Key points
- •
Prostate interventions can be used to both diagnose and treat prostate cancer.
- •
Although none have been shown to affect outcomes, and the data regarding side effects is heterogeneous, most show low rates of incontinence and impotence.
- •
Patient and treatment modality selection is a complex consideration, with diagnostic imaging providing an important component.
- •
Some treatments are not widely available or approved for use in all countries.
- •
In focal therapy, follow-up is paramount, as the entire prostate (and, in some cases, low-volume and low-grade disease) is not treated.
Introduction
Prostate cancer is the most common noncutaneous cancer and the second leading cause of cancer death in American men. Approximately one out of every six men in the United States will be diagnosed with prostate cancer in his lifetime, but only one in six of them will die of it. More than 2 million men diagnosed with prostate cancer were living in 2010, and the medical costs associated with prostate cancer for 2010 are estimated at $12 billion. This relatively low death rate sets prostate cancer apart from many other cancers. Complicating this phenomenon is increasing interest in “active surveillance” of prostate cancer, the idea that small-volume, low-grade disease can be safely observed with intervention reserved for the identification of progression of disease. ,
The goal of treatment is to both discriminate cancer that should be treated from that for which treatment can be safely deferred, and then to minimize the adverse effects of treatment while still achieving cure. This is complicated by the fact that the nerves involved in potency and continence lie alongside the prostate. A New England Journal of Medicine article reporting on 1201 men from multiple sites found incontinence rates of up to 20% for surgery and erectile dysfunction in up to 60% for radiation therapy at 2 years. Yet, the majority of prostate cancer is organ-confined at the time of diagnosis with current detection algorithms. This has driven interest in minimally invasive treatments for prostate cancer.
One persistent controversy in prostate cancer treatment is the concept of the “index tumor.” Prostate cancer can be a multifocal process, but there is growing interest in finding the largest, most aggressive of these tumors and treating it, while monitoring the rest for progression.
Detection and characterization of prostate cancer
The current paradigm for prostate cancer detection is screening with serum prostate-specific antigen (PSA) sampling and digital rectal examination (DRE). The PSA serum test is not approved for screening, but only for therapy monitoring, in the United States, and its use in this regard is controversial. Literature both supports and refutes a reduction in mortality from screening, with a concomitant increase in costs. , An additional wrinkle is that not all prostate cancer secretes PSA, which can also be elevated in benign conditions such as prostatic hyperplasia (BPH) and prostatitis. Additionally, the United States Preventive Service Task Force has issued a draft whereby it assigned PSA screening a grade D recommendation, meaning that it recommends against the use of PSA for screening men.
There are multiple ways of evaluating PSA: Commonly, a measurement of 4.0 ng/mL or higher is considered suspicious, with 4.0–10.0 a “gray zone” of overlap with levels seen in BPH and prostatitis. The percent free PSA is higher in benign conditions, but there is a large degree of overlap between ratios seen in cancer and benign conditions. PSA density compares the value against the size of the prostate, with lower densities seen in BPH, but again a large degree of overlap. The best indicator of suspicion is PSA velocity or doubling time, which reflects the rate of change over time, but even this is controversial and, of course, it requires at least two time points.
Regardless of the utility of prostate cancer screening, if either the PSA test or DRE is abnormal, most physicians will refer the patient for biopsy. The biopsies are normally done systematically rather than targeted, and the number of cores varies between 6 (one per sextant) and over 20 in saturation biopsy procedures, but most physicians perform 10–12 cores, or 2 per sextant. Transrectal ultrasound (TRUS) is used to localize the prostate, but not commonly suspicious regions within it. The false-negative rate for standard 12-core systematic prostate biopsies varies in the literature but conservatively measures about 20%. The biopsies are reported in terms of the percentage and length positive and the Gleason score, which consists of the dominant and subdominant pattern ranging from 1 to 5. Therefore, a result of “30% (1.5 mm) Gleason 3+4=7” means that of the prostate tissue in the sample, 30%, or 1.5 mm, was predominantly Gleason pattern 3 with some pattern 4.
Current conventional treatment
Currently, the standards of care for prostate cancer include medical therapy, surgery, and radiation therapy. Surgical extirpation of the prostate has shown the highest long-term success for organ-confined prostate cancer. Traditionally, this was performed as an open surgery, but laparoscopic and robot-assisted surgery have overtaken this approach recently. Although the markedly improved visual resolution and fine control over the surgical field afforded by robot-assisted surgery purports to afford improved outcomes, both in cancer control and side effects, this has not been proved.
Radiation therapy can be performed for organ-confined and locoregional disease, including after surgery. External-beam radiotherapy (EBRT) is often supplanted or augmented with image-guided, intensity-modulated techniques sometimes called “stereotactic body radiotherapy (SBRT),” with different trade names (e.g., Leksell Gamma Knife from Elekta AB, Cyberknife from Accuray, or Novalis Tx from Varian) used in marketing. Radioactive seed placement in brachytherapy affords some of the lowest rates of associated morbidity, but is usually reserved for localized low-grade disease. High dose rate (HDR) brachytherapy involves placement of plastic catheters transperineally into the prostate, through which a robot places highly radioactive seeds for a limited amount of time in order to give specified radiation doses to tissue based on three-dimensional image-based planning software. HDR brachytherapy patients usually require only a 23-hour clinic stay, but this is not yet widely available.
Medical therapy can encompass hormonal modulation by lutein hormone releasing hormone agonists (e.g., leuprolide) or antiandrogenic compounds (e.g., bicalutamide) as well as chemotherapy. Surgical orchiectomy can also be considered for hormone modulation.
For patients with small-volume, low-grade disease, active surveillance rather than definitive management (surgery, radiation therapy, or other focal therapy) is sometimes considered, but even here the criteria vary by institution and some patients will exit surveillance because of anxiety.
Image-guided diagnosis
The first area of prostate cancer management where radiology can play a role is in improved diagnosis. This is primarily achieved by both noninvasive imaging and image-guided biopsy.
Although ultrasonography is an excellent modality to identify the location of the prostate, and can often resolve structures within it, it has shown relatively poor ability to discriminate benign from suspicious areas. Magnetic resonance imaging (MRI) has steadily improved over the past couple of decades in its ability to characterize the prostate, not only localizing suspicious areas, but also suggesting the aggressiveness of these areas. , Initially used for surgical planning, high-resolution T2-weighted images are acquired with the use of an endorectal coil, supported with information from magnetic resonance spectroscopic imaging (MRSI) used to identify an alteration of the normal choline–citrate ratio to suggest malignant transformation of tissue. The application of diffusion-weighted imaging (DWI) and its concomitant apparent diffusion coefficient (ADC) map, and dynamic contrast-enhanced (DCE) perfusion imaging, has nearly obviated the endorectal coil for all but the most demanding protocols, as modern scanners with high-performance gradients sacrifice only the very fine detail of the prostate capsule necessary for a highly detailed differentiation of stage T2 (organ confined) disease from T3 (extraprostatic extension). MRSI, although the most specific component of multiparametric MRI, is also sometimes omitted. ,
A dedicated limited protocol which incorporates thin-section, small-field-of-view turbo spin-echo T2-weighted imaging of the prostate in 2 or 3 planes along with DWI incorporating b-values from at least 100 s/mm to at least 800 s/mm and DCE perfusion imaging can accurately identify suspicious areas in less than ½ hour of scanner time. Criteria for prostate cancer on MRI are listed in Table 20-1 . Generally, each component is ranked on a 1–5 scale, with the sum (or average) giving the relative level of suspicion (see Table 20-1 ). Some centers will use purely quantitative ADC and pharmacokinetic perfusion values (e.g., k trans ), whereas others will use the qualitative appearance of the DWI and ADC map and curve types; the important consideration is that the reporting be standardized.
Score | T2 (Peripheral) | T2 (Central) | DWI/ADC | Perfusion | Spectroscopy |
---|---|---|---|---|---|
1 | Uniform high signal intensity (SI) | Heterogeneous TZ adenoma with well-defined margins: “organized chaos” | No reduction in ADC compared with normal glandular tissue. No increase in SI on any high b-value image (≥b800) | Type 1 enhancement curve | Citrate peak height exceeds choline peak height >2 times |
2 | Linear, wedge-shaped, or geographic areas of lower SI, usually not well demarcated | Areas of more homogeneous low SI, however well marginated, originating from the TZ/BPH | Diffuse, hyper SI on ≥b800 image with low ADC; no focal features; however, linear, triangular, or geographic features are allowed | Type 2 enhancement curve | Citrate peak height exceeds choline peak height >1, <2 times |
3 | Intermediate appearances not in categories 1/2 or 4/5 | Intermediate appearances not in categories 1/2 or 4/5 | Intermediate appearances not in categories 1/2 or 4/5 | Type 3 enhancement curve | Choline peak height equals citrate peak height |
4 | Discrete, homogeneous low signal focus/mass confined to the prostate | Areas of more homogeneous low SI, ill-defined: “erased charcoal sign” | Focal area(s) of reduced ADC but isointense SI on high b-value images (≥b800) | For focal enhancing lesion with curve type 2–3 | Choline peak height exceeds citrate peak height >1, <2 times |
5 | Discrete, homogeneous low signal intensity focus with extracapsular extension/ invasive behavior or mass effect on the capsule (bulging), or broad (>1.5 cm) contact with the surface | Same as 4, but involving the anterior fibromuscular stroma or the anterior horn of the PZ, usually lenticular or water-drop shaped. | Focal area/mass of hyper SI on the high b-value images (≥b800) with reduced ADC | For asymmetric lesion or lesion at an unusual place with curve type 2–3 | Choline peak height exceeds citrate peak height >2 times |
The oncologic interventionalist can then decide if any potential targets are worth sampling and has the option of multiple methods of doing so. Image fusion with transperineal CT-guided biopsy is an option but one not documented in the literature except after anorectal resection. Direct MRI-guided biopsy and MRI-ultrasound fusion biopsy are two well-documented choices.
Direct MRI-guided biopsy has been in development for more than a decade. The setup commonly involves the patient prone with an external phased array and a dedicated biopsy guidance apparatus for a transrectal approach. Standard T2-weighted images are acquired with the biopsy guide attached to the table. Dedicated planning software is then used for biopsy guidance with fine adjustments of the biopsy guide. The biopsy device is then passed through the guide, with optional imaging of the device in place ( Figure 20-1 ). A core is acquired, and the position can then be adjusted to acquire an adjacent core as needed. , There is currently one FDA-approved device in the United States (DynaTRIM, Invivo Inc., Gainesville, FL), with at least two others in development, one of which incorporates a dedicated endorectal coil.
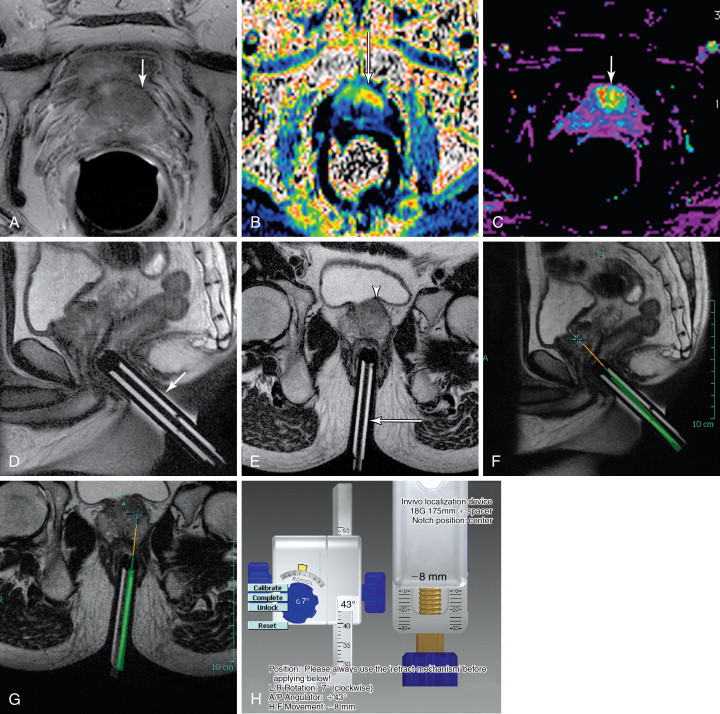
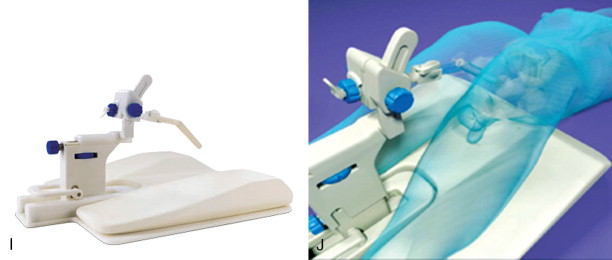
MRI-ultrasound fusion imaging is another consideration. Previously, this was done with “mental” image fusion. In other words, a planning MRI is acquired, and the same area is identified during TRUS based on the location of the target. One or more 18-gauge cores are then acquired. This is relatively straightforward to incorporate into standard systematic sextant biopsies. However, although this technique has been shown to significantly increase yield after a negative biopsy up to 55%, its reliability is uncertain. Prostate tissue characteristics can be quite different from MRI to ultrasound, complicating the coregistration.
New software and hardware combinations purport to fuse MRI and ultrasound data, either with image coregistration (Urostation, Koelis, La Tronche, France) or with mechanical localization (Artemis, Eigen, Grass Valley, CA). For this method, the targets and prostate are outlined on dedicated software, with semiautomatic registration of the prostate. The data are then transferred to the ultrasound interface. The prostate is scanned using a sweep of the ultrasound probe and its registration on the ultrasound data set is registered to the MRI data. The ultrasound interface then displays a three-dimensional representation of the prostate and targets with the direction of the probe and biopsy throw superimposed. The ultrasound probe is manipulated until the planned biopsy throw would intersect the target, and the biopsy device is then deployed ( Figure 20-2 ). These methods also allow for concomitant sextant biopsies. Some experts have found that, with experience, the image fusion becomes less important as they become attuned to how targets on MRI will appear on ultrasound and can identify them even in the absence of direct image fusion; this is even true of some urologists who are invested in imaging technology and work closely with their radiologists, such that these biopsies may be performed with a conventional TRUS setup after review of MR images.
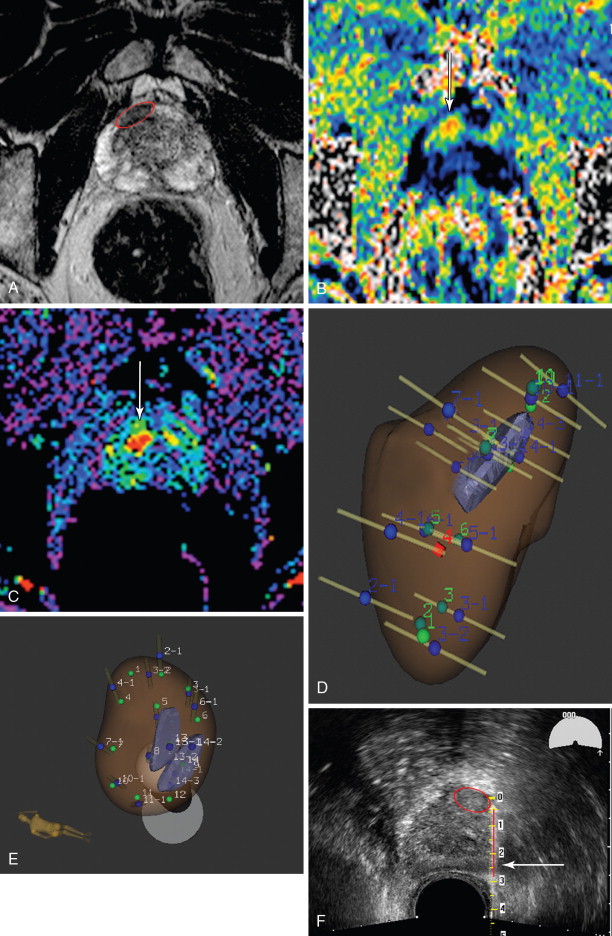
In both cases, because a transrectal approach will be undertaken, patients undergo a cleansing enema prior to arrival, and adequacy of the preparation is performed by DRE. Antibiotic prophylaxis, often a fluoroquinolone such as ciprofloxacin combined with a third-generation cephalosporin such as ceftriaxone, is taken before and also after the procedure. Analgesia is achieved with local anesthetic, such as 1% lidocaine administered just lateral to the prostate bilaterally, or (less effectively) with lidocaine jelly. Conscious sedation can also be employed. Commonly, at least two cores per target are acquired. Care should be taken to avoid the midline urethra. Complications are rare and include pain, bleeding, and prostatitis. Although a urethral fistula is possible, it is extremely uncommon.
Focal therapies for in situ prostate cancer
In order to minimize the morbidity associated with prostate surgery and radiation therapy in organ-confined disease, the concept of focal therapy has been developed as a middle ground between active surveillance and conventional definitive therapy. There are a number of choices of focal therapy modality, and a number of potential approaches. The choice of both is based in part on the extent of disease, in part on the expertise of the physician, and in part on the desires of the patient. The treatment can be as radical as ablation of nearly the entire prostate, sparing only one neurovascular bundle, or so focal as to only encompass the dominant prostate tumor excluding any subdominant tumors ( Figure 20-3 ). Focal treatment modalities include cryotherapy, high-intensity focused ultrasound (HIFU), photodynamic therapy (PDT), laser (photothermal) ablation, and, potentially, high dose rate brachytherapy, to name but a few. This section will cover HIFU, cryotherapy, and laser ablation. PDT is primarily a whole-gland treatment scheme, whereby a vascular-targeted photosensitizer is given intravenously and light is delivered using optical fibers placed using a brachytherapy grid, while the patient is otherwise kept in total darkness ( Figure 20-4 ). This procedure is relatively uncommon given the exquisite skin sensitivity to light resulting from the photosensitizer. High dose rate brachytherapy is primarily a whole-gland treatment regimen as well, but can be delivered focally. However, the treatment strategy and subsequent brachytherapy catheter placement is most commonly performed by the radiation oncologist.
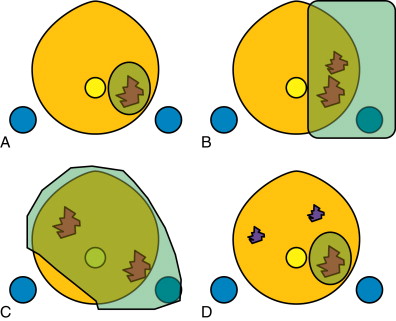
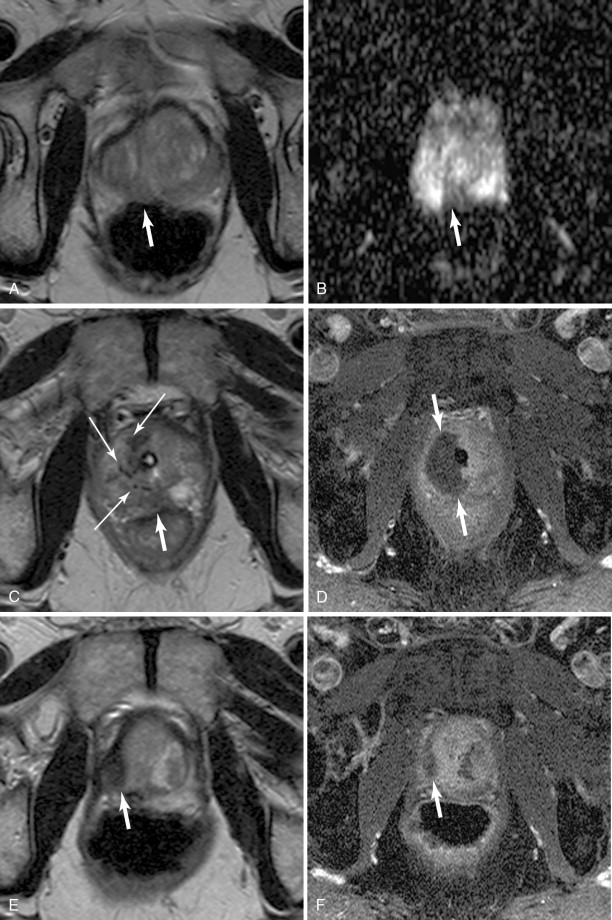
A consensus of experts recommends that potential focal therapy patients should have unilateral low- to intermediate-risk disease with clinical stage ≤cT2a, and that the choice of treatment type and extent will depend on the tumor volume and location and overall prostate size. Until recently, focal therapy usually involved treatment of the entire prostate, but an important consideration given imaging guidance is whether to only treat the “index” tumor, or the dominant suspicious area. How accurately it can be targeted, and the confidence in imaging and biochemical markers (such as PSA) to continue to survey the prostate for the need for additional treatment, will guide how “focused” the treatment is planned. This s ame consensus, however, specifically recommends template biopsies to confirm localization of disease, with MRI considered “an investigative tool” that would “require validation in prospective clinical trials” although this is not the practice of all the signers to the article in question.
High-intensity focused ultrasound
HIFU has the advantage that it is, potentially, completely noninvasive. There are two ways to guide the therapy, in combination with diagnostic ultrasound or using MRI guidance. The technique for the latter is more formally described in the chapter on uterine fibroid treatment, and, because it requires a sonographic window that would be obscured by the pubic symphysis, its pursuit for prostate cancer treatment has as yet been limited.
Therapeutic ultrasound is performed at lower frequency, usually 0.8–3.5 MHz, compared with diagnostic ultrasound, although modern ultrasound-guided HIFU units allow for imaging and therapy with a single transducer. During therapy, sound waves are focused to deposit the maximal power in a cylindrical volume of tissue, with the size usually on the order of a grain of rice (1–3 mm in diameter and 8–15 mm in length). Tissue ablation is accomplished by two processes. The deposition of thermal energy causes heating and resultant coagulative necrosis. Alternating cycles of compression and rarefaction from pressure alteration results in inertial cavitation as microbubbles of gas are drawn out of cells, which ultimately also results in coagulative necrosis. High-intensity devices deliver greater than 5 W of power per square centimeter. ,
Treatment units allow for planning of multiple adjacent ablation zones in order to accomplish larger volumes of treatment. Treatment planning is therefore accomplished using dedicated treatment planning software on the HIFU machine, and the treatment time varies based on the amount of tissue to be treated ( Figure 20-5 ). Treatment of the entire gland may take up to 2 hours or more.
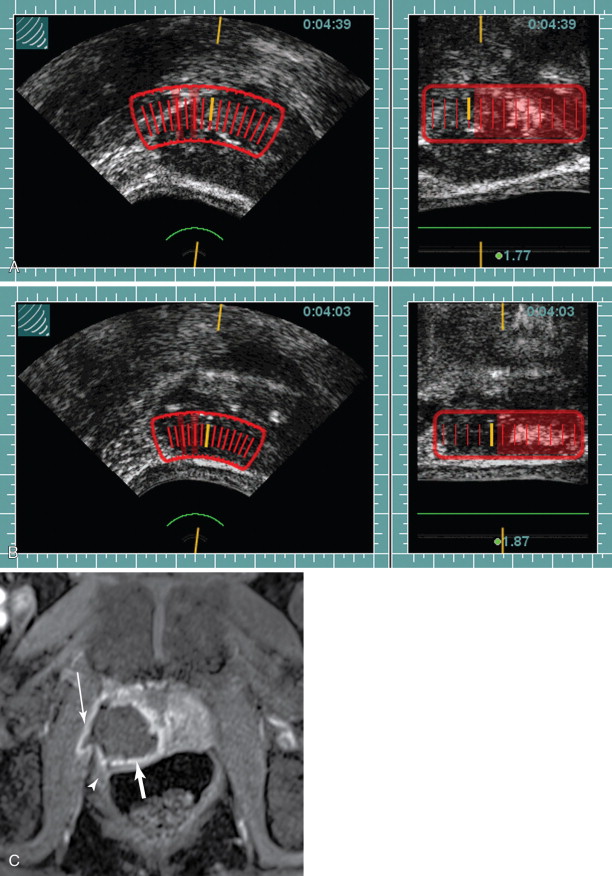
There are two HIFU systems commercially available. It should be noted that neither are approved by the United States Food and Drug Administration (FDA) for treatment of prostate cancer, but rather for uterine fibroids, although this modality is in clinical trials for prostate cancer ablation in the United States. The two available models are the Ablatherm® HIFU from EDAP-TMS (Lyon, France) and Sonablate® 500 from Focus Surgery (Indianapolis, IN). Both are approved in Europe for prostate treatment, and the former is approved in Canada, Russia, Australia, and South Korea and the latter in Japan.
Considerations in the choice of technology include safety monitoring of the rectum (available in both devices), focal length, and power density variation (available on the Sonablate 500) and power deposition either by preprocedure planning software (with Ablatherm) or by monitoring grayscale changes taken to signify steam generated by successful coagulation (on Sonablate 500).
Potential complications of HIFU, especially whole-gland treatments, include urethral stricturing, and for that reason, treatment is often combined with transurethral resection of the prostate (TURP). Rates of biochemical failure are difficult to determine, as the technique varies from series to series, but has been reported from as low as 0% (for PSA <1 ng/mL) to as high as 44% (PSA <0.1 ng/mL) in various series. Rates of incontinence also vary from as low as 0.5%–15% Stamey Grade I, and rates of erectile function are poorly characterized, if at all, in most series. More serious adverse effects include fistula formation reported in up to 2% of early series, although this is very rarely seen with modern equipment. , Also, given the relative novelty of this treatment and indolent nature of low-grade prostate cancer, mortality outcomes are not yet known. It is likely for this reason that its approval is lagging in the United States.
Cryotherapy
The tumoricidal effect of cryotherapy (or cryoablation) is likely primarily due to the formation of ice crystals, which burst cells through expansion. There is also the potential ischemic necrosis from vascular injury, dehydration, thrombosis from endothelial damage, and associated platelet activation, and possibly cryoactivation of an immune response to the tumor. The factors that determine efficacy include the nadir temperature, duration of freezing, rate of temperature normalization, and number of freeze–thaw cycles. Like any thermally ablative technique, heat sinks such as vessels may limit efficacy. In general, 3 minutes at −40°C is sufficient for tumoricidal effect. , However, many sites now rely on two freeze–thaw cycles for each probe position, with 8–11 minutes per freeze.
Modern cryotherapy devices use ultrasound guidance with a relatively small (17-gauge) treatment probe that allows for freezing with argon and thawing with helium gas. The technique is similar to that originally proposed. Normally, two to four probes are used per focus, with an additional probe placed contralaterally adjacent to the urethra or external sphincter but used for warming ( Figure 20-6 ). Urethral warming has been added to the original technique to reduce the likelihood of urethral sloughing. In addition, planning software, using three-dimensional ultrasound data combined with a traditional brachytherapy transperineal template for probe placement has made whole-gland treatment or hemiablation more straightforward. However, the prospect of true focal therapy of just the index lesion using real-time TRUS guidance remains a possibility, with the relative drawback that the ice ball limits resolution of tissue beyond it on ultrasound. Whether to use the transrectal approach, which may be crowded for multiple probe placements, or the transperineal brachytherapy-grid approach, varies based on user preference.
