Fig. 9.1
Structures involved in adrenergic signaling. (a) Host hormones epinephrine and norepinephrine. (b) The structure of AI-3 has not been solved, but may resemble epinephrine and norepinephrine. (c) The structure of LED209 that inhibits QseC signaling
9.5 Infectious Disease and Hormones
Eukaryotic cell-to-cell signaling is based on a variety of hormones, which are essential for eukaryotic development and homeostasis. Significantly, the hormones epinephrine and norepinephrine also promote EHEC growth and are co-opted as signals that EHEC uses to modulate expression of virulence traits (Lyte and Ernst 1992; Lyte et al. 1996; Freestone et al. 2000; Sperandio et al. 2003). Epinephrine and norepinephrine belong to the class of hormones called catecholamines. These hormones are derived from the amino acid tyrosine and are composed of a catechol and a side-chain amine. Epinephrine and norepinephrine are the most abundant catecholamines in the human body and are involved in the fight or flight response. Epinephrine and norepinephrine are present at micromolar concentrations in the intestine (Eldrup and Richter 2000) and play important roles in physiology of the GI tract by modulating smooth muscle contraction, submucosal blood flow, and chloride and potassium secretion (Horger et al. 1998). In addition to the central nervous system and adrenal medulla, the adrenergic neurons that are present in the enteric nervous system are the major sources of epinephrine and norepinephrine (Furness 2000; Purves et al. 2001). Additionally, immune cells including T cells, macrophages, and neutrophils produce and secrete epinephrine and norepinephrine (Flierl et al. 2008). Therefore, bacterial infections may results in increased epinephrine and norepinephrine concentrations in the GI tract due to the stress of the infection in conjunction with the immune response. Finally, the commensal GI microbiota also contribute to the generation of biologically active norepinephrine (and to a lesser extent epinephrine) in the lumen of the GI tract (Asano et al. 2012).
9.6 Bacterial Adrenergic Receptors
The mammalian adrenergic receptors that bind epinephrine and norepinephrine and transmit signals are called G-coupled protein receptors. GPCRs are transmembrane receptors are coupled to heterotrimeric guanine-binding proteins (G proteins). EHEC does not encode G proteins; therefore, EHEC senses epinephrine and norepinephrine via a different mechanism. The main signaling transduction systems in bacteria are two component systems (TCSs) (Clarke et al. 2006). TCSs are critical for bacteria to sense and respond to changes in the environment. A typical TCS is composed of a histidine sensor kinase (HK) located in the cytoplasmic membrane that perceives a stimulus and a cytoplasmic response regulator (RR) that controls the output (Jung et al. 2012). Upon sensing a specific environmental cue, the kinase autophosphorylates at a specific histidine residue and subsequently transfers this phosphate to an aspartate residue on its cognate RR. RRs are transcription factors that mediate the output of this signaling cascade by binding DNA to promote or repress gene expression (Jung et al. 2012). EHEC encodes two adrenergic receptors, QseC and QseE (Clarke et al. 2006; Reading et al. 2009), that upon sensing epinephrine and norepinephrine initiate a complex signaling cascade, which results in coordinated expression of virulence genes (Sperandio et al. 1999, 2002a, b, 2003; Clarke et al. 2006; Kendall et al. 2007; Reading et al. 2007, 2009; Hughes et al. 2009; Njoroge and Sperandio 2012; Pacheco et al. 2012; Gruber and Sperandio 2014) (summarized in Fig. 9.2).
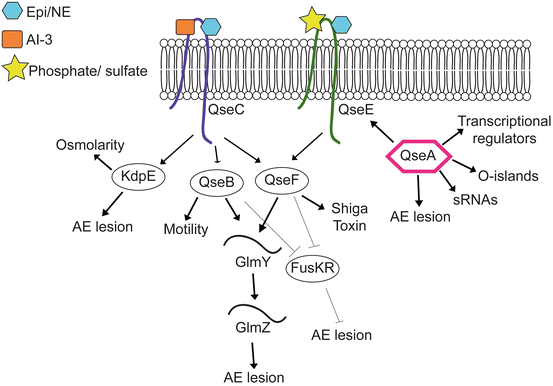
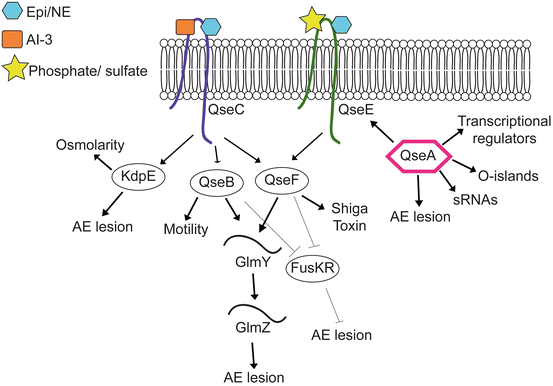
Fig. 9.2
Summary of the epinephrine/NE/AI-3 signaling cascade in EHEC. Arrows indicate positive regulation and lines with bars indicate negative regulation
9.7 The QseBC TCS
The gene encoding QseC was first identified in an array that compared gene expression between wild type (WT) EHEC and a luxS mutant (Sperandio et al. 2002a, b), and subsequent studies revealed that QseC directly senses, and autophosphorylates, in response to host-derived epinephrine and norepinephrine as well as the bacterial-derived AI-3 (Clarke et al. 2006). QseC is a global regulator in EHEC and influences expression of more than 400 genes in response to epinephrine and AI-3 (Hughes et al. 2009). QseC directs expression of genes involved in cell metabolism, virulence, motility and stress responses (Hughes et al. 2009). To mediate these responses, QseC phosphorylates three distinct RRs, QseB, QseF, and KdpE (Hughes et al. 2009). QseB activates genes encoding flagella and motility (Sperandio et al. 2002a, b); QseF coordinates expression of genes encoding AE lesions and stress responses (Reading et al. 2007); and KdpE regulates genes encoding potassium uptake, osmolarity, and AE lesion formation (Nakashima et al. 1992; Hughes et al. 2009; Njoroge et al. 2012). Adrenergic signaling is essential for EHEC virulence during infection, as a qseC mutant strain is attenuated for virulence in rabbit-infection models (Clarke et al. 2006; Rasko et al. 2008).
9.8 The QseEF TCS
A second TCS involved in adrenergic signaling was identified in a microarray study that compared differential gene expression in WT and the luxS mutant EHEC strains. This TCS was renamed QseEF, where QseE is the HK and QseF is the RR (Reading et al. 2007). The qseE and qseF genes are encoded within a polycistronic operon that also contains the yfhG gene, which encodes an uncharacterized protein, as well as glnB, which encodes the PII protein involved in nitrogen regulation (Reading et al. 2007). QseE senses epinephrine and norepinephrine, as well as the environmental signals phosphate and sulfate, but does not sense AI-3. Therefore, QseE functions to sense strictly host-derived signals, in contrast to QseC that senses host- and bacterial-derived molecules (Clarke et al. 2006; Reading et al. 2009). Finally, QseC activates transcription of qseEF, and therefore, in the epinephrine and norepinephrine signaling cascade, QseE is downstream of QseC (Reading et al. 2007).
QseEF regulates expression of genes involved in the SOS response and Shiga toxin production, as well as transcription of genes encoding for other TCSs, including RcsBC and PhoPQ (Reading et al. 2009, 2010; Njoroge and Sperandio 2012). Additionally, QseEF influences AE lesion formation through regulation of EspFu/TccP (Reading et al. 2007). EspFu/TccP is an effector encoded outside of the LEE that enhances AE lesion formation (Campellone et al. 2004; Garmendia et al. 2004). The LEE-encoded T3SS translocates EspFu into the host cell where it mimics the eukaryotic SH2/SH3 adapter protein and leads to actin polymerization during AE lesion formation (Campellone et al. 2004). Bioinformatic analyses revealed that QseF contains a σ54 activator domain, whereas the espFu gene contains a conserved σ70 promoter, suggesting that QseF regulation of EspFu was indirect. Moreover, purified QseF did not bind to the espFu promoter in electrophoretic mobility shift assays. Together, these data confirmed that QseEF regulation of EspFu requires an intermediate factor. Subsequent studies revealed that QseF regulates the sRNA GlmY, which is located immediately upstream from the qseGFglnB operon (Reichenbach et al. 2009). More recently, Gruber and Sperandio reported that GlmY, acting in concert with a second sRNA GlmZ, is the link between QseF and EspFu (Gruber and Sperandio 2014). Interestingly, GlmY and GlmZ promote espFu translation through cleavage of the transcript and negatively regulate expression of the LEE4 and LEE5 operons through destabilization of the mRNA (Gruber and Sperandio 2014).
9.9 Interplay Between QseBC and QseEF Sensing Systems
Single deletion strains of qseC or qseE are able to modulate gene expression in an epinephrine-dependent manner, whereas, qseC/qseE double mutant does not respond to epinephrine (Njoroge and Sperandio 2012). These findings suggest that QseC and QseE are the only adrenergic receptors in EHEC. QseC and QseE display convergent regulation of some target genes while differentially regulating others (Njoroge and Sperandio 2012). For example, QseB also promotes expression of GlmY, and thus regulates EspFu expression (Gruber and Sperandio 2014). Moreover, QseBC and QseEF negatively regulate expression of the TCS FusKR (Pacheco and Sperandio 2012). The HK FusK senses fucose in the GI tract, which EHEC uses to determine its location in the GI tract and correctly time expression of the LEE genes (Pacheco and Sperandio 2012). Further characterization of these regulatory cascades will provide a clearer understanding of how EHEC coordinates expression of these TCSs in order to precisely regulate virulence genes.
9.10 The Transcriptional Regulator QseA
QseA is a LysR-family transcriptional regulator that is activated by the AI-3/epi/NE signaling cascade (Sperandio et al. 1999, 2002a, b). QseA plays an important role in promoting ECHE virulence. QseA activates transcription of ler, and hence all the LEE genes (Sperandio et al. 2002a, b). The LEE1 operon contains two promoters, a distal P1 promoter, and a proximal P2 promoter (Mellies et al. 1999; Sperandio et al. 2002a, b). QseA binds both promoters to regulate ler expression (Sperandio et al. 2002a, b; Kendall et al. 2010). Consistent with the transcriptional data, a qseA mutant strain formed significantly less AE lesions compared to WT EHEC (Sperandio et al. 2002a, b). Subsequent studies demonstrated that QseA regulates grlRA transcription in a Ler-dependent and Ler-independent mechanism and also showed that QseA regulon extends beyond the LEE and includes genes encoded in O-islands, which are regions of the chromosome unique to EHEC (Hayashi et al. 2001; Perna et al. 2001), other transcriptional regulators, sRNAs, as well as qseE (Reading et al. 2007; Kendall et al. 2010).
9.11 Disruption of AI-3/Epi/NE Signaling as an Antivirulence Strategy
Bacterial infections may lead to severe morbidity and mortality; however, the ability to treat these diseases with conventional antibiotics is becoming more and more limited. This is due primarily to the fact that antibiotics have lost their effectiveness as many bacteria are becoming resistant, often to multiple types of antibiotics. Conventional antibiotics disrupt essential functions, including DNA replication and protein synthesis, and thus place selective pressure on bacteria to develop resistance. An alternative approach may be to develop anti-virulence drugs that target bacterial virulence, but that does not inhibit bacterial growth or lead to death of the bacterial cell (Rasko and Sperandio 2010).
QseC homologues are present in over 25 plant and animal pathogens (Rasko et al. 2008). Thus, disrupting QseC signaling may be an effective strategy to inhibit virulence. Indeed, a high throughput screen identified a small, synthetic compound called LED209 (Fig. 9.1c) that blocked QseC signaling and prevented virulence expression not only in EHEC, but also in enteroaggregative E. coli, Salmonella enterica serovar Typhimurium, and Francisella tularensis (Rasko et al. 2008; Curtis et al. 2014). LED209 functions as a prodrug that inhibits virulence by binding to and allosterically modifying QseC to disrupt activity (Curtis et al. 2014). LED209 specifically targets QseC and does not inhibit pathogen growth, suggesting that LED209 will not place selective pressure on pathogens to evolve resistance. An issue with an inhibitor of adrenergic signaling is that it may present adverse effects on the host. Significantly, LED209 did not present toxicity in cell culture or in rodents (Curtis et al. 2014), and future studies will need to be performed to confirm non-toxicity and efficacy in humans. Finally, some bacterial infections, including infections caused by Clostridium difficile and Salmonella, are associated with antibiotic use that disrupts the resident microbiota. Therefore, another important issue to be addressed concerns the effects of LED209 on the resident GI microbiota (Curtis and Sperandio 2011). Nevertheless, these recent findings underscore the potential of disrupting chemical signaling as a novel and effective antivirulence approach to treat diverse infectious diseases.
References
Abe A, Kenny B, Stein M, Finlay BB (1997) Characterization of two virulence proteins secreted by rabbit enteropathogenic Escherichia coli, EspA and EspB, whose maximal expression is sensitive to host body temperature. Infect Immun 65:3547–3555PubMedCentralPubMed
Barba J, Bustamante VH, Flores-Valdez MA, Deng W, Finlay BB, Puente JL (2005) A positive regulatory loop controls expression of the locus of enterocyte effacement-encoded regulators Ler and GrlA. J Bacteriol 187(23):7918–7930PubMedCentralCrossRefPubMed
Bhatt S, Edwards AN, Nguyen HTT, Merlin D, Romeo T, Kalman D (2009) The RNA binding protein CsrA is a pleiotropic regulator of the locus of enterocyte effacement pathogenicit island of enteropathogenic Escherichia coli. Infect Immun 77:3552–3568PubMedCentralCrossRefPubMed
Bhatt S, Romeo T, Kalman D (2011) Honing the message: post-transcriptional and post-translational control in attaching and effacing pathogens. Trends Microbiol 19:217–224PubMedCentralCrossRefPubMed
Clarke MB, Hughes DT, Zhu C, Boedeker EC, Sperandio V (2006) The QseC sensor kinase: a bacterial adrenergic receptor. Proc Natl Acad Sci 103(27):10420–10425PubMedCentralCrossRefPubMed
Curtis MM, Sperandio V (2011) A complex relationship: the interaction among symbiotic microbes, invading pathogens, and their mammalian host. Mucosal Immunol 4:133–138PubMedCentralCrossRefPubMed
Curtis MM, Russell R, Moreira CG, Adebesin AM, Wang C, Williams NS, Taussig R, Stewart D, Zimmern P, Lu B, Prasad RN, Zhu C, Rasko DA, Huntley JF, Falck JR, Sperandio V (2014) QseC inhibitors as an antivirulence approach for Gram-negative pathogens. mBio 5:e02165. doi:10.1128/mBio.02165-14 PubMedCentralCrossRefPubMed
Deng W, Puente JL, Gruenheid S, Li Y, Vallance BA, Vazquez A, Barba J, Ibarra JA, O’Donnell P, Metalnikov P, Ashman K, Lee S, Goode D, Pawson T, Finlay BB (2004) Dissecting virulence: systematic and functional analyses of a pathogenicity island. Proc Natl Acad Sci 101:3597–3602PubMedCentralCrossRefPubMed
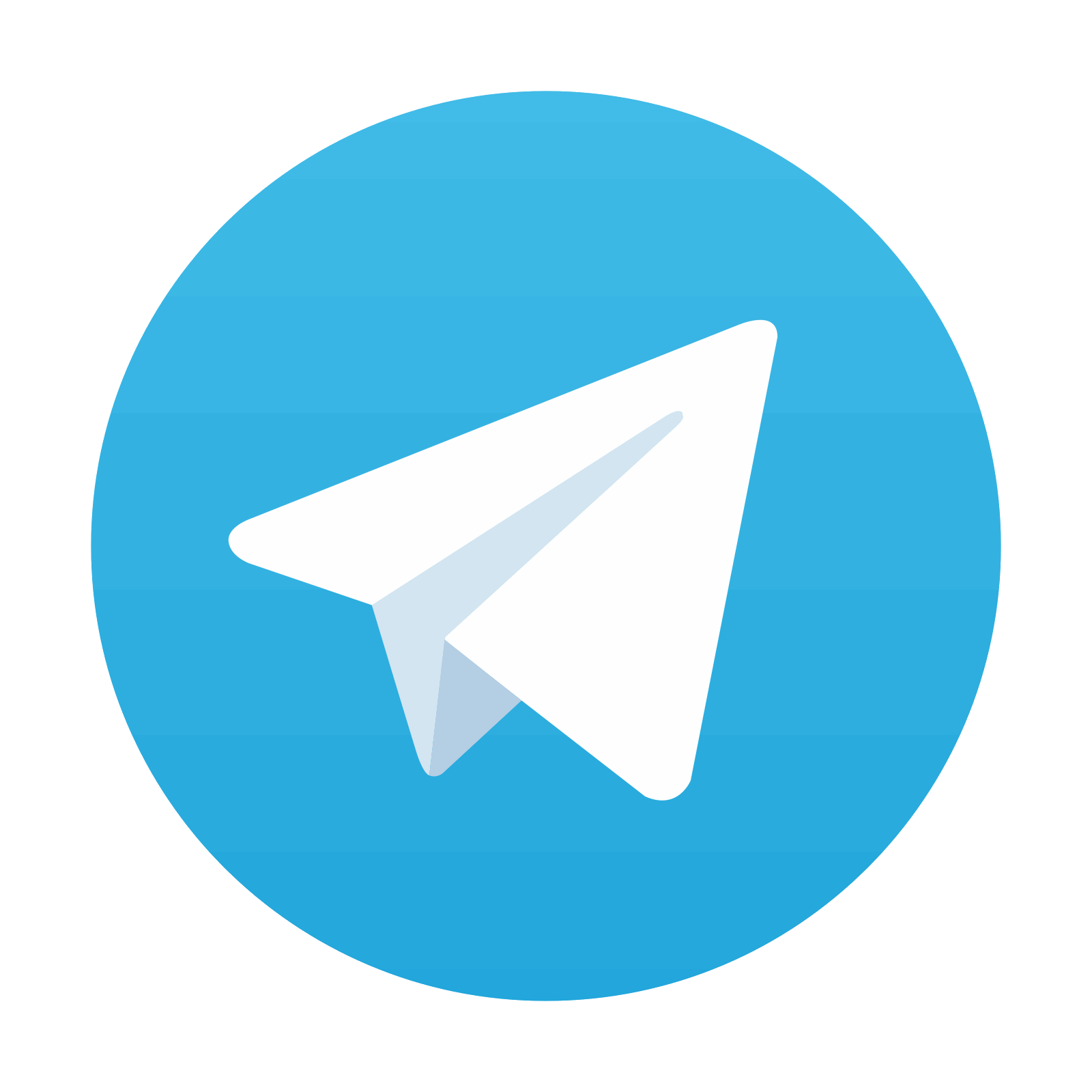
Stay updated, free articles. Join our Telegram channel

Full access? Get Clinical Tree
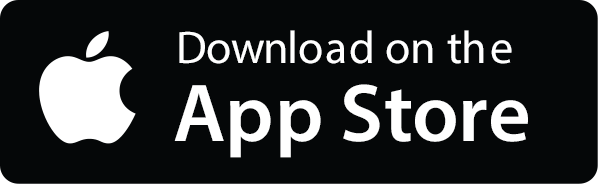
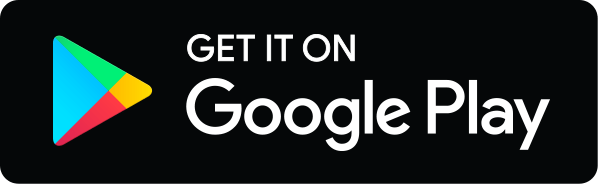