Worldwide, 6,044,000 women are diagnosed with cancer and 3,345,000 die from the disease each year (1). From 1975–2010, the age-standardized incidence rates for cancers in women have increased by 42% (2). The increase has been 6% in women versus 2% in men between 1999 and 2010 (2,3). Gynecologic malignancies account for 12% of these cancers (3,4), with most of this increase occurring in the postmenopausal population.
One of the accepted strategies for combating cancer in the twenty-first century is screening of the asymptomatic population for premalignant conditions and early-stage disease. Such screening strategies are based on criteria laid down by the World Health Organization (WHO) (5) (Table 10.1). Mass screening for cervical cancer fulfills most of these tenets and organized screening programs in numerous countries have led to a significant reduction in cervical cancer mortality (6). Success is dependent on a number of factors in addition to the effectiveness of the strategy. It includes a recognized need, clear objectives, well-defined target population, detailed screening and management protocols, appropriate infrastructure, quality assurance, and ongoing program evaluation.
Population screening is being investigated for ovarian cancer (7). The disease is usually diagnosed in advanced stages when chances for long-term survival are poor. Effective treatment is available for early-stage disease and there is preliminary evidence from an earlier randomized controlled trial (RCT) that ovarian cancer screening may increase survival (8). Encouraging results have been reported from a single arm ultrasound study using ultrasound, which showed an increased 5-year survival in women who participated in the study compared to women from the same institution who did not (9). However, data from the ovarian arm of the Prostate Lung Colorectal and Ovarian (PLCO) Cancer Screening RCT has shown no impact of screening (using CA125 >35 kU/L and transvaginal scan [TVS]) on mortality from the disease (10). Data from the largest RCT, the United Kingdom Collaborative Trial of Ovarian Cancer Screening (UKCTOCS), are expected in 2015 (11,12). Encouraging sensitivity, specificity, and positive predictive value (PPV) has been reported in the latter trial for the multimodal strategy, which uses CA125 interpreted using the Risk of Ovarian Cancer (ROC) algorithm (12).
Table 10.1 World Health Organization Criteria for a Screening Program
Tumor Markers
Tumor markers are molecules or substances produced by or in response to neoplastic proliferation that enter the circulation in detectable amounts. They indicate the likely presence of cancer, or provide information about its behavior. In the context of screening the value of the marker depends heavily on its sensitivity (proportion of cancers detected by a positive test) and specificity (proportion of those without cancer identified by a negative test). An ideal tumor marker would have a 100% sensitivity, specificity, and positive predictive value (PPV). In practice, this is never achieved. The most limiting factor is lack of specificity because the majority of markers are tumor-associated rather than tumor-specific and are elevated in multiple cancers as well as in benign and physiologic conditions. In most diseases, tumor markers are not diagnostic, but contribute to the differential diagnosis. They may also have an important role to play in screening, determining therapeutic efficacy, detecting recurrence, and predicting prognosis.
A wide variety of macromolecular tumor antigens—including enzymes, hormones, receptors, growth factors, biologic response modifiers, and glycoconjugates—have been investigated as potential tumor markers. Innovative techniques such as mass-spectrometry and next generation sequencing are constantly identifying novel biomarkers that could complement those previously identified by candidate gene or antibody-based techniques.
The number of clinically useful markers is limited, despite significant research and the discovery of a large number of markers. Poor study design leading to inconsistent conclusions has been cited as an important reason (13,14). These design problems relate to all three phases of biomarker discovery and validation; preanalytical (selection of cases and controls, sample processing, and storage), analytical (detection limits and precision of assays), and postanalytical (overfitting and data interpretation to yield “the next promising” marker) (14).
General guidelines on the application and use of tumor markers have been developed by a number of multidisciplinary groups following critical appraisal of available evidence (15–19). The National Cancer Institute’s Early Detection Research Network has suggested five phases for biomarker development: Preclinical exploration, clinical assays and validation, retrospective longitudinal repository studies, prospective screening studies, and clinical randomized trials for assessing end points of cancer screening. Guidelines covering methodologic issues related to measurement and internal and external quality control have been published. However, systematic reviews such as those conducted by Cochrane Collaboration are lacking in relation to tumor markers. Use of clinical sample sets to identify early detection markers adds further bias. To address this, the use of the PRoBE (prospective-specimen collection, with retrospective-blinded evaluation) design for biomarker discovery and validation has been recommended (20). This approach involves blinded case-control studies nested within a prospective cohort that represents the target population, with biologic specimens and clinical data being collected prior to ascertainment of outcome such as ovarian cancer diagnosis on follow-up.
A variety of imaging modalities have also been explored to identify morphologic characteristics of cancer. In gynecology, real-time ultrasound is most commonly used because it has minimal side effects and provides detailed tumor morphology, which can be quantified using a variety of scoring systems. Detailed characterization of morphology on transvaginal scanning remains an important component of ovarian cancer screening. However as our understanding of the natural history of high-grade serous cancer improves, it is likely that higher resolution imaging modalities will be increasingly important.
Neovascularization associated with malignancy is another marker that has been exploited in screening. Color-flow Doppler to detect altered patterns of blood flow and decreased resistance in the thin-walled new vessels in ovarian cancers has not been successful, but emerging strategies such as targeted microbubbles or autofluorescence, which have been successful in cervical and oral cancer screening, may play a role in ovarian cancer screening in the future.
An important aspect of screening is defining the risk groups. The target population for sporadic ovarian cancer is defined by age (50 years and older) in clinical trials, and for familial ovarian malignancy by family history criteria and the presence of BRCA1 or BRCA2 mutations. In the last decade, there has been significant progress in better defining risk based on mutations in moderate risk genes such as RAD51, and on alterations in the more common low penetrance genes, together with lifestyle and reproductive factors.
Ovarian and Fallopian Tube Cancer
Ovarian cancer accounts for 4% of cancers occurring in women, with more than 190,000 new cases diagnosed worldwide each year. Incidence rates are highest in the United States and Northern Europe, and lowest in Africa and Asia, with ovarian cancer accounting for most of the gynecologic cancer mortality in the developed world. Women without a significant family history or known gene mutations have a 1–2% lifetime risk of developing the disease. Approximately 85% of cases occur after age 50, and 80–85% of cancers are epithelial in origin. High-grade serous tumors are the most common, present at an advanced stage, and have the poorest outcome (21).
Our ability to screen for the disease has been hampered by our lack of knowledge of the molecular and biologic events in ovarian cancer. This has limited the goal of screening to detection of asymptomatic, early-stage disease in the absence of a known precursor lesion (7). However, this has changed dramatically with descriptive molecular pathology and experimental evidence suggesting that a significant proportion of high-grade serous cancers start as premalignant serous tubal intraepithelial carcinoma (STIC) lesions in the fimbrial end of the fallopian tube (22,23). This has opened up the exploration of novel techniques and biospecimens for detecting these early lesions. While it is likely that detecting these lesions will significantly impact future screening strategies, current evidence does not support screening. Although the US ovarian cancer screening trial demonstrated no mortality benefit of screening with CA125 cut-off and transvaginal ultrasound (TVS) (10), the US Preventive Services Task Force is still awaiting the final results of the UK trial (12), which is using an algorithm-based approach (11).
Biochemical Markers
CA125
CA125 is a 200-kilodalton (kd) glycoprotein recognized by the OC125 murine monoclonal antibody and first described by Bast et al. (24) in 1981. On cloning, it was found to have characteristics of mucin designated as MUC16 (25). It carries two major antigenic domains, classified as A, the domain binding monoclonal antibody OC125; and B, the domain binding monoclonal antibody M11 (26). The first CA125 immunoassay used the OC125 antibody for both capture and detection (27,28). The second-generation heterologous CA125 II assay incorporated M11 and OC125 antibodies and is widely used for measuring CA125. There are a number of CA125 assays available, most of which correlate well with each other and are clinically reliable (29). However, differences in reagent specificities and assay design can lead to variation in the values obtained, and the results may not be interchangeable. Changes in methodology may require baseline samples to be retested or parallel tested using both assays (29,30). This is of particular importance for patients who are undergoing serial monitoring, such as in screening trials.
CA125 is not specific to ovarian cancer and is widely distributed in adult tissues. It is found in structures derived from the coelomic epithelium (such as endocervix, endometrium, and fallopian tube) and in tissues developed from mesothelial cells (such as pleura, pericardium, and peritoneum) (31). It is expressed in the normal adult ovary (32), and has been characterized in epithelial tissues of the colon, pancreas, lung, kidney, prostate, breast, stomach, and gall bladder (27,28).
CA125 levels in body fluids or ovarian cysts do not correlate well with serum levels. Serum concentration is a function of antigen production by the tumor and of other factors that affect its release into the circulation (33,34). The widely adopted cutoff value of 35 kU/L is based upon the distribution of values in healthy subjects, where 99% of 888 men and women were found to have levels below 35 kU/L (35). However, CA125 values can show wide variation, and are influenced by age, race, menstrual cycle, pregnancy, hysterectomy, and a number of benign conditions. In postmenopausal women, CA125 levels tend to be lower than in the general population, and levels below 20 kU/L have been found (36–39). Levels fluctuate during the menstrual cycle and increase during menstruation (27,40). Levels in white women have been found to be higher than in African or Asian women (41). Caffeine intake, hysterectomy, and smoking in some (41) but not all reports (42,43) have been found to be associated with lower levels of CA125 (41).
A number of benign gynecologic conditions such as endometriosis, fibroids, infections, and pelvic inflammatory disease may increase CA125 levels. In pregnancy, peak CA125 values occur in the first trimester and postpartum (27,44–46), with wide fluctuations in levels as high as 300 kU/L being reported at these times; levels return to normal by 10 weeks postpartum (45). Levels of 112 kU/L and 65 kU/L have been found to correspond to the ninety-ninth percentile and the ninety-sixth percentile in the first trimester, respectively (47,48) but ideally different levels need to be defined for different stages of pregnancy and puerperium (49). CA125 may also be elevated by nongynecologic diseases causing any inflammation of the peritoneum, pleura or pericardium, pancreatitis, hepatitis, cirrhosis, ascites, tuberculosis, and other malignancies such as pancreatic, breast, colon, and lung cancer (27,33). The benign and physiologic conditions associated with an elevated CA125 can cause false-positive results when CA125 is used in diagnosis or screening.
Approximately 85% of patients with epithelial ovarian cancer have CA125 levels of greater than 35 kU/L (35,50), with elevated levels found in 50% of patients with stage I disease and more than 90% of patients with stage II to IV disease (27). CA125 levels are less frequently elevated in mucinous and borderline tumors compared to serous tumors (27,51,52). CA125 can be elevated in the preclinical asymptomatic phase of the disease, because raised levels have been found in 25% of 59 stored serum samples collected 5 years before the diagnosis of ovarian cancer (37).
In a prospective ovarian cancer screening study of Swedish women, a specificity of 97% and PPV of 4.6% were achieved using CA125 (30 kU/L) in 4,290 volunteers aged 50 years and older (53). Data from the Shizuoka Cohort Study on Ovarian Cancer Screening (SCSOCS) found that the interval between the first detection of a slightly elevated CA125 level and the diagnosis of disease at surgery was significantly shorter in patients with serous-type ovarian cancer, compared with those with nonserous-type disease (1.4 vs. 3.8 years, p = 0.011) (54). Although 47% of nonserous-type ovarian cancers developed in the setting of elevated CA125 levels between 35 and 65 kU/L, 75% of serous ovarian cancers developed when the CA125 levels were normal (<35 kU/L) (54).
In postmenopausal women, an elevated CA125 in the absence of ovarian cancer has been found to be a risk factor for death from other malignant disease (55,56). These findings have implications when screening asymptomatic postmenopausal women.
Improving Sensitivity, Specificity, and Discriminatory Ability
Pelvic Ultrasound as a Second-Line Test
The specificity of screening with CA125 was initially improved by the addition of pelvic ultrasound as a second-line test to assess ovarian volume and morphology. Using multimodal screening incorporating sequential CA125 measurements and pelvic ultrasound, a specificity of 99.9% and PPV of 26.8% for detection of ovarian and fallopian tube cancer were achieved in an RCT of 22,000 postmenopausal women (57,58). With the accumulation of data, ovarian morphology has been used to refine algorithms for the interpretation of ultrasound in postmenopausal women with elevated CA125 levels (59,60). In UKCTOCS, the addition of TVS in the multimodal arm has shown similarly encouraging results (12).
Time-series Algorithms
Developing a more sophisticated approach to replace absolute cutoff levels for the interpretation of CA125 levels has been a key step in strategy development. Detailed analysis of more than 50,000 serum CA125 levels involving 22,000 volunteers followed for a median of 8.6 years in the study by Jacobs et al. (8,58) revealed that elevated CA125 levels in women without ovarian cancer were static or decreased with time, whereas levels associated with malignancy tended to rise. This finding has been incorporated into a computerized algorithm that uses an individual’s age-specific incidence of ovarian cancer and CA125 profile to estimate her Risk of Ovarian Cancer (ROC) (61–63). The closer the CA125 profile to the CA125 behavior of known cases of ovarian cancer, the greater the ROC. The final result is presented as the individual’s estimated risk of having ovarian cancer with a ROC of 2% implying a risk of 1 in 50. The ROC algorithm increases the sensitivity of CA125 compared with a single cutoff value, because women with normal but rising levels are identified as being at increased risk. At the same time, specificity is improved because women with static but elevated levels are classified as low risk. Based on the risk categories (low-, intermediate-, and elevated-risk) women can be triaged to repeat CA125 alone, or CA125 and TVS. An abnormal TVS, or a high (1 in 5) risk based on CA125 levels leads to gynecologic assessment with a view to surgery. For a target specificity of 98%, the ROC calculation achieved a sensitivity of 86% for preclinical detection of ovarian cancer (61).
This approach first showed encouraging performance characteristics when evaluated prospectively in a pilot RCT in the mid-1990s (64). On prevalence screening in the ongoing UKCTOCS (12,65), the ROC has achieved encouragingly high sensitivity (89%), specificity (99%), and PPV (40%) in the prevalence screening (12), which has persisted during incidence screening (66,67). Similarly high specificity and PPV were reported in a single arm ovarian cancer screening study of 4,051 postmenopausal US women who underwent up to 9 screens (68).
The ROC algorithm is being evaluated prospectively in pilot ovarian cancer screening trials in high-risk women under the auspices of the Cancer Genetics Network (CGN) (69) and Gynecology Oncology Group (GOG) (70) in the United States, and in the United Kingdom Familial Ovarian Cancer Screening Study (UKFOCSS) (71). Preliminary data from UKFOCSS (Phase II) where women have been screened 4-monthly have indicated high (67–100%) sensitivity for ovarian and tubal cancer. There were no interval cancers diagnosed, and the only cancers not detected on screening were occult cancers picked up at risk reducing surgery. Although only 42% of incident screen-detected ovarian/fallopian tube cancers were Stages I and II, 92% were completely cytoreduced (71).
Preliminary data from UKCTOCS have indicated that serial CA125 monitoring using the ROC algorithm is able to detect ovarian cancer at tumor sizes that are too small to be detected by transvaginal ultrasound. Independent retrospective data from the PLCO trial suggest that CA125 velocity in the women with cancer (19.749 kU/L/month) is more than 500 times that (0.035 kU/L/month) of those who do not have cancer, indicating that CA125 velocity and time-series algorithms are significant predictors of ovarian cancer (72).
The parametric empirical Bayes (PEB) longitudinal screening algorithm has been described. When evaluated in the PLCO samples, it was found to detect ovarian cancer earlier than the single threshold rule (73). The recent focus has been on developing algorithms incorporating multiple markers. Serial sample sets from trial biobanks such as PLCO and UKCTOCS are crucial to this effort.
Other Markers
Accurate discrimination between benign and malignant masses is essential to avoid unnecessary operations in women with benign lesions, and to plan suitable surgery by appropriately trained gynecologic oncologists in tertiary care centers for those with cancer (74–76). Both prospective and retrospective data show that CA125 can be used as an adjunct in distinguishing benign from malignant masses, particularly in postmenopausal women (77–80). Using an upper limit of 35 kU/L, a sensitivity of 78%, specificity of 95%, and PPV of 82% can be achieved for ovarian cancer. The addition of human epididymis protein 4 (HE4) may add to this in premenopausal women, because it is elevated in ovarian cancer and less frequently elevated in benign conditions, especially endometriosis, which is commonly found in younger women (81). It is commercially available as Risk of Ovarian Malignancy Algorithm (ROMA), a test that combines HE4, CA125 II, and menopausal status. A systematic review by Ferraro et al. (82) looking at the performance of HE4 and CA125 in identification of ovarian cancer in women with suspected gynecologic disease has concluded that HE4 is superior to CA125. In addition, HE4 may improve sensitivity (83). Immunohistochemical data suggest that both HE4 and mesothelin may be discriminatory in CA125-negative cancers (84). Analysis of a panel of nine markers—CA125, SMRP, HE4, CA72–4, activin, inhibin, osteopontin, epidermal growth factor, and ERBB2 (HER2)—found that the addition of HE4 to CA125 without the use of ultrasound increased sensitivity to 76.4% at a specificity of 90% and 81% at a specificity of 90% (85).
The option of using a combination or panel of markers rather than a single biomarker to improve screening performance has been explored. Of the many hundreds of serum markers that have been investigated, only a few have been validated for clinical use (86–89). Limited sensitivities and specificities further constrain their use for screening purposes. The majority of studies trying to identify markers complementary to CA125 have focused on clinical sample sets that were collected from symptomatic patients at the time of diagnosis, and controls recruited in hospital settings. They are therefore not representative of the population undergoing screening. Over the past few years, preclinical samples have become increasingly available from the biobanks of the large screening trials. Nested case-control studies within the PLCO cohort using the PRoBE design (20) have reported that within 6 months of diagnosis, serum CA125 remained the single-best biomarker for ovarian cancer (sensitivity of 86%, 95% CI 0.76 to 0.97) with HE4 the second best, with a sensitivity of 73% (95% CI 0.60 to 0.86).
The repeated validation of CA125 as the best single biomarker for ovarian cancer has led to efforts to further characterize CA125 in order to improve the performance characteristics. A cancer-specific glycoform of CA125 (O-glycosylated CA125) has been able to discriminate between invasive and benign ovarian neoplasms in women with CA125 elevations in the range of 30 to 500 kilounit/L, with a specificity of 60% at a sensitivity of 90% (90).
Modeling has been undertaken to define the requirements of a screening test that would be able to detect the tumor earlier than is currently possible. A model, which incorporates ovarian tumor growth and CA125 shedding data, has suggested that a tumor may grow unnoticed for over 10.1 years and reach a volume of 25 mm3 before becoming detectable by current blood assays. The specificity and detection limit of biomarker assays would need to improve 100-fold in order to enable detection within this first decade of tumor growth (91). Brown and Palmer (92) reached similar conclusions using published data from occult serous cancers found following prophylactic salpingo-oophorectomy in BRCA mutation carriers. They showed that serous cancers spend, on average, greater than 4 years in situ, stage I or II, and possibly a further 1 year as stage III or IV, before they present clinically. The tumors are less than 1 cm during this occult period, only increasing to 3 cm when they progress to stage III or IV (93). An annual screen would need to detect tumors 1.3 cm in diameter to detect half the tumors in stage I and II, and tumors of 0.5 cm diameter to achieve a 50% reduction in serous ovarian cancer mortality. The goal in detecting high-grade serous ovarian cancer may need to be in detection of low volume rather than early-stage disease.
New High-Throughput Approaches
Proteomics is the study of the expression, structure, and function of all proteins as a function of state, time, age, and environment (94). Although the initial euphoria related to ovarian cancer proteomic profiles and markers has been significantly moderated by a number of reports pointing out potential issues such as cross-platform reliability, reproducibility, sample processing, and analytical sensitivity of minute samples (95–99), significant progress has been made in the last decade in use of proteomic technologies to identify peptides or individual markers that relate to cancer risk (100,101). A combination of mass spectra generated by these new technologies and artificial intelligence–based informatic algorithms is being used to discover small sets of key protein markers that can discriminate normal from ovarian cancer patients (102) and can then be validated using more conventional assays. Recent refinements in these technologies have meant that fine mapping of a number of markers is feasible in a much shorter space of time (97,103). Advances in bioinformatics are leading to the development of sophisticated algorithms to analyze the large volume of preprocessed mass-spectrometric data and identify the most informative “common” peaks, but these approaches still need further refinement (96,98).
Recent studies using proteomic technology have confirmed that CA125 remains the best single marker for nonmucinous ovarian cancer, but 60 autoantibodies specific to ovarian cancer and independent of CA125 levels have also been discovered (104). Other identified proteomic markers such as C-reactive protein (105) are proteins and peptides that are produced as part of the host response to inflammation and are unlikely to be of clinical utility. It is likely that markers discovered through proteomic profiling will be important in the future. Well-designed, large prospective, multicenter clinical trials are required to validate and standardize this technology (99,106).
Metabolomics refers to the rapid, high-throughput characterization, and quantification of small-molecule metabolites, which are the end products of cellular regulatory processes. Preliminary data suggest that metabolomics is a promising automated approach, in addition to functional genomics and proteomics, for analyses of molecular changes in malignant tumors. In a preliminary study of 66 invasive ovarian carcinomas and 9 borderline ovarian tumors, a gas chromatography/time-of-flight mass spectrometry (GC-TOF MS) was able to detect 291 metabolites, of which 114 were already annotated compounds. A model built on these data could distinguish 88% of the borderline tumors from the carcinomas (107).
Epigenetic mechanisms have been shown to be extremely important in the initiation and progression of human cancer (108). Freely circulating hypermethylated tumor-derived DNA has been shown to be present in serum or plasma of patients with cancer (109,110). However, before the clinical utility of circulating epigenetic markers can be determined, a number of issues related to standardization of methodology, type of assay, reproducibility, efficacy, and comparison with other markers need to be addressed (111). In a clinical case-control series, a DNA methylation signature was found to distinguish patients from healthy controls (112). More promising, a multiplex methylation-specific PCR assay of seven candidate genes that analyzes the methylation status of cell-free serum DNA was shown to have superior sensitivity (85.3% vs. 56.1%) and specificity (90.5% vs. 64.15%) compared with CA125 alone in detecting early-stage ovarian cancer (113).
miRNAs are generally stable and can be detected in serum and plasma. Initial tissue based studies identified some miRNA that were upregulated and others that were downregulated when profiling ovarian cancers and normal ovaries (114–116). DNA released from dead cancer cells varies in size, whereas DNA released from nondiseased cells undergoing apoptosis is uniformly truncated (117). Circulating tumor DNA may serve as a surrogate marker for active, fast-growing invasive tumors (111). Cell-free DNA has been suggested as a potential marker, as it reflects the release of both normal and tumor-derived DNA into the circulation through cellular necrosis and apoptosis. In a study of 164 women with invasive epithelial ovarian carcinoma, 49 with benign ovarian neoplasms and 75 age-matched controls, elevated preoperative total plasma cell-free DNA levels were observed. Women with levels >22,000 GE/mL had decreased survival (p < 0.001), and a 2.83-fold increased risk of death from ovarian cancer (p < .001) (118).
Cancer Specific Biomarkers and Novel Biospecimens
Mutations in cancer-related genes TP53, EGFR, BRAF and KRAS are some of the early molecular genetic events in ovarian cancer. New technologies, such as BEAMing, are able to detect small amounts of mutant alleles in cell-free body fluids, which can be quantified with unprecedented sensitivity (119). Forshew et al. (120) recently identified mutations in TP53 at allelic frequencies of 2–65%, in plasma from patients with advanced ovarian cancer who had high levels of circulating tumor DNA (ctDNA).
Kinde et al. (121) recently reported a sensitive massively parallel sequencing method to detect mutations in a panel of 12 genes. Applying this method to 14 liquid cytology cervical samples from women with ovarian cancer who had mutations, they were able to identify the expected tumor-specific mutations. These results suggest that tumor DNA can be detected in a proportion of patients with ovarian cancer in a standard liquid-based cervical cytology specimen (121). Salivary transcriptomes have also been evaluated as possible ovarian cancer markers (122).
Morphologic Markers
Real-time ultrasonic screening is aimed at detecting the earliest possible architectural changes in the ovary that accompany carcinogenesis. The transvaginal route is preferred because its better resolution leads to a more accurate assessment of ovarian and endometrial morphology, and it does not require a full bladder. However ultrasound has a subjective element (123), and therefore there is a need for accreditation and quality assurance in large multicentre screening. Encouragingly, postmenopausal women undergoing screening find TVS acceptable (124).
Both ovarian volume and morphology have been assessed, with cutoffs for volume ranging from 10–20 mL, depending on menopausal status (125). The persistence of abnormalities on repeat scanning 4 to 6 weeks after initial detection helps to reduce false-positive rates and increase PPV (126). Even when ovarian lesions appear to be complex, solid, or bilateral, many may resolve, as demonstrated by serial ultrasonography in the University of Kentucky Ovarian Cancer Screening Program (127). The lack of physiologic changes in ovarian volume in postmenopausal women further decreases the number of false positives in this group.
Even in older women, there is a high prevalence of benign ovarian lesions. In an ultrasonic and histopathologic autopsy study of 52 consecutive postmenopausal women who died from causes other than gynecologic or intraperitoneal cancer, 56% were found to have a histologically confirmed benign adnexal lesion of 50 mm in diameter (128). Ultrasonography used in this manner can therefore lead to the detection of many benign ovarian tumors, which results in unnecessary surgery in healthy, asymptomatic women.
As data accumulate with long-term follow-up of the participants of the early screening trials, it has been possible to further define the risk of ovarian cancer associated with various ultrasonic morphologic findings. Unilocular ovarian cysts less than 10 cm in diameter are found in 18% of asymptomatic postmenopausal women aged over 50 years and are associated with an extremely low risk of malignancy (129). In contrast, complex ovarian cysts with wall abnormalities or solid areas are associated with a significant risk of malignancy (126,129). Restricting the definition of abnormality to such complex ovarian morphology helps increase the specificity and PPV of ultrasonic screening (60).
To further decrease the number of false positives, some screening protocols use a weighted scoring system or morphologic index based on ovarian volume, outline, presence of papillary projections, and cyst complexity (i.e., number of locules, wall structure, thickness of septae, and echogenicity of fluid). There is no standardized index as yet (130–135). Others use subjective assessment of the gray-scale images. Based on gross anatomic changes at the time of surgery, papillary projections have the highest and simple cysts and septal thickness the lowest correlation with a diagnosis of ovarian malignancy (136). The University of Kentucky group has developed a serial morphologic index, which increased with time in the cancers, whereas it either decreased or remained stable in those with benign tumors, akin to the change in CA125 with time. The addition of this index in a serial-performing algorithm may increase specificity in screening by reducing the false positives (137). Newer modalities, such as pattern-recognition computer models and artificial neural networks (138–141), may increase the reproducibility of results and improve ultrasonic performance.
In the differential diagnosis of benign from malignant disease, a number of strategies have been explored to reduce the false-positive rate and facilitate discrimination between benign and malignant ovarian lesions. Subjective evaluation using gray scale and Doppler (pattern recognition) by an experienced examiner has been reported to be better than mathematical logistic regression models (141) or CA125 alone (142). In a systematic review of risk scores available to triage pelvic masses, the Risk of Malignancy Index (RMI) has been found to be the best predictor of malignancy and the model of choice (143). The RMI combines serum CA125 values with ultrasound detected ovarian morphology and menopausal status (144). The pooled sensitivity for an RMI cutoff of 200 was 78% at 87% specificity (143). Ultrasonic assessment for an “ovarian crescent sign” in women with an RMI above 200 has been shown to improve accuracy (145).
The sensitivity of RMI for early-stage disease is low when CA125 levels are normal and scan findings a poor discriminator. Nevertheless, in the premobile computing era, the ease of applying the RMI has gained widespread clinical use. The International Ovarian Tumor Analysis (IOTA) group reported a logistic regression model incorporating 12 variables, which was superior to CA125 alone (146,147) and equivalent to RMI even when the ultrasonic scanning was performed by operators with varied training (148). A systematic review looking at various imaging modalities has suggested that 3D ultrasonography has a higher sensitivity and specificity when compared to 2D ultrasonography, and of the more sophisticated imaging modalities, magnetic resonance imaging performed the best (149). Formal evidence of their effectiveness in reducing surgical intervention in screening trials is awaited.
Increasingly, conservative management is the norm for adnexal cysts judged to be benign at transvaginal ultrasonic examination when they are detected either incidentally (150) or in screening trials in postmenopausal women. Follow-up of such women in ongoing randomized screening trials will be important in validating such strategies.
Development of new imaging diagnostic approaches is expected to significantly advance detection of ovarian cancer. One such approach explores use of light-induced intrinsic tissue fluorescence or autofluorescence, which is lost in cancerous/precancerous epithelial tissue and has been successfully used in cervical and oral cancer screening. In a preliminary study using ex vivo reflectance and autofluorescent optical imaging of fallopian tubes removed at surgery, McAlpine et al. (151) demonstrated encouraging sensitivity (73–100%), specificity (83–92%), PPV (50–78%), and NPV (91–100%) for detection of STIC lesions. Its use in screening will require improvement of the predictive ability, and its incorporation into endoscopy to allow in vivo real-time imaging of the fallopian tube lumen. Other modalities being explored in mouse models include laparoscopic nonlinear microscopy (152).
Vascular Markers
Neovascularization is an obligate early event in tumor growth and neoplasia (153). Fast-growing tumors contain many new vessels that have less smooth muscle in their walls and therefore provide less resistance to blood flow when compared with vessels within benign ovarian tumors. Color-flow Doppler imaging uses these altered blood-flow patterns as markers to differentiate malignant from physiologic and benign lesions. It has been used as a first-line screening test in combination with transvaginal ultrasound (154,155) and as a second-line test following an abnormal ultrasound (156,157) in both general and high-risk population screening.
The early promise of Doppler to differentiate between malignant and benign ovarian masses and therefore improve the specificity of ultrasound (154,155) was not confirmed in subsequent studies (135,158,159). Although it was demonstrated that the mean pulsatility index of vessels supplying ovarian cancers was lower than that of vessels supplying benign ovarian tumors, the overlap in vascular resistance between these two groups prevented reliable separation of malignant from benign disease. It was thought that lack of blood flow in an ovarian tumor, as detected by color Doppler, may preclude cancer (159), but this was not substantiated, as 6% of ovarian tumors without blood flow were malignant in the Kentucky screening trial (135). Even when Doppler examinations were simplified and limited to the expression of internal color flow, gray-scale sonography was still a more sensitive indicator of malignancy than Doppler sonography (160). The effectiveness of a screening strategy that incorporates Doppler evaluation of ovarian masses in addition to gray-scale sonography is yet to be established. Although UKCTOCS is collecting Doppler data on abnormal adnexal masses detected on ultrasonic screening, these data are not part of the screening algorithm (12).
For differential diagnosis of women presenting with pelvic masses, color Doppler flow indices and tumor morphology, as assessed by gray-scale imaging, have been incorporated into a logistic regression model (146), which has been prospectively validated in a multicenter study (147). Pattern-recognition models using a combination of gray-scale and color Doppler ultrasound have been found to be better than CA125 alone in differential diagnosis (142). Some studies have shown that three-dimensional power Doppler examinations may be more accurate than two-dimensional Doppler examinations (161,162), although this remains controversial (163). Data have demonstrated the superior performance of color flow Doppler compared to 2D scanning to analyze blood flow to suspicious areas or masses (149).
Contrast-enhanced transvaginal ultrasound with microbubbles that are small enough to pass through capillaries can detect abnormal flow found in areas of neovascularization (164). The higher resolution of such techniques may allow detection of low-volume tumors in future screening strategies. A more powerful use of contrast-enhanced ultrasonic imaging is by targeting tumor angiogenesis at the molecular level. Ultrasonographic microbubbles may be targeted with one of several antibodies. As the tumors grow, there is a change in the relative uptake of each targeted microbubble, suggesting that such noninvasive molecular profiling of tumor angiogenesis could be useful as a diagnostic tool (165).
Target Populations
Two distinct populations are at increased risk for ovarian cancer: the general population and a high-risk population.
General Population
Most ovarian cancers are sporadic, and occur in the general population. More than 90% of sporadic cancers occur in women older than 50 years, so screening studies in the general population usually target this group. Some of the other known risk factors in the general population such as oral contraceptive use, parity, and hysterectomy have the potential for determining risk. Over the past few years, as a result of a massive international effort of the Ovarian Cancer Association Consortium (OCAC), genome-wide association studies (GWAS) have identified eight susceptibility loci for serous epithelial ovarian cancer (166–170). By themselves, these loci confer increased risk of magnitude less than 1.5-fold. However, a multiplicative effect of gene–gene and gene–environment interaction may allow better risk prediction (171), similar to the “polygenic” approach of combining multiple, low-penetrance susceptibility alleles to refine breast cancer risk stratification (172). The implementation of such a screening strategy will depend on organizational, ethical, legal, and social factors, in addition to usefulness and cost-effectiveness (173).
High-Risk Population
Hereditary syndromes account for approximately 5–10% of ovarian cancers. Female relatives of affected members from ovarian, breast, breast and ovarian, or Lynch syndrome (LS) families who have a greater than 10% lifetime risk of developing ovarian cancer are considered to be high risk. Much of this risk results from mutations arising in the BRCA1, BRCA2, and mismatch repair (MMR) genes. A recent meta-analysis has indicated that the average cumulative risk by age 70 for ovarian cancer is 40% (35–46%) in BRCA1 mutation carriers and 18% (13–23%) in BRCA2 mutation carriers (174). A genome-wide association study (GWAS) of BRCA1 carriers has identified two novel ovarian cancer risk modifier loci, 17q21.31 and 4q32.3. The first is associated with both BRCA1 and BRCA2, while the latter has only a BRCA1-specific association. The risk of ovarian cancer can now be recalculated based on these known ovarian cancer risk-modifying loci. It could be 28% or lower (in the 5% of BRCA1 carriers at the lowest risk), or 63% or higher (in the 5% at the highest risk) (175). These recent findings have important implications for risk prediction and clinical management for BRCA1 carriers. MMR gene-mutation carriers have a lifetime risk of ovarian cancer of approximately 10–12% (176).
Current Ovarian Cancer Screening Trials
Two distinct screening strategies have emerged, one based on ultrasound and the other on measurement of serum CA125, with ultrasound as the second-line test (multimodal screening) (12,68,125,177–188). Overall, the data from large prospective studies in the general population (Table 10.2) suggest that sequential multimodal screening has superior specificity and PPV. However, ultrasonography as a first-line test may offer greater sensitivity for early-stage disease.
Trials in the General Population
The RCTs in the general population aim to assess the impact of screening on ovarian cancer mortality. In the UKCTOCS trial, 202,638 postmenopausal women aged 50 to 74 have been randomized in a 2:1:1 fashion to either control or annual screening with transvaginal ultrasound (ultrasound group) or CA125 interpreted using the ROC algorithm followed by TVS as a second-line test (multimodal group). Apart from ovarian cancer mortality, the trial also addresses the issues of compliance, health economics, and the physical and psychological morbidity of screening. Both strategies have had encouraging sensitivity for primary invasive epithelial ovarian and fallopian tube cancers (89.5% and 75%, respectively) on the prevalence (initial) screen. The multimodal strategy had a significantly higher PPV (35.1% vs. 2.8%), resulting in lower rates of repeat testing and surgery, likely reflecting the higher detection rates of benign adnexal masses and borderline tumors in the ultrasound group (12). The superior sensitivity (87.7% vs. 67.6%) and PPV (22.3% vs. 4.4%) of the multimodal compared to the ultrasound strategy was confirmed on incidence screening (66,67). Screening in UKCTOCS was completed in December 2011, with the results of the mortality impact of screening awaited in 2015.
Multimodal screening is currently ongoing in the single-arm prospective study of 4,051 low-risk postmenopausal US women aged 50 and older in the MD Anderson study (68). During 11 years of screening, the ROC algorithm had picked up 4 of the 10 women with ovarian cancer, all of whom had “early-stage, high-grade” disease, with no interval cancers reported. This study has provided independent validation of the UK findings.
The PLCO Cancer Screening Trial enrolled 78,237 women aged 55 to 74 at 10 screening centers in the United States, with balanced randomization to intervention and control arms. The women underwent annual screening with both serum CA125 (interpreted using a 35 kU/L cutoff) and transvaginal ultrasound for 3 years followed by CA125 alone for a further 2 years. In the trial, all positive screening tests and abnormal findings were evaluated and managed by the participants’ physicians, with no prescribed study protocol (178). The results of the prevalence screen in 28,816 screened women reported 29 neoplasms, of which 20 were invasive and 9 borderline. Of all screened women, 4.7% had an abnormal scan and 1.4% an abnormal CA125. The PPV for invasive cancer was 3.7%, 1%, and 23.5% for abnormal CA125, TVS, and both CA125 and TVS, respectively (178). The trial has reported on the impact of screening on mortality. At a median follow-up of 12.4 years (25th–75th percentile 10.9 to 13), there were 118 and 100 deaths in the screening and control arm, respectively, with a mortality rate ratio of 1.18 (95% CI 0.91 to 1.54) (10). The validity of these findings has been questioned in view of the lengthy follow-up after end of screening, with 40.6% of the ovarian cancers being diagnosed after screening had been completed, and lack of a protocol driven screening and treatment (189). Crucially, a single threshold rule was used to interpret CA125. The trial reported a high (15%) complication rate in women undergoing surgery for false-positive findings (10). Based on these findings, USPSTF reaffirmed their previous recommendation that screening for ovarian cancer should not be undertaken in the general population (11). Despite this, a survey of US physicians showed that one in three physicians believed that ovarian cancer screening was effective and were likely to offer it to the women (190).
Table 10.2 Prospective Ovarian Cancer Screening Studies in the General Population
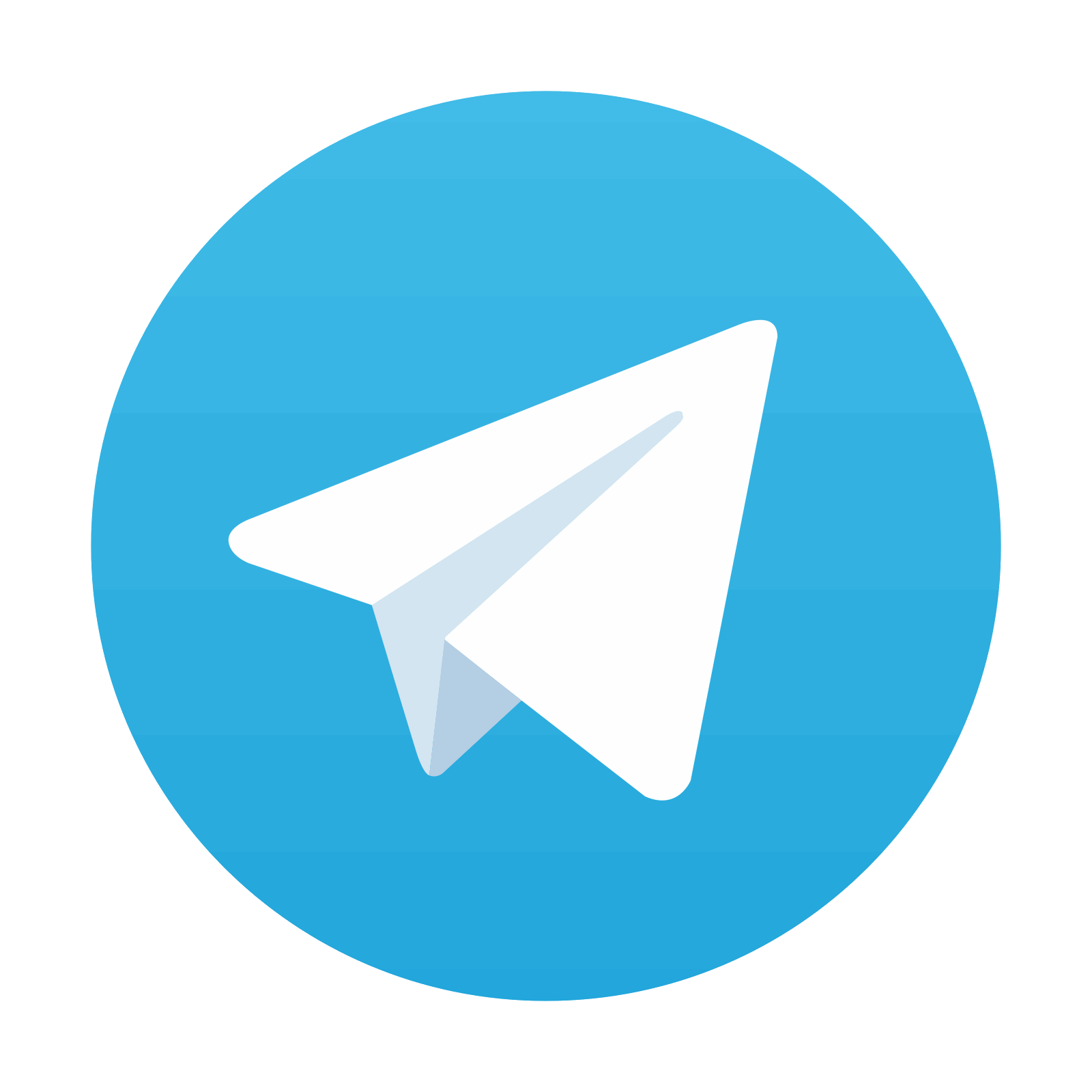
Stay updated, free articles. Join our Telegram channel

Full access? Get Clinical Tree
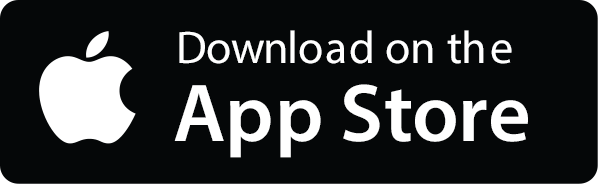
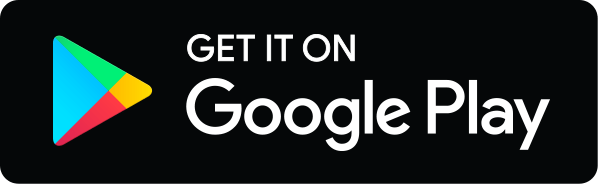