces premature involution as a result of apoptosis of TECs. In contrast to chronic involution which is a result of aging, the thymus undergoes acute involution as a result of stress, which is mediated by adrenal corticosteroids. Injections of glucocorticoids eliminate as much as 75% of the thymocytes within 2 to 3 days, affecting both the cortex and the medulla, but the effects are more pronounced in the cortex. Most of the cortical thymocytes are cortisone sensitive, whereas the medullary thymocytes are cortisone resistant. As a result of acute stress the lymphocytes undergo pyknotic changes, fragmentation of the nuclei, and eventually a decrease in size; subsequently they are phagocytosed by macrophages.
Recovery takes place within 8 to 10 days and is marked by an early increase in mitotic activity in the subcapsular cortex.
Pathology of Human Thymus
Myasthenia Gravis
In some of the patients with myasthenia gravis, the thymus shows an increase in the number of medullary epithelial cells containing thymic hormones and development of follicles with GCs composed of B cells and many plasma cells,101 i.e., histologic changes which are typical of reactive peripheral lymphoid organs. These follicles are located outside the epithelial basement membrane, predominantly in the medulla, and are surrounded by many Hassall corpuscles; but the cause of these histopathologic lesions is unknown. Antibodies against the acetylcholine receptor, in the neuromuscular junction, play an important role in the pathogenesis of myasthenia gravis; and it is intriguing that this receptor, or a structurally similar antigen, has been detected on thymic cells.102 Patients with myasthenia gravis have circulating antibodiesreacting in culture with the striations of myoid cells from animal and human thymuses, as well as muscle cells expressing acetylcholine receptors.103 These are the “myasthenogenic” factors in the blood of the patients, which in mice induce many characteristic features of the disease, i.e., reduced amplitudes of the miniature endplate potentials and a reduction of the number of acetylcholine receptors available for binding of bungarotoxin
up to 38% to 54%. Approximately 10% to 15% of patients with myasthenia gravis have thymomas, but these histologic changes are not characteristic of myasthenia gravis, since they have been observed in patients with other diseases such as endocrinopathies, i.e., Addison disease and thyrotoxicosis; and autoimmune diseases, such as systemic lupus erythematosus.
up to 38% to 54%. Approximately 10% to 15% of patients with myasthenia gravis have thymomas, but these histologic changes are not characteristic of myasthenia gravis, since they have been observed in patients with other diseases such as endocrinopathies, i.e., Addison disease and thyrotoxicosis; and autoimmune diseases, such as systemic lupus erythematosus.
Similar lesions have been identified in thymuses from patients with multiple sclerosis, supporting a well-documented fact that the thymus and the brain share some antigens such as the Thy-1 antigen (CD90).
Dysplasia. The thymus of patients with severe combined immunodeficiency disease is characterized by scant lymphocytes with reduced numbers of epithelial cells.
Thymomas. Tumors of the thymus are epithelial, lymphocytic, or consist of a mixture of both cellular elements; while others are mixtures of ductal and glandular structures mixed with epithelial cells. These cases are thought to imitate the early ontogenetic stages of the thymic anlage and support the view of the double origin of the thymic epithelium. Thymic lymphomas that appear as mediastinal masses are associated with acute T cell leukemia in children who bear the phenotype of cortical thymocytes in the early stages of their development. The diagnosis of primary thymic epithelial tumors has been controversial, because of the difficulties in their histopathologic classification and prognostication of their clinical behavior. A new histologic classification has recently been proposed by the World Health Organization (WHO),104 which provides an improved platform over pre-existing classifications for clinical practice in relation to assessment and treatment of the patients.
SECONDARY LYMPHOID ORGANS
Lymph Nodes
Ontogeny
The fundamental concepts of lymphoid organ neogenesis were generated approximately 100 years ago, and the ontogeny of the secondary lymphoid organs, i.e., lymph nodes (LN), Peyer patches (PP), and spleen is highly complex (reviewed in Refs. 105-107). The evolution of their development generated structures, the lymphoid organs of the immune system, to fit their function, i.e., as defenders of the integrity of “self.” This of course requires enhancement of their “sensitivity of antigen recognition” in order to facilitate activation and differentiation of antigen-specific cells, to be followed by the activation and deployment of the defence mechanisms of the body. The LN and PP develop from the budding of a group of cells from large vessels of lymph sacs which are penetrated by connective tissue and lymphatic vessels interconnecting with other vessels forming a network; this is regulated by the PROX1 gene which is expressed on lymphatic endothelial cells and is required for budding and sprouting of the lymphatic endothelium108. The budding occurs in one spot in the anterior cardinal veins of the neck and generates a lymph sac, which gives rise to the lymphatics of the neck, thorax, heart, lungs, and forelegs regulated by the PROX1 gene.109 After the development of the lymphatics, circulating CD45+ CD4+ CD3− hematopoietic progenitor cells from the liver provide the signals for the induction of LNs and PPs. Lymphotoxin (LT) is essential for the formation of LNs and PPs, as shown in mice rendered deficient in LT by gene targeting,110 and in the white pulp of the spleen normal segregation of B and T cells does not occur. Both phenotypes of T cells, i.e., CD4+CD8− and CD4−CD8+ are present in a normal ratio.
Lymphocytes positive for immunoglobulin-M are present in increased numbers in both the spleen and peripheral blood. These findings suggest that LT is essential in the normal development of peripheral lymphoid organs.111 Lymphocytes positive for immunoglobulin M are present in increased numbers in both the spleen and peripheral blood. The membrane-bound LT forms heterotrimers, i.e., LT-1-2, which bind to the LT receptor (LT-R). The LT-1-2 triggers expression of kinase NIK, which induces the nuclear transcription factor k-B and initiates an alternative pathway for its activation, which plays a pivotal role in innate and adaptive immunity.112 The interaction of LT 1-2 with its receptor LTR constitutes a major pathway in the development of the architecture and the function of the secondary lymphoid organs 113 for adaptive immune responses. These findings document the crucial roles of LT- and LT-R in building and maintaining the architecture of lymphoid organs and ensuring the function of the immune response against invading pathogens.
Chronic inflammation, autoimmunity, or cancer may interfere with the development of secondary lymphoid organs; and the maintenance of their microarchitecture depends on interactions of cells of hematopoietic and nonhematopoietic origin, as well as the chemokines CXCR5 and CCR7.114,115
The chemokines CCL12 and CCL19, which bind to chemokine receptor CCR7, and the CXCL13, which binds to receptor CXCR5, are important for the colonization of lymphoid organs by T and B lymphocytes and antigen-presenting cells.114 The enzyme IKK kinase limits gene activation in inflammatory responses, and downregulates signaling by the transcription factor NF-κB. Upon completion of the connective tissue skeleton the first cells which colonize the LN in mice express the CD4+CD3− phenotype, and after stimulation with IL-2, differentiate to NK cells, or, depending on the combination of cytokines, will give rise to antigen-presenting cells. The precursors of these cells are CD45+/CD4+/CD3− and IL7 R+ residing in the liver.116
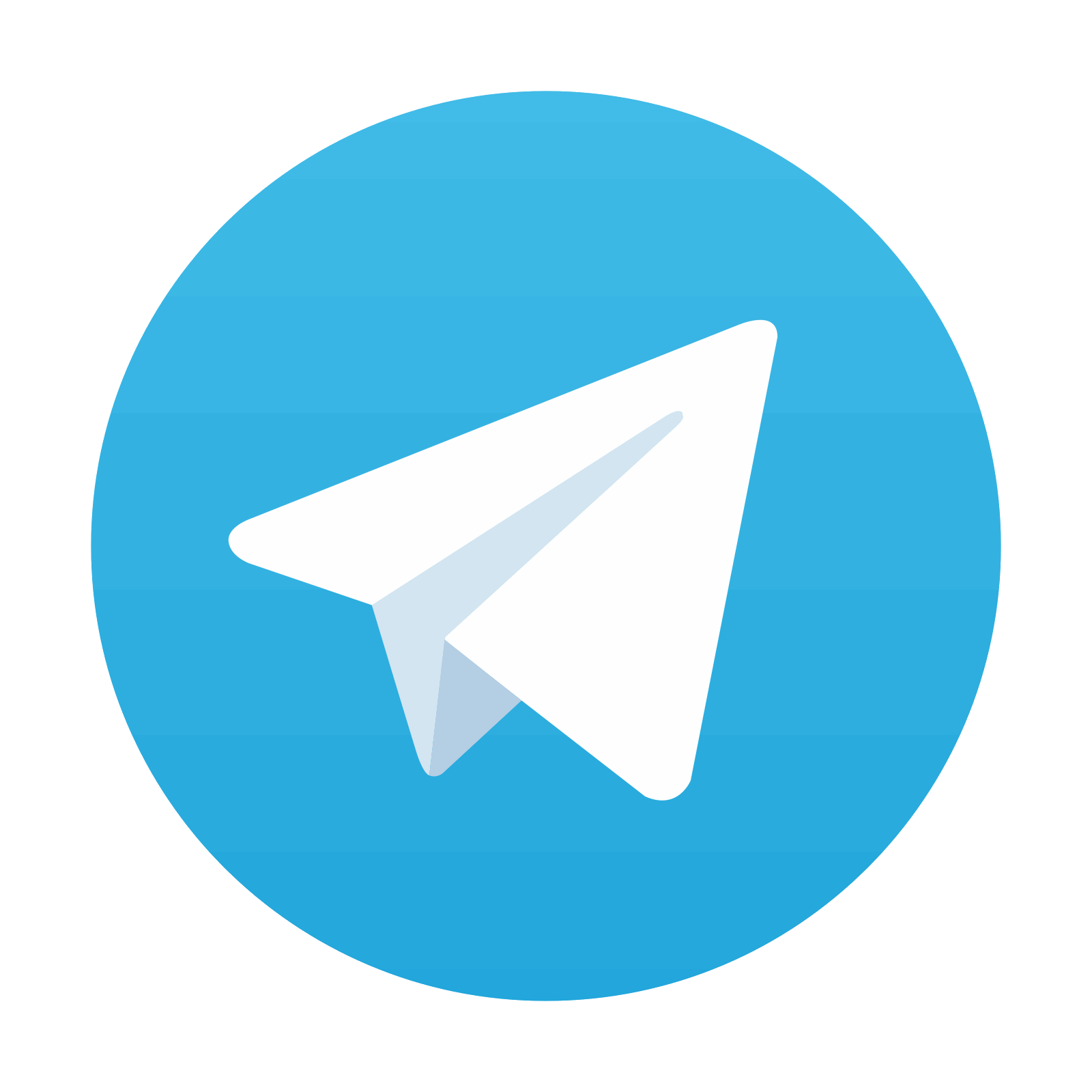
Stay updated, free articles. Join our Telegram channel

Full access? Get Clinical Tree
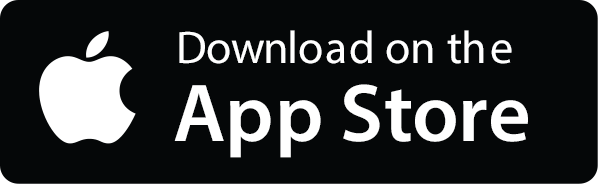
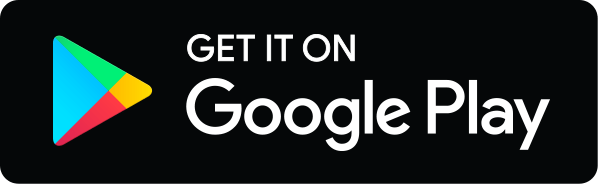
