The Immune System and Host Defense Against Infections
Joseph B. Margolick
Richard B. Markham
Alan L. Scott
INTRODUCTION
The human immune system comprises a diverse array of cells found throughout the human body (Box 10-1) that protect it against the pathogenic effects of infectious organisms that may enter and threaten the body. The goal of this chapter is to describe (at a level appropriate for a non-immunologist) how this protection is provided. Astonishing insights into immunity have been realized in the last few decades, more than can be covered in an introductory overview chapter. Additional information is provided in feature boxes scattered throughout this chapter, along with referrals to other sources for those who may want to learn more detail. The last 30 years have been an exciting and challenging time for both immunology and infectious disease epidemiology. In this chapter, you will encounter the best of both worlds.
Box 10-1 Cells of the Immune System
Cells of the immune system include lymphocytes, mononuclear phagocytes, and dendritic cells. These cells are derived from bone marrow precursors that circulate throughout the body in the bloodstream and populate the lymphoid organs, where they mature as described later in this chapter.
In the peripheral blood, approximately one-fourth of the white blood cells are lymphocytes and approximately one-twentieth are monocytes, the circulating form of mononuclear phagocytes. Less than 1% are dendritic cells. At any given time, only 2% of lymphocytes are found in the peripheral blood. The rest are present in the lymphoid tissues such as lymph nodes and collections of lymphoid aggregates that are found below mucosal surfaces in the body, such as gut-associated lymphoid tissue (GALT).
Research on immune functions uses many experimental methods, both in vivo and in vitro. In many cases, immunogenic cells perform the same functions in tissue culture, where they are relatively easy to measure, as they do in the body. Because these functions are generally similar across the immune systems of humans and of other animals, inferences from animal models of disease often have direct applicability to human host defense and immune function. Moreover, the advent of modern molecular microbiology and recombinant DNA methods has made it possible to isolate and characterize many of the molecules produced by immune cells that regulate and/or mediate the functions of the immune system, and also to gain insight into the functions of these molecules in animal models by inserting or deleting (“knocking out”) the genes that code for these molecules. These studies have revealed a complex and dynamic interaction between cells of the immune system and pathogens.
The immune system is traditionally considered to have three defining characteristics. First, it can discriminate between self and non-self—that is, what is normally present in the host and what is not. Second, it remembers what it has encountered (memory). Memory allows the immune system to react more quickly and effectively to a stimulus it has encountered previously. Third, it responds only to the pathogen that is at hand (specificity). In this chapter, we also explain how the immune system attains these characteristics.
Two distinct but interrelated arms of the immune system—one general and one highly specific—have evolved for the recognition of pathogens and foreign molecules. Both arms involve a complex of cell surface receptors and soluble molecules that work in concert to identify a pathogen through unique aspects of its molecular makeup, and tag it for elimination.
The first arm is designed to work within minutes after a pathogen establishes residency in the vertebrate host. It utilizes receptors that are constitutively expressed on mononuclear and polymorphonuclear phagocytic cells and on “killer” cells that recognize pathogen-associated molecular patterns (PAMPs). These receptors provide a general signal that certain types of microorganisms are present, and they initiate cellular mechanisms that can clear the foreign pathogen from the body. This ready-to-use capacity to rapidly recognize pathogens is referred to as the innate immune response. The term “innate” refers to the fact that these responses do not require time to develop but rather are ready to go at any time. The main cell types involved in innate immunity are macrophages, dendritic cells, and natural killer (NK) cells (Box 10-2 and Box 10-3). Epithelial cells also play a role in the innate immune response.
Most encounters with microorganisms and toxins are not obvious to the person who experiences them because the innate immune response is able to eliminate the threat of infection. However, in those situations where cells and molecules of the innate immune response fail to control and eliminate a pathogenic organism, the role of the innate response shifts to one of initiating, modulating, and mediating the second, highly specific arm of the vertebrate defense system—the adaptive immune response. The term “adaptive” refers to the fact that these responses take time to develop and are modified over this time to best respond to the specific infection. The cells that regulate and carry out most of the major effector functions of the adaptive immune response are lymphocytes called T cells and B cells. These lymphocytes express cell surface antigen-specific receptors that confer the inducibility and specificity that are the hallmarks of the adaptive immune response. In addition, after clearing the pathogen, the cells of the adaptive immune response develop a long-lived “memory” of the exposure that can be quickly mobilized upon re-exposure to the same antigens.
Box 10-2 Macrophages and Dendritic Cells
Mononuclear phagocytes circulate in the peripheral blood as monocytes, and migrate into tissues where they become macrophages. Their functions are to ingest and eliminate infectious agents, process and present antigens, and regulate the functions of other immune cells, both innate and adaptive.
In tissues, dendritic cells have a characteristic shape with many long cytoplasmic processes (dendrites). They travel through the peripheral blood to tissues, where they persist in an immature form. When stimulated to mature by infection or injury, they become avid inducers of innate immunity. The mature cells also migrate to lymphoid organs carrying antigens bound to their cell surface, and function as potent antigen-presenting cells. Uniquely, these cells can induce a primary immune response. They also affect the cytokine secretion pattern of the antigen-activated T cells.
Box 10-3 Types and Names of Lymphocytes
Three types of lymphocytes are found in the peripheral blood: T cells, B cells, and natural killer (NK) cells. These cells are morphologically indistinguishable by conventional microscopic techniques, although NK cells are often a bit larger and have more granules in their cytoplasm than either T or B cells.
T and B cells are named after the organs that are necessary for their maturation. T cells mature in the thymus, a gland located underneath the breastbone or sternum, in mammals and rodents. B cells received their name from the bursa of Fabricius, an organ found in birds that is essential for B-cell maturation. Humans do not have this organ, so B cells mature in the bone marrow in humans. NK cells are described in Box 10-8.
Lymphocyte biology is a relatively new field (at least relative to the ages of the authors). Only in 1960 was it discovered (by Peter Nowell) that resting lymphocytes could be triggered to become activated, and only in 1964 was it discovered (by John Gowans) that lymphocytes recirculated in the body from blood to lymph and back to blood, even though recirculation of red cells had been demonstrated in 1628 by William Harvey.
In the following review, we explore the cells and molecules that play key roles in the functions of both the innate and adaptive immune responses. The main cells involved in adaptive immunity are lymphocytes and macrophages (see Box 10-3).
Pathogens that reside inside cells pose a special challenge to the immune system, because they are not directly accessible to detection. However, cells containing pathogens are themselves altered at the cell surface, and these changes can be recognized by both the innate and the adaptive immune systems. As mentioned earlier, the innate immune system can recognize molecular patterns present on the pathogen itself. It can also recognize patterns on the surface of infected cells, which may be genetically altered as a result of being infected. For example, infection of a cell may cause a normal surface molecule to be expressed at abnormally high or low levels. This is yet another example of how innate immunity does not depend on the identity of the infecting pathogen. (Innate immunity is also triggered by non-infectious processes that affect the integrity of cells, such as heat injury, radiation, toxic exposures, or, in some cases, neoplastic transformation.)
The first step in the immune response to a pathogen is the recognition of the pathogen. It has long been known that the immune system can distinguish self from non-self or foreign antigens. How this was accomplished remained a fascinating mystery for many years, but the essential mechanisms have now been clarified, in work that resulted in at least seven Nobel Prizes.
RECOGNITION OF PATHOGENS
What does the immune system actually recognize, or react to? Substances that can trigger an immune response are called antigens. More specifically, the receptors on cells of the immune response recognize small sub-regions on each antigen, termed epitopes or antigenic determinants. A single antigen molecule can have many epitopes that can be recognized by different receptors. Epitopes can be made up of amino acids, sugars, lipids, or nucleotides. In adaptive immunity, lymphocyte receptors recognize highly unique epitopes on pathogen-derived antigens. Those antigens that are recognized during this response are not normally present in the body, because they are derived from particular molecules present in bacteria, viruses, parasites, or other organisms. In contrast, the receptors used in the innate immune response recognize pathogen-derived antigens that are not species-specific, but rather are representative of a class of microorganism, such as virus, bacteria, fungi, or parasite.
In recent years, much work has been directed at defining the precise chemical nature of antigens, including which characteristics an antigen must have to elicit an effective immune response. This work has been motivated by very practical concerns, such as the need to develop vaccines and understand immune responses to dangerous organisms.
Antigen Recognition in Adaptive Immunity: T and B Cells
The cells that are responsible for specific recognition of foreign antigens (i.e., adaptive immunity) are B and T lymphocytes (see Box 10-3). These cells have surface proteins that bind to (recognize) antigens with high specificity and affinity: each particular surface protein can bind effectively to one and only one antigen. These surface recognition proteins are called antigen receptors, and it is the precision of these receptors (they will bind to only one epitope out of all possible epitopes) that is responsible for the amazing specificity of the immune system. (Box 10-4). For T cells, the epitope is usually a small peptide; for B cells, it is frequently more than a small peptide that is recognized. When the antigen receptor binds its antigen, the B or T cell becomes activated and the immune response is initiated.
Box 10-4 Specificity of Antibodies
For many years, the specificity of antibodies produced by immunizing experimental animals has been exploited to identify substances in experiments and in clinical medicine. This has been particularly true since the development of methods for making monoclonal antibodies to any desired antigen. Monoclonal antibodies are derived from a single B cell and, therefore, cannot be contaminated by antibodies with other specificities.
Both B and T cells can recognize any antigen that might possibly be encountered, including synthetic antigens that do not exist in nature. How such a vast array of receptors could exist was a puzzle to immunologists during the many years when it was believed that each unique antibody molecule was encoded by its own gene, because there is not enough DNA in the entire body to code for one gene for each possible antibody. The key to this diversity was determined first for B cells (Figure 10-1), and subsequently shown to apply to T cells (Figure 10-2) as well. One section of the receptor protein consists of relatively constant amino acid sequences that are shared by many receptors and coded for by a small number of genes. The second section is a highly variable part of the receptor
that recognizes the vast diversity of antigens which is created by allowing rearrangement of gene segments to generate a huge number of permutations (Box 10-5). The genes coding for the constant and variable segments are assembled within a given B or T cell into a single DNA sequence that codes for the final receptor molecule that will be expressed by that cell (see Box 10-7 for a more detailed description of this process).
that recognizes the vast diversity of antigens which is created by allowing rearrangement of gene segments to generate a huge number of permutations (Box 10-5). The genes coding for the constant and variable segments are assembled within a given B or T cell into a single DNA sequence that codes for the final receptor molecule that will be expressed by that cell (see Box 10-7 for a more detailed description of this process).
Although generated by similar mechanisms, Band T-cell antigen receptors work in different ways. The B-cell receptor recognizes antigens in their native form—that is, as they exist in nature. As a consequence, the antigen does not need to be manipulated in any way, and the B cell can recognize the antigen by itself. This is true whether the B-cell receptor, which is an antibody molecule, is attached to the surface membrane of the B cell, or has been secreted from the cell and is free of the B cell entirely.
The T-cell antigen receptor differs from the B cell antigen receptor in two ways. First, it cannot recognize native antigens. Instead, it recognizes only antigens that have been broken down into short, epitope-sized peptide fragments. This process, which is referred to as antigen processing, can occur within the cytoplasm of many types of cells, called antigen-presenting cells. The processed antigen is then carried to the surface of the antigen-presenting cell by major histocompatibility(MHC) proteins. (Box 10-6) This leads to the second major difference between B- and T-cell recognition of antigens: the T-cell receptor binds not only to the processed peptide, but also to the carrier (MHC) protein on the surface of the antigen-presenting cell. This process of re-expressing the processed antigen on the surface of the antigen-presenting cell is called antigen presentation. In other words, the T-cell antigen receptor, even though it is specific for one peptide, is also specific for one MHC protein, and T-cells recognize an antigen only if it is bound to the correct MHC protein. (Figure 10-3). For this reason, antigen recognition by T cells is said to be MHC-restricted, and antigen-presenting cells and T cells must be histocompatible for T-cell activation to occur. Because of MHCrestriction, one person’s T cells will not recognize any antigens unless they are presented by that person’s own antigen-presenting cells (or antigen-presenting cells from another person who happens to have some of the same particular MHC proteins). In summary, T-cell responses require the processing and presentation of antigens by an antigen-presenting cell to a histocompatible T cell.
Box 10-5 Generation of Receptor Diversity
The receptors for both T and B cells have a number of functional domains or regions. A large proportion of both of these receptors have common functions, such as anchoring the receptor to the cell membrane and binding to a number of accessory proteins both at the cell surface (to stabilize cell-cell interactions) and in the cytoplasm (where they act as a binding point for the proteins that participate in the transduction of signals from the cell membrane to the nucleus that result in cell activation and differentiation). These regions of the receptors are, therefore, termed constant regions.
Both T- and B-cell receptors have a domain that is specifically responsible for binding to a small portion of an antigen (i.e., an epitope). Some aspects of these receptors are illustrated in Figures 10-1, 10-2, and 10-3. This antigen-binding site is unique for each different B-cell receptor (i.e., antibody) or T-cell receptor and is the source of antigen specificity for a B cell or a T cell, respectively. This part of the receptor is, therefore, called the variable region.
The vast number of unique specificities used by the receptors of the adaptive immune response are generated by a random combinatorial mechanism that is independent of antigens. T-cell and B-cell receptors are composed of multiple protein chains. (See Figures 10-1, 10-2, and 10-3.) Each chain is encoded by a number of gene segments that are spliced together at the DNA level within a given T or B cell to form a complete gene for a specific receptor. A large number of germline gene segments encode the domains responsible for antigen recognition. These segments are assembled in a random fashion that, along with random insertions and deletions of nucleotides at splicing junctions, determines the final amino acid sequence of this variable region of the receptor protein, which in turn determines the antigen specificity of each receptor chain. Following molecular events that assemble the genes for the variable region of the receptor with those for the constant regions, the multiple protein chains are manufactured by the cell and joined to make the mature receptor. The interactions among the variable regions of these multichain molecules then determine the fine specificity of each receptor.
Current estimates of the number of different T-cell and B-cell receptors that are generated by the random use of multiple germline variable genes, imprecise joining of gene segments, and random use of different chains generated approach 1018! This vast array of receptor molecules accounts for the astounding ability of the immune system to recognize any possible antigen.
Box 10-6 General Function of MHC Proteins
MHC proteins are part of a general protein-trafficking system within the cell. Intracellular proteins are continually being broken down into small peptides, and those peptides are taken up by MHC proteins within the cell and carried to the cell’s surface. If a peptide being carried by the MHC protein is derived from a normal cellular protein, it does not elicit an immune response from T cells. Conversely, if the peptide is derived from a pathogen (or other foreign substance), T cells that recognize it (along with the MHC protein) are triggered and an immune response is initiated. Moreover, if a T cell were to encounter an MHC molecule from a different individual carrying a peptide from that individual, the foreign MHC-peptide combination would also be recognized, triggering an immune response. This latter circumstance arises when tissues from an individual of one MHC type are transplanted into an individual with another MHC type. Thus, when a T cell engages the MHC-peptide complex, it will initiate an immune response if either the peptide or the MHC molecule is derived from a “foreign” source.
MHC proteins are highly variable from person to person, and, in fact, are among the most variable proteins known (Box 10-7). It is this enormous variability from person to person that allows the immune system to distinguish self from non-self—that is, to differentiate between one’s own cells and antigens and someone else’s. Two broad classes of MHC molecules exist: Class I molecules, which are expressed on all nucleated cells in the body, and Class II molecules, which are primarily expressed by cells of the immune system (monocytes/macrophages, dendritic cells, B cells, and activated T cells). In humans, MHC proteins are also called human leukocyte antigens (HLA), and we refer to a person’s “HLA type.” MHC Class I proteins are the primary antigens responsible for graft rejection and must be expressed by a target cell for this cell to be killed by an antigen-specific CD8 T cell.1 MHC Class II must be the same on the antigen-presenting cell and the CD4 T cells for the latter to be triggered.2 In fact, all of the cells listed previously as expressing Class II MHC molecules are important antigen-presenting cells, even B cells. Of note, activated B cells can use their antigen-specific surface receptor to facilitate antigen uptake as the first step in antigen processing. For this reason, B cells can process antigens that are present in very low concentrations, and they are important cells for presentation of antigens to T cells during mature immune responses.3 However, B cells specific for a given antigen are much less numerous than other antigenpresenting cells, which explains why the non-specific antigen-presenting cells are essential.
Because they vary so widely among individuals, the MHC proteins from different people will interact differently with the foreign proteins or peptides encountered by those individuals. Most of the antigens
associated with pathogens are complex molecules that can be degraded by antigen-processing cells into many different peptides. Among individuals with different MHC proteins (i.e., different HLA types), therefore, different peptides may bind most efficiently to a given individual’s repertoire of MHC proteins. For example, if a protein contains epitopes A and B, and individuals X and Y have different HLA types, then only epitope A may be presented by antigen-presenting cells in individual X and only epitope B may be presented by the corresponding cells in individual Y. Some individuals may have an MHC type that will not bind either of these epitopes; these individuals will not recognize this protein at all. This point means that immunizing a human population against a T-cell epitope (i.e., eliciting a cellular immune response at a population level) is much more difficult than eliciting a B-cell response, because B cells recognize native antigens, which are the same for everybody. Given the huge diversity of MHC proteins in the human population, development of vaccines that will induce general T-cell immunity represents an extremely formidable challenge. It is not a coincidence that almost all successful vaccines generated to date have depended largely on B-cell responses (i.e., antibodies) rather than T-cell responses.
associated with pathogens are complex molecules that can be degraded by antigen-processing cells into many different peptides. Among individuals with different MHC proteins (i.e., different HLA types), therefore, different peptides may bind most efficiently to a given individual’s repertoire of MHC proteins. For example, if a protein contains epitopes A and B, and individuals X and Y have different HLA types, then only epitope A may be presented by antigen-presenting cells in individual X and only epitope B may be presented by the corresponding cells in individual Y. Some individuals may have an MHC type that will not bind either of these epitopes; these individuals will not recognize this protein at all. This point means that immunizing a human population against a T-cell epitope (i.e., eliciting a cellular immune response at a population level) is much more difficult than eliciting a B-cell response, because B cells recognize native antigens, which are the same for everybody. Given the huge diversity of MHC proteins in the human population, development of vaccines that will induce general T-cell immunity represents an extremely formidable challenge. It is not a coincidence that almost all successful vaccines generated to date have depended largely on B-cell responses (i.e., antibodies) rather than T-cell responses.
Box 10-7 Diversity of MHC Proteins
In humans, more than 2100 distinct MHC allele sequences have been described. Human Class I MHC proteins include HLA-A, HLA-B, and HLA-C proteins, which have 319, 609, and 161 known variants, respectively. Class II MHC proteins include HLA-DR, HLA-DQ, and HLA-DP; these have 406, 72, and 120 known variants, respectively. The number of distinct protein molecules that can be generated is not precisely known, but is certainly very large.
MHC proteins contain indentations or clefts that can accommodate small peptides (9-15 amino acids long). The characteristics of the amino acids surrounding the MHC cleft determine the peptide that can become bound in the cleft and, therefore, is presented to T cells.
Antigen Recognition in Innate Immunity
A third type of lymphocyte, termed natural killer (NK) cells, plays a key role in triggering innate immunity (Box 10-8).
The mechanism by which NK cells are triggered to kill has only recently been elucidated. Although they do not have antigen receptors, NK cells have surface receptors that inhibit their killing function. These inhibitory receptors, termed killer inhibitory receptors (KIRs), recognize abnormal levels of MHC Class I molecules on all other nucleated cells in the body. Thus, if the target cell expresses MHC Class I to a normal degree, the NK cell is not triggered and the target cell is not killed. Conversely, down-regulation of MHC Class I molecule expression (which is common in some virally-infected cells and some tumor cells) removes the inhibition of the NK cell, so that the target cell is killed.
Box 10-8 History of Natural Killer Cells
NK cells were discovered to be the cells responsible for the background killing that was detectable when peripheral blood cells were tested for cytotoxicity against various target cells. Researchers found that there was usually some level of cytotoxicity in such assays that did not depend on the presence of antigen in the assay, did not require priming of the cells, and was not mediated by the cytotoxic lymphocytes that were known at the time (i.e., the cytotoxic T cells). The cytotoxic cells turned out to be large granular lymphocytes. They were named natural killer (NK) cells because they did not need to be induced (primed) by in vitro treatments of the cells.
This mechanism appears to be an important component of host defense against certain viral infections.4, 5 Many viruses interfere with expression of MHC molecules by the host cell (possibly as a means of evading cytotoxic CD8+ T-cells, which require MHC Class I molecules to be expressed on the target cell, as discussed previously). Thus, NK cells help prevent the virus from getting away with this trick. This mechanism elegantly explains the function of NK cells.
After this understanding was reached, in somewhat of a surprise, stimulatory NK receptors were discovered by researchers. The function of NK cells is regulated by the balance of signals coming from the KIR and the stimulatory receptors. It has been hypothesized that stimulatory receptors on NK cells may be important in situations in which MHC proteins are over-expressed, which is uncommon in infectious diseases but may occur in neoplastic or pre-neoplastic conditions.
Macrophages and dendritic cells have been described in Box 10-2. How do they recognize antigens? In contrast to the specific recognition of particular antigens by T-cells, as described previously, macrophages and dendritic cells recognize molecules common to multiple pathogens, or structures derived from such antigens. For this reason, antigen receptors on these cells are referred to as pattern recognition receptors (Box 10-9).6
Box 10-9 Examples of Pattern Recognition in Innate Immunity
Examples of molecules that are recognized by their pattern are certain carbohydrates and lipopolysaccharides, including endotoxins. Another example is nonmethylated GpC motifs in bacterial DNA; these motifs are not present in mammalian DNA. These patterns are recognized by a family of receptors called toll-like receptors, so named because they resemble a type of receptor found in fruit flies called a toll receptor.8, 9
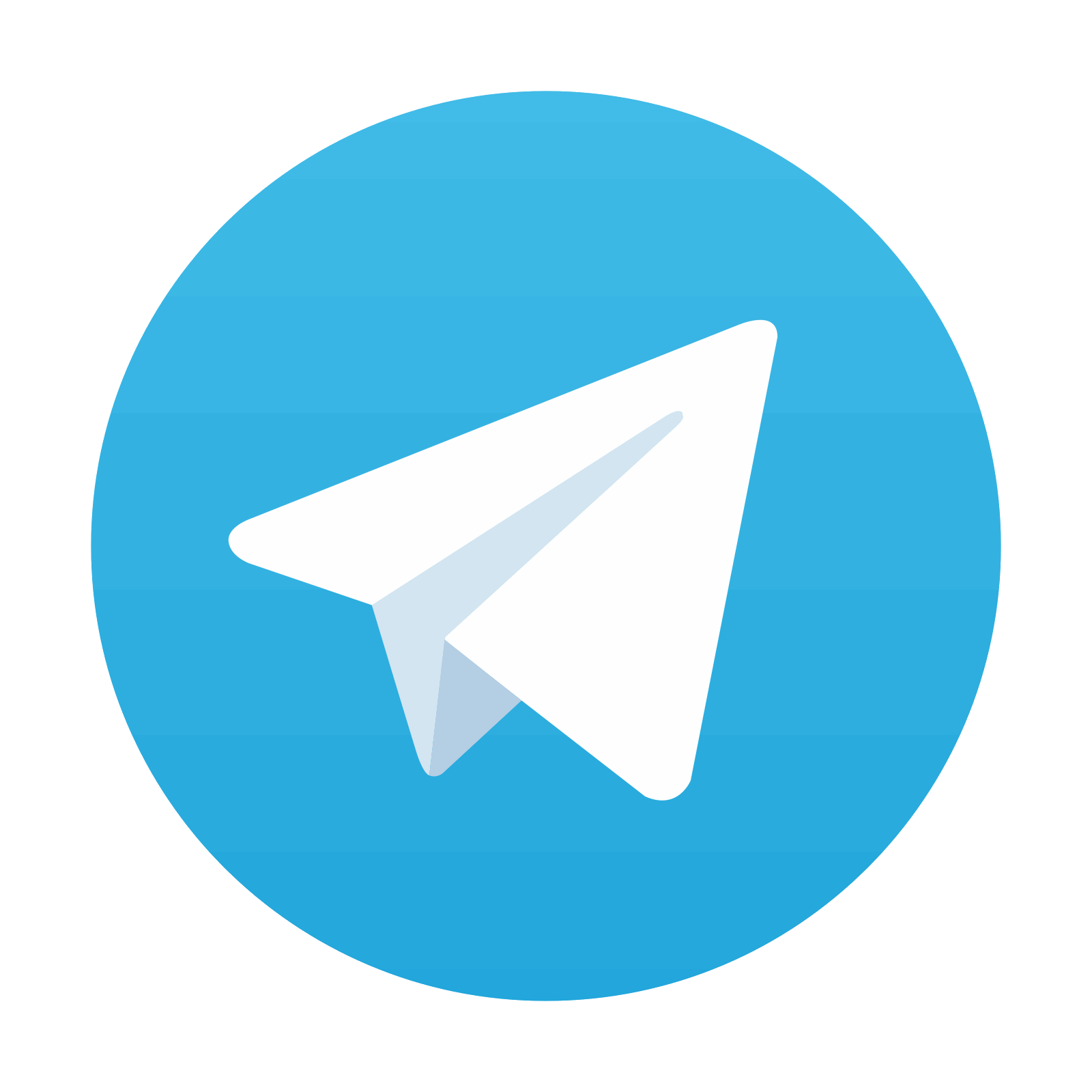
Stay updated, free articles. Join our Telegram channel

Full access? Get Clinical Tree
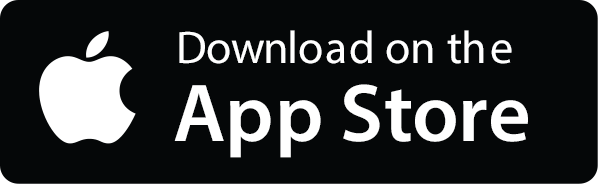
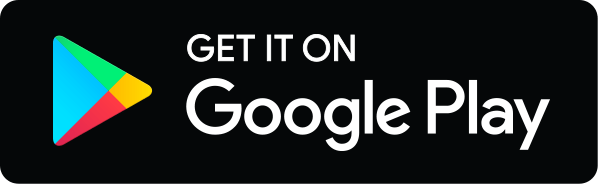
