in a different channel. The interference is corrected by applying fluorescence compensation based on data from single-stained samples. This is usually done using cells or beads before or during the data acquisition phase. However, modern FCM data analysis software also allows collection of uncompensated data and applying compensation during analysis. Before data acquisition, standard reference particles (fluorescent microspheres) should be used to adjust the PMT voltage settings so that the beads fall in approximately the same location or the same “target channels,” predetermined for each fluorochrome.
![]() FIGURE 2.1. Principles of multicolor flow cytometry. A single cell suspension is hydrodynamically focused with sheath fluid to intersect lasers (three-laser system is shown). Fluorescence signals are collected by multiple fluorescence emission detectors, separate for every laser. Examples of fluorochromes detected by different lasers are given according to Table 2.1. Detected signals are amplified by photomultiplier tubes and converted to digital form for analysis. |
TABLE 2.1 TABLE OF FLUOROCHROMES COMMONLY USED IN CLINICAL FLOW CYTOMETRY | ||||||||||||||||||||||||||||||||||||||||||||||||||||||||||||||||||||||||||||||||||||||||||||||||||||||||||||||||||||||||||||||||||||||||||||||||||||
---|---|---|---|---|---|---|---|---|---|---|---|---|---|---|---|---|---|---|---|---|---|---|---|---|---|---|---|---|---|---|---|---|---|---|---|---|---|---|---|---|---|---|---|---|---|---|---|---|---|---|---|---|---|---|---|---|---|---|---|---|---|---|---|---|---|---|---|---|---|---|---|---|---|---|---|---|---|---|---|---|---|---|---|---|---|---|---|---|---|---|---|---|---|---|---|---|---|---|---|---|---|---|---|---|---|---|---|---|---|---|---|---|---|---|---|---|---|---|---|---|---|---|---|---|---|---|---|---|---|---|---|---|---|---|---|---|---|---|---|---|---|---|---|---|---|---|---|---|
|
A droplet generator
A droplet charging and deflecting system
A collection component
The electronic circuitry for coordinating the timing and generation of droplet-charging pulses
cell lines (hybridomas) that produce an antibody of unique specificity, avidity, and isotype can be established. In the early days of the application of MAbs to immunology, many laboratories were immunizing mice with leukocytes. The obtained hybridomas produced many antibodies that reacted with leukocytes, but the identities of the molecular targets were not known. The reactivity spectrum of the antibody could be described by staining multiple different cell types, and in most cases the target antigen could be isolated by immunoprecipitation or Western blotting and its molecular weight and other structural characteristics determined.
TABLE 2.2 LIST OF CD ANTIGENS MOST COMMONLY USED IN FLOW CYTOMETRY IMMUNOPHENOTYPING OF HEMATOLOGIC SAMPLES | |||||||||||||||||||||||||||||||||||||||||||||||||||||||||||||||||||||||||||||||||||||||||||||||||||||||||||||||||||||||||||||||||||||||||||||||||||||||||||||||||||||||||||||||||||||||||||||||||||
---|---|---|---|---|---|---|---|---|---|---|---|---|---|---|---|---|---|---|---|---|---|---|---|---|---|---|---|---|---|---|---|---|---|---|---|---|---|---|---|---|---|---|---|---|---|---|---|---|---|---|---|---|---|---|---|---|---|---|---|---|---|---|---|---|---|---|---|---|---|---|---|---|---|---|---|---|---|---|---|---|---|---|---|---|---|---|---|---|---|---|---|---|---|---|---|---|---|---|---|---|---|---|---|---|---|---|---|---|---|---|---|---|---|---|---|---|---|---|---|---|---|---|---|---|---|---|---|---|---|---|---|---|---|---|---|---|---|---|---|---|---|---|---|---|---|---|---|---|---|---|---|---|---|---|---|---|---|---|---|---|---|---|---|---|---|---|---|---|---|---|---|---|---|---|---|---|---|---|---|---|---|---|---|---|---|---|---|---|---|---|---|---|---|---|---|
|
Mature lymphocytes are characterized by low side scatter and strong CD45 expression (lymph region, Fig. 2.2 plot B).
Monocytes have higher SS and strong CD45 expression (monocyte region, Fig. 2.2 plot B).
Erythropoietic precursors are CD45 negative and have low SS (CD45- ery region, Fig. 2.2 plot B).
Granulopoietic precursors and granulocytes are weakly CD45 positive and have high SS (CD45 dim, gran region, Fig. 2.2 plot B).
Early hematopoietic precursors of various lineages, including CD34+ stem cells, are characterized by low CD45 expression and low SS (blast region, Fig. 2.2, plot B).
Histograms (for one parameter), where relative fluorescence or scatter is on the x-axis and the number of events with given characteristics on the y-axis
Two-parameter dot plots, where each signal is visualized by one dot and given a parameter on the x– and y-axes; various cell populations can be then “painted” with different colors
Density plots, where hotspots indicate large numbers of events resulting from discreet population of cells and colors can give the graph a three-dimensional feel
Contour diagrams, where joined lines represent similar numbers of cells
Demographic identification of patient
Identification of the hospital or division sending the sample
Type of specimen (bone marrow aspirate, peripheral blood, other biologic fluids)
Timing of observation (first diagnosis or follow-up)
Diagnostic hypothesis made by the sender
List of antigens and type of immunofluorescence analysis carried out
Absolute number of cells in the sample
Quality of the sample, in terms of viability
General description of the gating procedure
Immunophenotype of abnormal cells present in the sample
Description of other (normal) cells
FIGURE 2.3. A. Examples of analysis of B-cell compartment in bone marrow samples. Ten-color MAb panel, Navios flow cytometer, and Kaluza software (Beckman Coulter) were applied. Panel consists of Kappa-FITC/Lambda-PE/CD19 ECD/CD34-APC/CD10-APC-A750/CD23-APC-A700/CD20-PC7/CD38-PC5.5/CD5 Pc Blue/CD45-Krom Orange. The live cell gate is created and fractions of lymphocytes, granulocytes, monocytes, and the like are evaluated as shown in Figure 2.2. The B-cell gate is created on a CD19/SS plot and expression of CD5, CD23, and CD10 is analyzed within the B-cell population. Kappa and lambda light chain expression is analyzed within total B-cell, CD5+ B-cell, or CD10+ B-cells as appropriate. Expression of CD34 and CD38 within the C19+ B-cell population can also be analyzed (see Fig. 2.3B). The fraction of plasma cells can be estimated using CD38 bright expression and high SS on the CD38/SS plot (not shown). Upper row: population of B-cells with B-CLL/small lymphocytic lymphoma-related phenotype (CD19+, CD5+, CD23+, CD20 dim, kappa dim, CD10-) consistent with bone marrow involvement in a patient who was diagnosed with small lymphocytic lymphoma in a lymph node biopsy and had no peripheral lymphocytosis. Bone marrow biopsy showed rare nodular lymphoid infiltrates. Middle row: CD5-CD10-CD23- lambda+ B-cell population in a patient with Waldenström macroglobulinemia. Lower row: population of CD19+ CD10+ B-cells strongly expressing CD20 and kappa in a patient with bone marrow involvement by a follicular lymphoma. B. Examples of analysis of B-cell compartment in a lymph node cell suspension. Ten-color MAb panel, Navios flow cytometer, and Kaluza software (Beckman Coulter) were applied. Panel consists of Kappa-FITC/Lambda-PE/CD19 ECD/CD34-APC/CD10-APC-A750/CD23-APC-A700/CD20-PC7/CD38-PC5.5/CD5 Pc Blue/CD45-Krom Orange. The live cell gate is created and fractions of lymphocytes, granulocytes, monocytes, and the like are evaluated as shown in Figure 2.2. The B-cell gate is created on the CD19/SS plot and expression of CD5, CD23, and CD10 is analyzed within the B-cell population. Most B-cells were positive for CD20, CD38, and CD10, and showed monotypic lambda expression.
Diagnostic conclusions
methodology, interlaboratory comparison, or verification with specimens obtained from patients with a confirmed diagnosis. A minimum of 10 to 20 samples (10 normal, 10 abnormal) is recommended for accuracy assessment. The acceptance criteria will also be variable depending on the required degree of accuracy for the intended use, nevertheless should be clearly defined for each assay. Ninety percent, or greater, agreement between methods is generally required for accuracy.
TABLE 2.3 EXAMPLES OF 10-COLOR FLOW CYTOMETRY PANELSa IN IMMUNOPHENOTYPING OF LEUKEMIA AND LYMPHOMA | ||||||||||||||||||||||||||||||||||||||||||||||||||||||||||||||||||||||||||||||||||||||||||||||||||||||||||||||
---|---|---|---|---|---|---|---|---|---|---|---|---|---|---|---|---|---|---|---|---|---|---|---|---|---|---|---|---|---|---|---|---|---|---|---|---|---|---|---|---|---|---|---|---|---|---|---|---|---|---|---|---|---|---|---|---|---|---|---|---|---|---|---|---|---|---|---|---|---|---|---|---|---|---|---|---|---|---|---|---|---|---|---|---|---|---|---|---|---|---|---|---|---|---|---|---|---|---|---|---|---|---|---|---|---|---|---|---|---|---|
|
patterns. Following reports by Terstappen et al.,29,30 and 31 several groups provided descriptions of clearly delineated differentiation stages of various hematopoietic cell lineages.32,33,34,35 and 36,37,38 A detailed review of all available data is beyond the scope of this chapter; a summary of the most important and well-established issues is provided below.
by a decrease in CD44, disappearance of CD45 and acquisition of bright CD235a expression. At transition to the polychromatophilic/orthochromatophilic stage, erythroblasts show loss of HLA-DR, further decrease in CD44, and a mild decrease in CD36.51,52
CD34+CD10+ Terminal deoxynucleotidyl transferase (TdT)+CD79a+CD19neg common lymphoid progenitor (CLP): early B (E-B) stage.
CD34+CD19+CD10+TdT+CD20-cytIgM- pro-B-cell stage.
After down-regulation of CD34 and TdT they become CD34-CD19+ CD10+ CD20 heterogenous pre-B that can be further subdivided in I and II subsets.
CD34-CD19+CD20+CD10dim/- IgM+ immature (IM)-B-cells.
After expression of light chains, cells become CD10-CD19+ CD20+ IgM+ IgD+ mature B-cells.
in reactive BM. Eosinophilic myelocytes can be identified by high side scatter, intermediate CD45 (at a level slightly higher than neutrophilic myelocytes), low to intermediate CD11b, intermediate CD13, and low CD33 with bright CD66b and no CD16 expression. Mature eosinophils show increased levels of CD45 and CD11b with a decrease in CD33 and are negative for CD16.51,64
TABLE 2.4 SURFACE MARKER EXPRESSION DURING MATURATION OF GRANULOPOIETIC PRECURSORS IN THE BONE MARROW | ||||||||||||||||||||||||||||||||||||||||||||||||||||||||||||||||||||||||||||||||||||||||||||||||||||||||||||||||||||||||||||||||||||||||||||||||||||||||||||||||||||||||||||||||||||||||||||||||||||
---|---|---|---|---|---|---|---|---|---|---|---|---|---|---|---|---|---|---|---|---|---|---|---|---|---|---|---|---|---|---|---|---|---|---|---|---|---|---|---|---|---|---|---|---|---|---|---|---|---|---|---|---|---|---|---|---|---|---|---|---|---|---|---|---|---|---|---|---|---|---|---|---|---|---|---|---|---|---|---|---|---|---|---|---|---|---|---|---|---|---|---|---|---|---|---|---|---|---|---|---|---|---|---|---|---|---|---|---|---|---|---|---|---|---|---|---|---|---|---|---|---|---|---|---|---|---|---|---|---|---|---|---|---|---|---|---|---|---|---|---|---|---|---|---|---|---|---|---|---|---|---|---|---|---|---|---|---|---|---|---|---|---|---|---|---|---|---|---|---|---|---|---|---|---|---|---|---|---|---|---|---|---|---|---|---|---|---|---|---|---|---|---|---|---|---|---|
|
TABLE 2.5 AVERAGE RELATIVE FREQUENCY OF MAJOR LYMPHOID CELL SUBSETS IN NORMAL TISSUES | |||||||||||||||||||||||||||||||||||||||||||||||||||||||||||||||||||||
---|---|---|---|---|---|---|---|---|---|---|---|---|---|---|---|---|---|---|---|---|---|---|---|---|---|---|---|---|---|---|---|---|---|---|---|---|---|---|---|---|---|---|---|---|---|---|---|---|---|---|---|---|---|---|---|---|---|---|---|---|---|---|---|---|---|---|---|---|---|
|
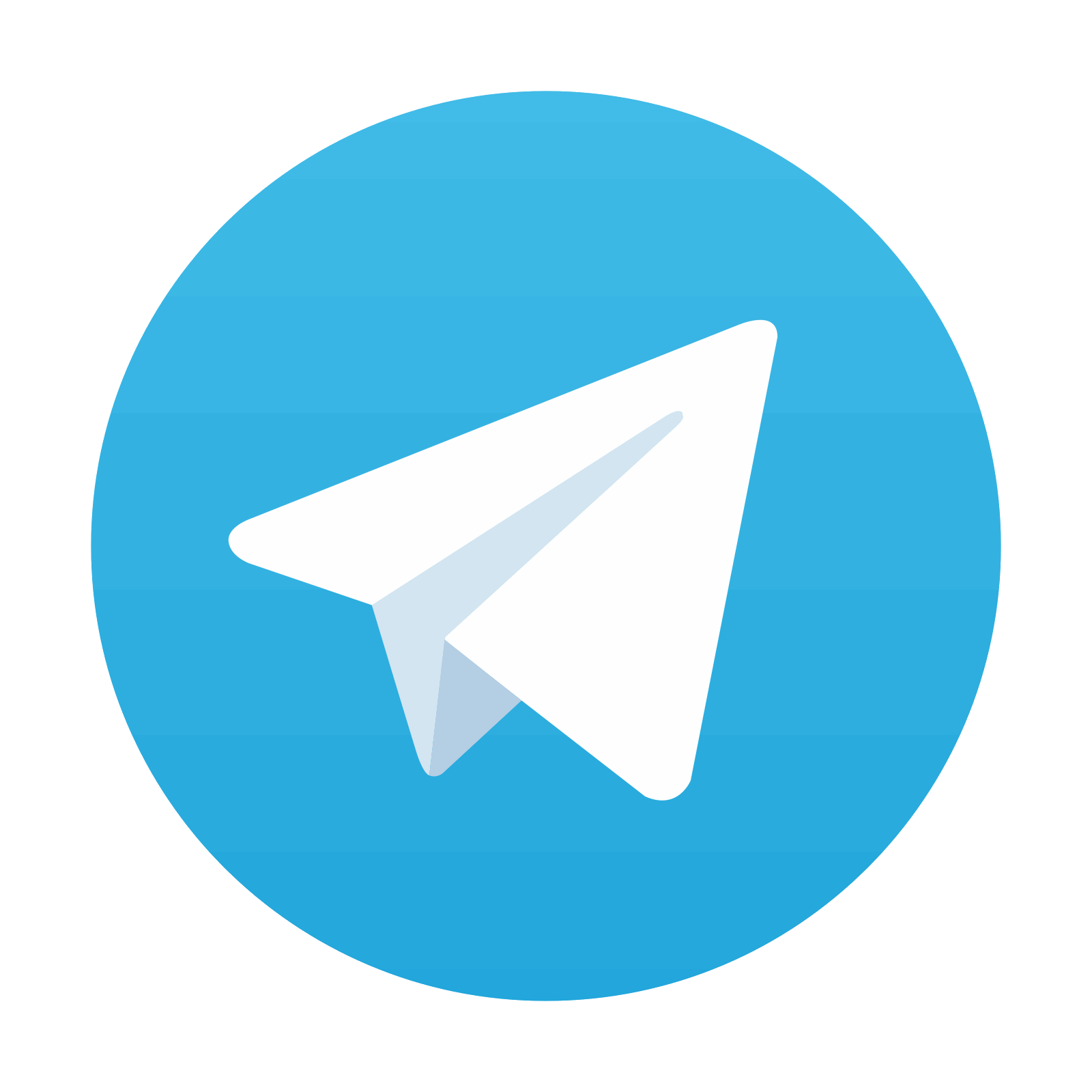
Stay updated, free articles. Join our Telegram channel

Full access? Get Clinical Tree
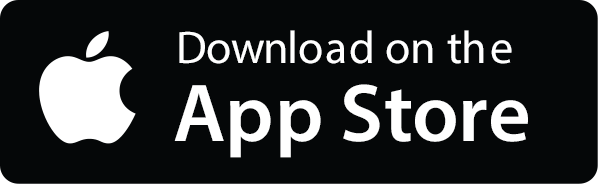
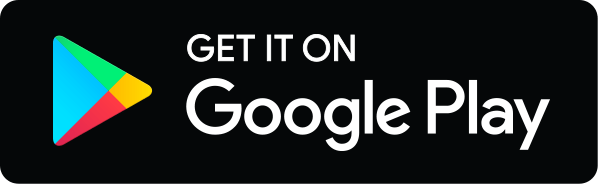