Paul S. Mead
Yersinia Species (Including Plague)
The genus Yersinia includes 18 described species, of which 3 are important human pathogens.1 Two of these, Y. enterocolitica and Y. pseudotuberculosis, are enteric pathogens usually acquired through ingestion of contaminated food or water. In humans these agents cause acute enteritis and mesenteric lymphadenitis (pseudoappendicitis). The third species, Y. pestis, causes plague. Although closely related to Y. pseudotuberculosis, Y. pestis has undergone a marked evolutionary shift to become a highly invasive flea-transmitted disease. Humans are incidental hosts for all three pathogens, which otherwise circulate among rodents, pigs, birds, insects, and other domestic and wild animals. Several Yersinia species include organisms that were formerly considered biochemically atypical isolates of Y. enterocolitica but have been reclassified as Y. intermedia, Y. frederiksenii, and Y. kristensenii. Y. intermedia and Y. frederiksenii have been recovered rarely from patients with enterocolitis and in a few instances have been associated with soft tissue infections.
Yersinia Pestis
History
Plague has been credited with causing at least three major pandemics over the past 1500 years.2 The first struck the Byzantine Empire during the sixth century, killing an estimated 40 million persons in the Mediterranean basin. The second pandemic began in Central Asia and spread west along caravan routes to reach the Crimean Sea port of Kaffa (now Feodosiya in Ukraine) in 1346.3 The Mongols, who were battling the Genoese for control of the city, catapulted bodies of plague victims over the city walls. Although it is not clear how effective this was in transmitting infection, it is the first reported use of plague as a weapon. Genoese ships returning to Italy soon introduced plague into several port cities, and from 1347 to 1354 the “Black Death” spread swiftly through Europe and the British Isles, killing an estimated one third of the population.4 The epidemic advanced so quickly that some historians have questioned whether it was actually caused by plague.5 Nevertheless, recent evidence from archeological and transmission studies strongly supports the role of Y. pestis as the cause of the Black Death.6–8 Plague persisted in Europe, causing periodic outbreaks, for the next 300 years.
The third or “modern pandemic” began in China’s Yunnan Province in the 1850s. Infection spread along trade routes to port cities, including Hong Kong, where Alexandre Yersin isolated the causative agent in 1894. Four years later, Paul-Louis Simond identified the plague bacillus in the tissues of dead rats and subsequently proposed a role for fleas in transmission. Over the ensuing 20 years, the newly invented steamship helped disseminate Y. pestis–infected rats and fleas to port cities worldwide, including several in North America. Rat-associated plague was soon brought under control in most urban areas; however, infection spread to other species of rodents, becoming entrenched in rural areas of the Americas, Africa, and Asia. In the first half of the 20th century, India was most severely affected by plague epidemics, with more than 20 million cases and 10 million deaths. Plague was an important problem in war-torn Vietnam, where thousands of cases were reported annually during the 1960s and 1970s.9 Recently, plague has resurged in the nations of sub-Saharan Africa and the adjacent island of Madagascar, areas that now account for more than 95% of cases reported to the World Health Organization (WHO).10,11
Along with its historical importance, Y. pestis has considerable potential as a biological weapon. During World War II, the Japanese military dropped clay pots with plague-infected fleas over areas of China, apparently causing outbreaks. Both the United States and the Soviet Union evaluated Y. pestis as a potential weapon during the Cold War era, and in the 1980s a Soviet defector to Great Britain revealed that the Russians had succeeded in preparing a powdered aerosolized form, genetically engineered to be resistant to several antibiotics. The potential for misuse by terrorists is considered an important national security threat, requiring special measures for medical and public health preparedness.12 The plague bacillus is designated as a Tier I (formerly Category A) select agent whose handling is regulated by federal law.13,14
Microbiology
Y. pestis is an aerobic, gram-negative coccobacillus that exhibits bipolar staining with Giemsa, Wright’s, and Wayson stains. A member of the Enterobacteriaceae, it grows well on blood or MacConkey agar and in nutrient broths such as brain-heart infusion. When incubated at 37° C, small colonies 1 to 2 mm in diameter are visible on blood or MacConkey agar after 24 to 48 hours. At 72 hours, colonies grown on blood agar can take on a raised, irregular, “fried egg” morphology. Y. pestis does not form spores and, unlike Y. enterocolitica and Y. pseudotuberculosis, is nonmotile when incubated at lower temperatures. It does not ferment lactose and is citrate, urease, and indole negative.15
Genomic studies indicate that Y. pestis evolved from the enteric pathogen Y. pseudotuberculosis as recently as 1500 to 20,000 years ago.16 The transition from enteric to flea-borne pathogen required the ability to survive in the flea gut and to achieve high concentrations in the blood of mammalian hosts. These traits were endowed in part through the acquisition of two plasmids encoding factors that are differentially expressed at temperatures encountered in fleas and mammals.17–19 The 110-kb pMT1/pFra plasmid encodes both Yersinia murine toxin (Ymt), which is necessary for colonization of the flea midgut, and the fraction 1 (F1) envelope antigen, which inhibits phagocytosis in mammals (Table 231-1). The smaller 9.5-kb pPCP1 plasmid encodes a plasminogen activator protein (Pla protease) that is responsible for temperature-dependent coagulase and fibrinolysin activities. The origins of these plasmids remain uncertain; however, approximately half of the DNA sequences of the pMT1/pFra plasmid are shared with a plasmid of Salmonella enterica serotype Typhi.20
TABLE 231-1
Key Virulence and Transmission Factors of Yersinia pestis
LOCATION | FACTOR(S) | PUTATIVE FUNCTION |
pPCP1 plasmid (9.5 kb) | Plasminogen activator (Pla protease) | Protease: targeted activity at mammalian tissue barriers; adhesion: enhances invasion of mammalian cells; coagulase: promotes blockage of flea midgut |
pCD1 plasmid (70-75 kb) | Yersinia outer protein (Yop) virulon | Type III secretion system inhibits phagocytosis and lymphocyte proliferation; transport of effector proteins to host cells |
V antigen | Facilitates intracellular survival; required for type III secretion system translocation pore | |
pMT1/pFra plasmid (∼110 kb) | Fraction 1 antigen | Expressed at higher temperatures; creates capsule that interferes with phagocytosis |
Yersinia murine toxin (Ymt) | Phospholipase D activity upregulated at lower temperatures, necessary for colonization of flea midgut and blockage formation; toxic for mice and rats | |
Chromosome (4.6 Mb) | Hemin storage system (hms) | Proteins produced at lower temperatures; iron acquisition; colonization of flea proventriculus, biofilm production |
Yersiniabactin (Ybt) system | Siderophore; iron acquisition | |
Yersinia Fe uptake system | Adenosine triphosphate–binding cassette transport system; iron acquisition | |
Lipopolysaccharide | Temperature-dependent remodeling of lipid A structure; prevents containment by mammalian immune response | |
pH 6 fimbriae antigen (psa) | Blocks phagocytosis; pH dependent |
Data from references 1, 12, and 14.
As with the other yersiniae, the plague bacillus also has an approximately 70-kb plasmid that mediates expression of virulence factors that prevent production of proinflammatory cytokines, increase resistance to phagocytosis, and enhance intracellular survival.21 Chromosome-mediated factors include a potent lipopolysaccharide endotoxin and a pigmentation factor, the hemin storage locus (hms), that regulates iron uptake and enables the bacteria to form blockages of the flea gut that enhance transmission.17,18
Y. pestis isolates can be classified into three biovars based on their ability to ferment glycerol and convert nitrate to nitrite. It has been postulated that these biovars, Antiqua, Medievalis, and Orientalis, reflect strains associated with the first, second, and third pandemics, respectively. Recent studies suggest, however, that Y. pestis isolates represent as many as eight genetically distinct populations that do not correlate entirely with each biovar.22,23
Epidemiology
Although not the scourge it once was, plague remains a threat in many parts of the world, especially rural Africa (Fig. 231-1).11,24 Routine reporting of cases to the WHO was discontinued in 2004; however, in the preceding 15 years a total of 38,310 cases (average ∼2500 cases per year) and 2845 deaths (7% fatality rate) were reported from 25 countries.25 Nearly 80% of cases were reported from Africa, 15% from Asia, and the remainder from the Americas. Madagascar, Tanzania, the Democratic Republic of the Congo, Vietnam, Mozambique, Namibia, and Peru each accounted for more than 1000 cases. Reports of human plague cases in Algeria and Libya, decades after the last occurrence, underscore the potential for disease reemergence.26 Nearly all cases occur in rural areas, with the exception of a few rat-associated urban outbreaks in the port city of Mahajanga, Madagascar.27
Natural Cycles
The epizootiology of plague is complex and not completely understood.28 Fundamentally a flea-transmitted disease of rodents, plague is found in endemic foci scattered throughout the world. Different foci involve different rodent and flea species, each with their own ecology and predilection for disease. Maintenance in nature is generally assumed to involve enzootic cycles in which relatively resistant rodent species and their associated fleas sustain low-level transmission. These enzootic cycles are believed to pose little risk for human infection (Fig. 231-2). Periodically and perhaps related to climatic conditions, infection spreads to more susceptible rodent species, triggering an amplifying epizootic with mass rodent die-offs and rapid dispersal of infected fleas. Epizootics increase the risk for transmission to humans, especially if they involve rodent species living in close proximity to humans. Worldwide, the most concerning are the peri-domestic rats, Rattus rattus and R. norvegicus, and their highly efficient flea vectors, Xenopsylla cheopis and X. braziliensis. In the western United States, epizootics occur among ground squirrels (Spermophilus spp.), prairie dogs (Cynomus spp.), and chipmunks (Tamias spp.), as well as less familiar rodent species.
Transmission to Humans
Humans are “dead-end” hosts that become infected through flea bites, direct contact with tissues or secretions of infected animals, or rarely through inhalation of infectious aerosols (see Fig. 231-2). Flea-borne transmission is especially common during epizootics when fleas seek alternate blood sources due to the death of their rodent host. Hunters become infected through direct inoculation while skinning or handling carcasses of infected rodents, rabbits and hares, wild cats, and coyotes.29 Direct inoculation is associated with an increased risk for septicemia and death. Domestic pets are also an important source of potential exposure. Domestic cats that eat infected rodents develop pharyngeal infections that can be transmitted directly to humans through respiratory droplets, causing primary pneumonic plague.30 Although dogs rarely become clinically ill with plague, they may play an important role by transporting rodent fleas into the home. A study in New Mexico found that plague patients were more likely than matched controls to have allowed dogs to sleep on their bed at night.31 Once infected, humans who develop pneumonic plague pose a risk for person-to-person transmission to close contacts through respiratory droplets. Limited outbreaks have been reported in northern Africa, the Middle East, and Central Asia after the consumption of uncooked meat from camels, goats, and other ungulates, which are also susceptible to infection.32
In the United States, plague is endemic in the 17 contiguous western states extending from the Pacific Coast to the Great Plains. A total of 437 cases in humans were reported during 1970 through 2010 (∼10 cases per year), with 60 deaths (14% mortality). Approximately 80% of human cases occur in New Mexico, Arizona, and Colorado and 10% in California (Fig. 231-3).33 Human plague is most common during May through October when fleas are active; wintertime cases are rare and often associated with hunting. Males are slightly more likely to be infected, and more than half of cases occur in persons younger than 20 years of age. Incidence is highest among Native Americans and Hispanics, although non-Hispanic whites account for the majority of cases.34 Within endemic areas, elevated plague risk is associated with close contact with rodents and their feline and canine predators, the presence of harborage and food sources for wild rodents in the vicinity of homes, and possibly a failure to control fleas on pet dogs and cats.31,35 Expanding suburban development in prime plague habitat has led to increasing cases in some areas and a shift toward higher socioeconomic groups in recent years.36 Travelers with plague can present a diagnostic challenge when encountered outside endemic areas (peripatetic plague) and may trigger concern about possible terrorist exposures.37 Recent instructive cases include the death of a wildlife biologist from primary pneumonic plague after necropsying an infected mountain lion38 and the occurrence in a laboratory researcher with occult hemochromatosis who died of sepsis caused by an attenuated laboratory strain that lacked virulence genes necessary for iron absorption.39,40
Pathogenesis
Fleas become infected when feeding on a bacteremic host. In the cooler environment of the flea, the bacillus expresses a variety of factors that facilitate colonization of the flea midgut, replication, and blockage of the flea intestine (see Table 231-1).19 Starved of sustenance, “blocked” fleas feed aggressively, regurgitating bacteria into the bite wound at each attempt. Many of the inoculated bacteria are likely phagocytized and killed by polymorphonuclear lymphocytes; however, a few are taken up by mononuclear cells unable to kill them and carried via lymphatics to the regional lymph nodes.17 Growing at 37° C, the bacteria begin expressing F1 envelope antigen, enhancing their ability to resist subsequent phagocytosis by polymorphonuclear lymphocytes.2,17 Within the lymph node, the bacilli stimulate an intense inflammatory response that is detectable clinically as a bubo. Microscopic examination of the fully developed bubo reveals invasion by polymorphonuclear leukocytes, hemorrhagic necrosis with destruction of normal architecture, and dense concentrations of extracellular bacilli. Bacteremia is common and, in the absence of specific therapy, can lead to secondary pneumonia, disseminated intravascular coagulation, acute renal failure, and irreversible shock.41 Blockage of vessels in cooler acral sites, including fingers, toes, ears, and nose, can lead to gangrene (Fig. 231-4), a startling feature that may have given rise to the name “Black Death.”42
Clinical Manifestations
Plague takes several different clinical forms depending in part on the route of exposure. In the United States, 80% to 85% of patients present with primary bubonic plague, 15% with septicemic plague, and 1% to 3% with pneumonic or other forms of plague. The usual incubation period is 2 to 7 days but can be as short as 1 day for patients with primary pneumonic plague.
Bubonic Plague
Bubonic plague results from cutaneous exposure and is characterized by the sudden onset of high fever, chills, weakness, and headache. A bubo or swelling of regional lymph nodes becomes apparent in the groin, axilla, or neck within the first day. Buboes vary from 1 to 10 cm and elevate the overlying skin, which may be warm and erythematous. Palpation typically elicits extreme tenderness. Bubonic plague is distinguished from other forms of lymphadenitis by its sudden onset, the intensity of inflammation in the bubo, and the usual absence of obvious skin lesions or associated ascending lymphangitis (Fig. 231-5). Nevertheless, careful examination distal to the bubo will occasionally reveal a small papule or scab demarcating the site of the flea bite.41,43 Rarely, ulcers or eschars may develop that can be confused with those of anthrax or tularemia (Fig. 231-6). The differential diagnostic possibilities for bubonic plague include cat-scratch disease, staphylococcal or streptococcal lymphadenitis, tularemia, filarial lymphadenitis, chancroid and other sexually transmitted diseases, and strangulated hernia.
Septicemic Plague
Septicemic plague is characterized by the sudden onset of high fever without associated bubo or other obvious localizing signs. Illness is rapidly progressive, leading to overwhelming sepsis and organ failure within a few days. Typically, the diagnosis is not considered until Y. pestis is isolated from blood cultures. Some patients have gastrointestinal symptoms of nausea, vomiting, diarrhea, or abdominal pain, which may further complicate the diagnostic workup.44 Because of delays in diagnosis and treatment, the case-fatality rate for septicemic plague in the United States is approximately 28%, three times higher than for bubonic plague.45,46
Pneumonic Plague
Pneumonic plague occurs in two forms, secondary and primary, both of which are frequently fatal and potentially contagious to close contacts.47,48 Secondary pneumonic plague is the more common form and arises through hematogenous spread of bacteria from a bubo or other source. Approximately 10% of all plague patients in the United States develop secondary pneumonic plague, usually as a result of delayed treatment of bubonic infections. Secondary pneumonic plague begins as an interstitial process with cough productive of scant, tenacious sputum, typically beginning 5 to 6 days after illness onset. Chest radiographs reveal diffuse alveolar infiltrates that are almost always bilateral and often accompanied by pleural effusions.49 If untreated, sputum will become more copious and eventually bloody, and death will often occur within 3 to 4 days.
Primary pneumonic plague is a fulminant condition that results from direct inhalation of bacteria into the lungs. This can occur through contact with another patient with pneumonic plague, exposure to animals (especially cats) with respiratory or pharyngeal plague, laboratory exposures, or, potentially, as a result of intentional aerosol release for purposes of terrorism. Symptoms begin within 1 to 4 days after exposure. Patients experience sudden onset of fever, chills, headache, malaise, and rapidly advancing tachypnea, dyspnea, hypoxia, chest pain, cough, hemoptysis, and general signs of endotoxemia. Chest radiographs demonstrate a lobar pneumonia initially (Fig. 231-7), followed by dense consolidation and bronchopneumonic spread to other lobes of the same or opposite lung.30,50 Sputum is often purulent but may be watery, frothy, and copious and also may be blood tinged or grossly hemorrhagic, at which point it may contain large numbers of plague bacilli.47 Histologically, numerous bacilli and inflammatory cells fill the alveolar space (Fig. 231-8). Untreated pneumonic plague is almost always fatal, and mortality is very high in persons whose treatment is delayed beyond 24 hours after symptom onset. The case-fatality rate for pneumonic plague in the United States since 1950 approaches 50%.
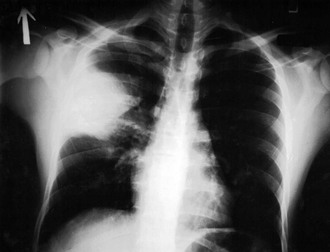
To prevent person-to-person transmission, patients with suspected pneumonic plague should be managed in isolation under respiratory droplet precautions.12,51 Strict isolation under negative pressure and filtered exhausting is not necessary because infectious dispersal by fine aerosol or dried droplet nuclei does not occur. Person-to-person transmission of pneumonic plague requires close contact, typically with a patient who is in the late stages of infection and coughing copious amounts of bloody sputum.52 The last case of person-to-person respiratory spread in the United States occurred in Los Angeles in 1924. There have been at least nine subsequent cases of primary pneumonic plague, two resulting from laboratory exposures and others from exposures to domestic cats with respiratory plague, none of which resulted in transmission to other persons.30
Other Syndromes
Plague meningitis is a rare complication that can occur acutely or as a delayed manifestation of inadequately treated bubonic plague. Symptoms include fever, headache, sensorial changes, and meningismus. Examination of cerebrospinal fluid reveals a pleocytosis with predominance of polymorphonuclear leukocytes. Bacteria are frequently demonstrable with a Gram or Wayson stain of cerebrospinal fluid sediment. Another rare presentation is pharyngeal plague, which resembles acute tonsillitis. The anterior cervical lymph nodes are usually inflamed, and Y. pestis may be recovered from a throat culture or by aspiration of a cervical bubo. Asymptomatic pharyngeal colonization with Y. pestis has also been reported among close contacts of patients with pneumonic plague.
Laboratory Findings
Laboratory findings in patients with plague are similar to those of patients with other serious gram-negative infections. Peripheral white blood cell counts generally range from 10,000 to 25,000 cells/mm3 with a predominance of immature neutrophils. Leukemoid reactions with counts as high as 50,000/mm3 or greater can occur. Platelet counts may be normal or low in the early stages of bubonic plague. Bacteremia is common; in one series, single blood cultures obtained at the time of hospital admission were positive in 27% of cases.43 Without prompt treatment, patients develop such high-level bacteremia that bipolar staining bacilli can be seen in the peripheral blood smear, a finding that suggests the diagnosis and is associated with a poor outcome (Fig. 231-9). Disseminated intravascular coagulation, thrombocytopenia, elevated results of liver function tests, and impaired renal function are all common in advanced stages of illness.43
Diagnosis
Plague should be considered in any patient with an acute febrile illness and a risk for exposure to infected animals or fleas in a plague-endemic area. A careful history and thorough physical examination are required to make a timely diagnosis; a delayed or missed diagnosis of plague is associated with a high case-fatality rate. In the United States, the Laboratory Response Network (LRN) provides upgraded, standardized diagnostic testing for Y. pestis and other select agents.13,53 All state public health laboratories have the ability to conduct rapid and confirmatory testing or, if necessary, forward materials to the CDC for confirmation and advanced procedures, including molecular subtyping.54
When plague is suspected, diagnostic specimens should be obtained promptly and effective antimicrobial therapy initiated immediately thereafter.12 Chest radiographs should be obtained to rule out pneumonia. Appropriate diagnostic specimens include blood cultures and bubo aspirates, sputum, tracheobronchial washes, swabs of skin lesions or pharyngeal mucosa, and cerebrospinal fluid, as indicated by signs and symptoms. Bubo aspirates are especially useful and can be obtained by inserting a 20-gauge needle on a 10-mL syringe containing 2 mL of sterile saline solution into the bubo and withdrawing the plunger several times until the saline becomes blood tinged.
Primary specimens can be stained with Wayson or Giemsa stain, and with Gram stain, and examined using light microscopy. In the case of pneumonic plague, stain and culture of bronchial washings or expectorated sputum may yield a putative diagnosis quickly. With Wayson staining, Y. pestis appears as light blue bacilli with dark blue polar bodies, giving the organisms a closed safety-pin appearance that is characteristic of but not pathognomonic for Y. pestis. If possible, the specimens should also be examined using direct fluorescent antibody (DFA) testing. Presumptive identification of Y. pestis can be made by polymerase chain reaction assay.15,54,55
Laboratory confirmation is best achieved through the isolation of Y. pestis from body fluids or tissues. Samples should be inoculated onto suitable culture media (e.g., brain-heart infusion broth, sheep blood agar, chocolate agar, or MacConkey agar) and held for 5 to 7 days.15 Plague bacilli are readily distinguished from other gram-negative bacteria by staining properties, growth characteristics, and biochemical profiles. Nevertheless, automated identification systems frequently misidentify Y. pestis as other species, an error that frequently delays diagnosis.56 Isolates are confirmed in reference laboratories by lysis with Y. pestis–specific bacteriophage.
In patients with negative cultures, plague can be confirmed serologically by passive hemagglutination testing for antibodies to Y. pestis F1 antigen. A fourfold or greater change in titer between an acute serum and a convalescent serum collected 3 to 4 weeks later is considered diagnostic, as is a single titer greater than or equal to 1 : 128 in an unvaccinated patient with compatible illness.15 A small percentage of plague patients will develop diagnostic antibody levels within 5 days after illness onset, most seroconvert within 1 to 2 weeks, a few seroconvert more than 3 weeks after onset, and fewer than 5% fail to seroconvert.57 A rapid, handheld chromatographic assay designed to detect Y. pestis F1 antigen in patient samples is being evaluated for rapid presumptive diagnosis at the bedside.58
For diagnosis in fatal cases, samples of lymph nodes, liver, spleen, lungs, and bone marrow should be collected at autopsy for culture, DFA antibody testing, and histologic studies, including immunohistochemical staining. Cary-Blair or a similar holding medium can be used to transport swabs or tissues for culture.
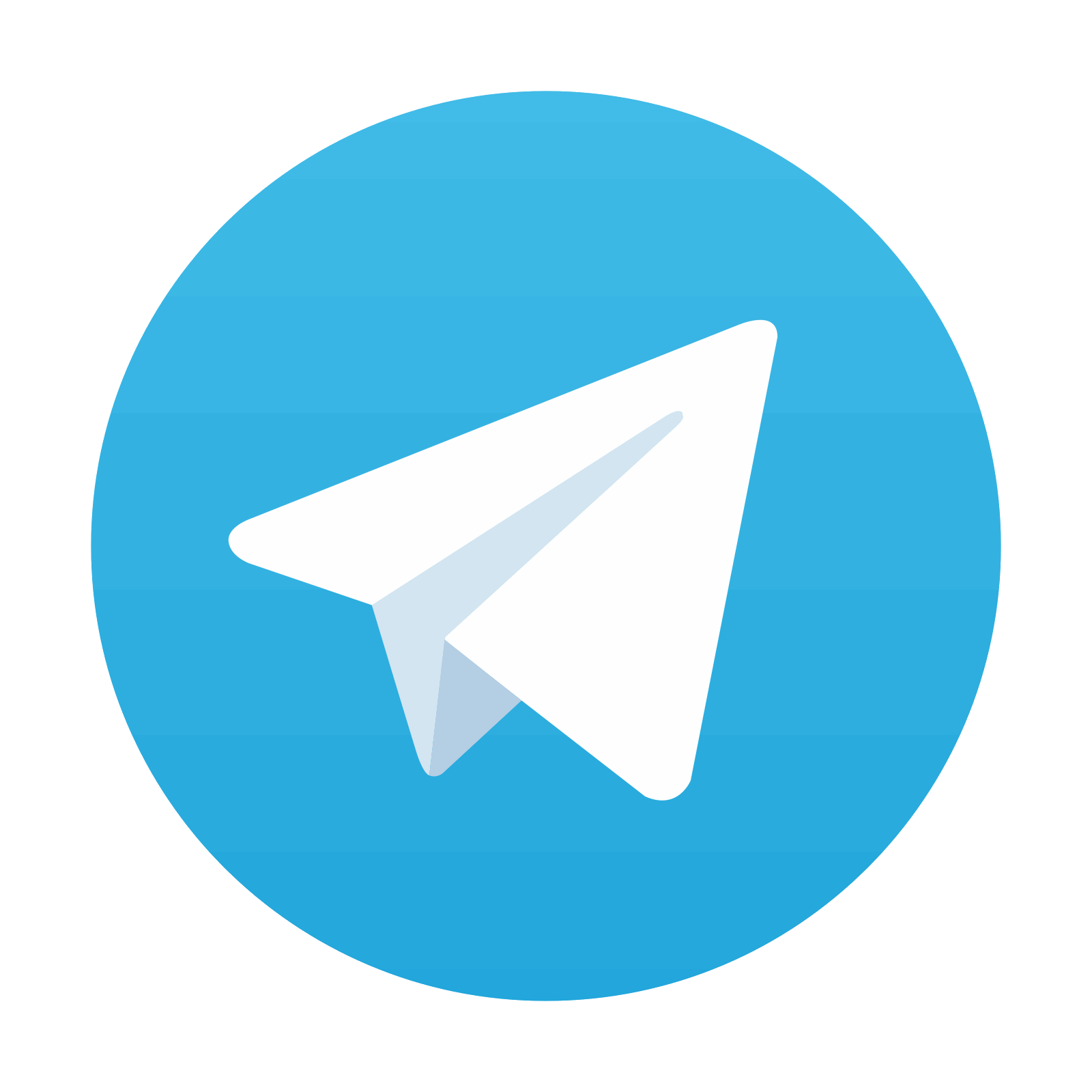
Stay updated, free articles. Join our Telegram channel

Full access? Get Clinical Tree
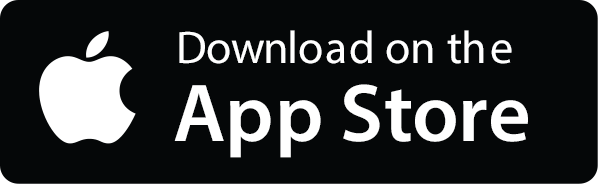
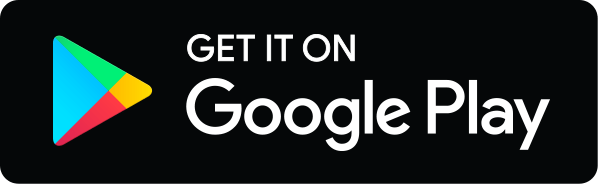