HCV belongs to the genus Hepacivirus in the Flaviviridae family (Forns and Bukh 1999). It has a single-stranded linear RNA genome of approximately 9,600 nucleotides that encodes a large polyprotein of approximately 3,000 amino acids (Bartenschlager et al. 2011). The structure of the HCV genome and functions of the various viral proteins are shown in Fig. 2. HCV exists as six major genotypes with genotype 1 being the dominant genotype in the USA, Europe and Australia (Simmonds et al. 2005). Genotype 3 is more prevalent in India and Southeast Asia, whereas genotype 4 is more commonly seen in the Middle East and Africa (Kamal and Nasser 2008). Each genotype contains multiple subtypes that are detected by viral sequencing and that are identified with lower case letters (a, b, c, etc.). HCV genotype influences treatment response and also the severity of liver disease. For example, chronic infection with genotype 1b may be associated with the development of more advanced fibrosis, cirrhosis and HCC. In fact, a recent meta-analysis found that genotype 1b HCV infection was associated with a doubling of the risk of HCC development compared to infection with other genotypes (Raimondi et al. 2009).
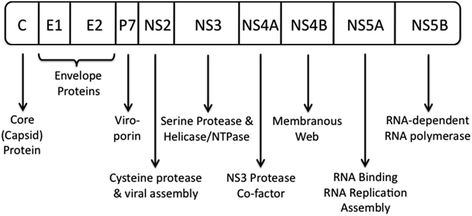
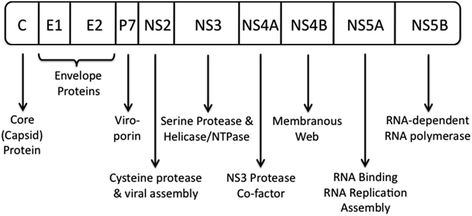
Fig. 2
HCV genome structure and functions of viral proteins. Adapted from Bartenschlager et al. (2011)
Like other RNA viruses, the RNA polymerase protein of HCV lacks proofreading ability, and as a consequence, replication of the viral genome is error-prone resulting in a high mutation rate. The result is great genetic heterogeneity that leads to the evolution of diverse viral quasispecies. This viral diversity interferes with the development of effective host humoral immune responses against the virus, thereby promoting viral persistence within infected individuals (Forns and Bukh 1999).
3 Hepatitis C and Associated Risk Factors for HCC Development
HCV is recognized as a major cause of HCC globally. In a large population-based prospective study, infection with HCV conferred a 20-fold increased risk of developing HCC compared to HCV-negative individuals (Sun et al. 2003). This strong association between chronic HCV and HCC has been noted since the early 1980s, when the virus was known as non-A, non-B hepatitis (Kiyosawa et al. 1984). Almost all HCV-related HCCs occur in the setting of established cirrhosis, with cirrhosis itself being a strong independent risk factor for developing HCC. In the setting of HCV-induced cirrhosis, the incidence of HCC is between 2 and 8 % per year (Bruix et al. 2005). Consequently, HCC develops many years (often 2–3 decades) after initial HCV infection.
However, not all HCV-related HCCs occur in patients with pre-existing cirrhosis. In a large prospective study, about 17 % of HCV-positive patients with HCC were not cirrhotic, but were noted to have at least an Ishak fibrosis score of 3 or more on serial liver biopsies (Lok et al. 2009), indicating that even in the absence of cirrhosis, HCC may develop in HCV-infected individuals with established chronic hepatitis and advanced liver fibrosis.
Risk factors that may contribute to the progression of HCV-associated liver disease leading to cirrhosis and HCC include concurrent alcohol consumption, older age at time of infection, male gender, co-infection with HIV or hepatitis B, immunosuppression, associated insulin resistance or non-alcoholic steatohepatitis, and a higher degree of inflammation and fibrosis on liver biopsy (Chen and Morgan 2006).
Significant alcohol intake of >40 g alcohol/day in women and >60 g of alcohol/day in men for more than 5 years is associated with a two to threefold increased risk of cirrhosis and decompensated liver disease in HCV-infected individuals (Wiley et al. 1998). Furthermore, the risk of developing HCC is doubled in HCV-infected individuals who consume >60 g of alcohol/day compared to those consuming <60 g/day (Donato et al. 2002). Also, the presence of chronic HCV infection has been associated with more advanced liver disease and increased mortality in alcoholic individuals compared to alcoholic patients with chronic hepatitis B infection (Mendenhall et al. 1991).
Age of infection is also an independent risk factor for the development of more severe liver disease in chronic HCV. After controlling for duration of HCV infection, patients who acquire HCV infection at an older age (>40 years old) are significantly more likely to progress to advanced liver fibrosis than individuals infected at a younger age (Poynard et al. 1997). The incidence of HCC is up to 29 times higher for individuals who become infected with HCV after 39 years of age compared to those infected before the age of 19 years (Pradat et al. 2007).
In the setting of HIV–HCV co-infection, a low CD4 count is associated with higher HCV viral loads as well as an accelerated progression to cirrhosis (Di Martino et al. 2001). Other causes of immunosuppression, such as organ transplantation, have also been associated with more rapid liver fibrosis progression (Berenguer et al. 2000). Finally, individuals with HCV–HBV co-infection may also be at a higher risk of HCC development (Cho et al. 2011).
How HCV infection results in the development of HCC is not entirely clear. There is evidence to suggest that HCV may interact with various intracellular signal transduction pathways or effect epigenetic changes, thereby altering hepatocyte physiology to directly promote malignant transformation. For example, HCV core protein has been noted to interact with the mitogen-activated protein (MAP) kinase signalling pathway, thereby promoting cell proliferation (Hayashi et al. 2000). Also, it has been shown that the tumour suppressor gene p16INK4A in tumour tissue resected from the livers of HCV-infected patients with HCCs is hypermethylated, and this results in the inactivation of p16INK4A, a feature not seen in non-HCV-associated HCCs (Li et al. 2004). Secondly, immune-mediated liver inflammation and the promotion of apoptosis of HCV-infected hepatocytes result in a compensatory stimulation of cell proliferation to replace dead hepatocytes. The increased cell turnover permits the accumulation of genetic mutations within hepatocytes, and this together with the surrounding inflammatory liver milieu promotes HCC development (Hino et al. 2002).
4 Prevention of HCC in Patients With Hepatitis C–induced Cirrhosis
The incidence of HCV-related HCC continues to rise worldwide because of the increasing number of individuals with chronic HCV infection, the presence of associated co-morbidities and the longer survival of patients with advanced liver disease as a result of improved management of the complications of liver failure. HCC develops at an annual rate of about 3 % in patients with HCV-related cirrhosis, with an 11.5 % four-year risk of HCC (Serfaty et al. 1998), hence emphasizing the importance of HCC screening in this population. The successful clearance of HCV with interferon therapy in patients with cirrhosis is associated with a threefold reduction in the risk of subsequent HCC development (Bruno et al. 2007; Singal et al. 2010b), indicating that despite the presence of cirrhosis, successful antiviral therapy of HCV-infected patients will still reduce the future risk of HCC. However, as the risk of HCC is not completely eliminated despite achieving viral eradication, ongoing HCC screening is still indicated in patients with cirrhosis. In contrast, there appears to be no benefit of interferon therapy on reducing HCC risk if viral eradication has not been successful. In patients with HCV-induced cirrhosis who have not achieve viral clearance with standard antiviral therapy, the provision of long-term half-dose maintenance pegylated interferon may reduce the incidence of HCC development (Lok et al. 2011). In this study, the beneficial effect in reducing HCC incidence was not seen in HCV-infected patients who had advanced hepatic fibrosis but not cirrhosis. However, in a meta-analysis of four studies with 1,152 non-responders, there was no statistically significant reduction in HCC risk in patients maintained on low-dose interferon compared with those who did not receive maintenance therapy (Singal et al. 2010b).
A meta-analysis of patients with HCV-induced cirrhosis who developed HCC has shown that after curative treatment for HCC via local ablative therapy or surgical resection, successful eradication of HCV with antiviral therapy was associated with a reduced risk of HCC recurrence from 61 to 35 % (Singal et al. 2010a). Furthermore, successful treatment with pegylated interferon and ribavirin was associated with improved hepatic functional reserve and increased survival (96 % vs. 61 % at 3 years) in this cohort (Ishikawa et al. 2012). In patients undergoing liver transplantation for HCV-associated HCC, interferon therapy for recurrent HCV post-liver transplant was found to decrease subsequent HCC recurrence from 27 to 4 % (Kohli et al. 2012). These studies indicate that antiviral therapy is important in the secondary prevention of HCC in HCV-induced cirrhosis.
5 Prevention of Cirrhosis and HCC in Patients with Hepatitis C–Induced Chronic Hepatitis
The potential long-term benefits of successful antiviral therapy of HCV-infected patients with chronic hepatitis include the normalization of serum transaminase levels a reduction in hepatic necroinflammation and fibrosis, improvement in health-related quality of life and the reduction in HCC risk, all of which enhance patient survival (Patel et al. 2006). We know that HCC primarily develops in HCV-infected patients with cirrhosis. In order to reduce the risk of developing HCV-related HCC, the aim would be to provide treatment to eradicate HCV prior to the development of cirrhosis. Indeed, studies have shown that successful viral clearance with antiviral therapy results in clinical and histological improvement in the vast majority of patients (Marcellin et al. 1997), with an associated reduction in the risk of subsequent development of cirrhosis and HCC (Pradat et al. 2007).
In a large multi-centre European study of largely non-cirrhotic (89 %) HCV-infected patients, successful viral eradication was associated with the progression to cirrhosis in only 2.3 % of patients with no patients developing HCC, whereas a failure to achieve viral clearance was associated with progression to cirrhosis in 20 % of patients and development of HCC in 4.2 % (Pradat et al. 2007). In another study of HCV-infected patients, the majority of whom (90 %) did not have cirrhosis, successful viral clearance with interferon therapy was associated with a reduction in the risk of HCC development from 2.31 to 0.24 %/year (Maruoka et al. 2012). This study also showed that patients who failed to achieve viral eradication despite antiviral therapy had the same risk of subsequent HCC development as patients who did not receive antiviral therapy (Maruoka et al. 2012).
Even in non-cirrhotic patients who do not achieve viral clearance with interferon therapy, the provision of long-term interferon monotherapy appears to reduce the risk of subsequent development of HCV-related HCC. In a retrospective study, patients with genotype 1 HCV infection who failed previous therapy with interferon, 93 % of whom did not have cirrhosis at the time of treatment, receiving a further 48 weeks or more of interferon monotherapy was associated with a reduction in the 10-year incidence of HCC from 16.4 to 11.5 % (Takeyasu et al. 2012).
6 Antiviral Treatment for Hepatitis C
A vaccine for the prevention of HCV is not yet available, and therefore, the only effective means to prevent the development of liver cirrhosis and HCC is with antiviral therapy. The aim of such treatment is to clear the virus, thereby halting the progression of liver injury and fibrosis. The currently available standard of care in the treatment for chronic HCV infection comprises the combination of pegylated interferon alpha and ribavirin. However, the advent of direct-acting antiviral (DAA) drugs has vastly changed the landscape of antiviral therapy for chronic HCV and may provide a more effective approach to the long-term prevention of HCV-associated HCC.
6.1 Standard of Care Therapy
The current standard of care for the treatment for HCV infection consists of a combination of subcutaneous pegylated interferon alpha given weekly and daily oral ribavirin (Fried et al. 2002). The two forms of pegylated interferon currently approved for HCV treatment are pegylated interferon alpha-2a (Pegasys, Hoffmann-La Roche, Nutley, NJ) and pegylated interferon alpha-2b (Pegintron, Merck Sharp and Dohme, Whitehouse Station, NJ), with no statistically significant difference in efficacy seen between the two forms (McHutchison et al. 2009b). Interferon alpha exerts its immunoregulatory properties by binding to interferon alpha 1 and 2 cell surface receptors to induce JAK-STAT signal transduction. Upon ligand binding, receptor-associated Janus kinase (JAK) tyrosine kinases are activated, leading to the phosphorylation and activation of members of the signal transducers and activators of transcription (STAT) family of transcription factors (Kisseleva et al. 2002). These transcription factors then translocate to the cell nucleus where they transcribe several genes involved in cell cycle regulation, apoptosis and promotion of an antiviral state within hepatocytes (Thomas et al. 2003). Besides the direct effect against virally-infected host cells, interferon alpha is also involved in modulating the immune system by enhancing the CD8+ cytotoxic T cell response against infected hepatocytes, as well as promoting the proliferation of B cells to augment the production of antibodies against HCV (Thomas et al. 2003).
Ribavirin (Copegus, Hoffmann-La Roche, Nutley, NJ; Rebetol, Merck Sharp and Dohme, Whitehouse Station, NJ) is a synthetic guanosine nucleoside analogue which has in vitro activity against a range of RNA and DNA viruses (Patterson and Fernandezlarsson 1990). When used as monotherapy in patients with chronic HCV, ribavirin was noted to reduce serum aminotransferase levels without having an antiviral effect (Dibisceglie et al. 1995). The precise mechanism by which ribavirin produces its antiviral effect when combined with pegylated interferon is unknown; however, there are a number of hypotheses. Ribavirin is administered as an orally active pro-drug, which is metabolized within the body into a form that resembles purine RNA nucleotides, thus enabling the incorporation of ribavirin into the viral genome during RNA replication. This induces hypermutation of the viral RNA genome resulting in viral lethality (Crotty et al. 2002). Ribavirin also inhibits the viral RNA-dependent RNA polymerase that is essential for HCV replication and it is also an inosine monophosphate dehydrogenase inhibitor. Inhibition of this intracellular enzyme leads to the depletion of intracellular GTP levels, thus decreasing viral protein synthesis and reducing RNA genome replication (Streeter et al. 1973).
6.2 Predictors of Pegylated Interferon/Ribavirin Antiviral Response
A successful response to treatment, which effectively equates to permanent viral eradication, is defined as the absence of HCV RNA in the serum 24 weeks after the completion of antiviral therapy and is referred to as a sustained virological response (SVR) (Pradat et al. 2007). An undetectable HCV RNA in the serum at week 4 of treatment, known as a rapid virological response (RVR), is predictive of a highly favourable outcome, with over 86 % of patients achieving an RVR subsequently attaining an SVR (Yu et al. 2007). If viral eradication is achieved prior to the development of cirrhosis, and no other hepatotoxic factors were present, hepatic fibrosis is expected to improve and the patient’s risk of developing HCC returns to that of the baseline population.
There are a number of host and viral factors that can predict antiviral treatment response prior to commencing therapy, with the most reliable of these being the HCV genotype. Patients infected with genotypes 2 and 3 attain much higher viral clearance rates than genotype 1–infected patients (Hadziyannis et al. 2004).
Single nucleotide polymorphisms at different loci within the IL28B gene on chromosome 19, which encodes interferon lambda 3, have also recently been shown to be strongly associated with response to interferon therapy, especially for individuals infected with genotype 1 HCV. Compared to patients possessing CT and TT polymorphisms at the rs12979860 locus of this gene, patients with a CC polymorphism are twice as likely to achieve viral clearance with pegylated interferon/ribavirin treatment (Ge et al. 2009).
In addition to the HCV genotype and IL28B gene polymorphisms, a low pre-treatment HCV viral load (<600,000 IU/mL) also predicts for a favourable outcome to antiviral treatment (Fried et al. 2002). Other factors that predict a poor response to treatment with pegylated interferon/ribavirin include vitamin D deficiency, the presence of insulin resistance or a body mass index of more than 30 kg/m2 (Bressler et al. 2003; Romero-Gomez et al. 2005), and the presence of cirrhosis or decompensated liver disease (Fried et al. 2002; Manns et al. 2001).
Although pegylated interferon and ribavirin treatment offers permanent eradication of the virus from chronically infected individuals, it is extremely expensive and is associated with a significant number of side effects. Furthermore, overall cure rates using the standard regimen are disappointing in patients infected with genotype 1 in whom SVR rates of 40–50 % are achieved compared to 70–80 % in patients with genotypes 2 and 3, and approximately 70 % in patients with genotype 4 (Chevaliez and Pawlotsky 2007; Khuroo et al. 2004).
6.3 Direct-Acting Antiviral Agents
Significant advances have recently been made in the treatment for chronic HCV infection. The development of direct-acting antiviral agents (DAAs), which target specific HCV proteins crucial for the replication cycle of HCV, have resulted in significant improvements in achieving SVR. This has been most beneficial for patients infected with HCV genotype 1, patients with poor pre-treatment prognostic factors, and non-responders and relapsers to pegylated interferon and ribavirin therapy. Some of the DAAs that are currently in use or in development are listed in Table 1.
Table 1
HCV direct-acting antiviral agents
DAA | Type of inhibitor | Study phase | Genotypic activity | Barrier to resistance | Company |
---|---|---|---|---|---|
Telaprevir | NS3/4A protease (1st generation, 1st wave) | Phase III | 1 | Low | Vertex |
Boceprevir | NS3/4A protease (1st generation, 1st wave) | Phase III | 1 | Low | Merck Sharp and Dohme |
Danoprevir | NS3/4A Protease (1st generation, 2nd wave) | Phase IIb | 1 | Low | Roche/Genentech |
Asunaprevir | NS3/4A protease (1st generation, 2nd wave) | Phase III | 1 | Low | Bristol-Myers Squibb |
Vaniprevir | NS3/4A protease (1st generation, 2nd wave) | Phase IIb | 1 | Low | Merck Sharp and Dohme |
MK-5172 | NS3/4A protease (2nd generation) | Phase II | 1, 2, 3 | Moderate | Merck Sharp and Dohme |
Mericitabine | NS5B polymerase (nucleoside) | Phase IIb | 1, 2, 3 | High | Roche/Genentech |
Sofosbuvir | NS5B polymerase (nucleoside) | Phase III | 1, 2, 3 | High | Pharmasset/Gilead |
BI207127 | NS5B polymerase (non-nucleoside) | Phase IIb | 1 | Low | Boehringer Ingelheim |
VX-222 | NS5B polymerase (non-nucleoside) | Phase II | 1 | Low | Vertex |
ABT-072 | NS5B polymerase (non-nucleoside) | Phase II | 1 | Low | Abbott |
Daclatasvir | NS5A | Phase IIb | 1 | Low | Bristol-Myers Squibb |
Protease inhibitors: The first NS3/4A protease inhibitors to be licensed include telaprevir (Incivek, Vertex) and boceprevir (Victrelis, Merck Sharp and Dohme). Both are only effective in patients infected with genotype 1 HCV. The addition of these protease inhibitors to standard of care therapy significantly improves SVR rates in both naïve patients and those who have failed to achieve an SVR with previous therapy.
In a large phase III, randomized, double-blind, placebo-controlled trial of treatment-naïve patients infected with genotype 1 HCV, telaprevir was administered for 12 weeks together with pegylated interferon and ribavirin, after which pegylated interferon and ribavirin were continued for a further 12–36 weeks (Jacobson et al. 2011). Telaprevir increased SVR rates from 44 to 75 % compared to standard therapy alone. Even for patients with high pre-treatment viral loads, SVR was improved from 36 to 74 % with the addition of telaprevir (Jacobson et al. 2011). In another large phase III study, after a 4-week “lead-in” phase of pegylated interferon and ribavirin, boceprevir was added and therapy with all three drugs continued for a total of 28–48 weeks (24–44 weeks of boceprevir) (Poordad et al. 2011). The addition of boceprevir enhanced SVR rates from 38 to 66 % compared to pegylated interferon and ribavirin alone. In both studies, the SVR rates in treatment-naïve cirrhotic patients were also improved from 33 to 62 % with the addition of telaprevir (Jacobson et al. 2011), and 38–52 % with the addition of boceprevir (Poordad et al. 2011).
For patients who experienced virological relapse after becoming HCV RNA PCR negative with previous pegylated interferon and ribavirin, the addition of telaprevir for the first 12 weeks of a 48-week course of pegylated interferon and ribavirin treatment improved SVR rates from 24 % to over 80 % compared to pegylated interferon and ribavirin alone for 48 weeks (Zeuzem et al. 2011a). The addition of boceprevir to pegylated interferon and ribavirin for 44 weeks after the 4-week “lead-in” phase also resulted in an improvement in SVR from 29 to 75 % compared to pegylated interferon and ribavirin alone for 48 weeks (Bacon et al. 2011). For treatment-experienced cirrhotic patients, the results were also encouraging for relapsers, with SVR rates increasing from 13 to 84 % with telaprevir (Zeuzem et al. 2011a), and from 20 to 83 % with boceprevir (Bacon et al. 2011).
Common side effects of telaprevir therapy include skin rash, anaemia and gastrointestinal symptoms, while common side effects of boceprevir include anaemia and dysgeusia (Jacobson et al. 2011; Poordad et al. 2011). Ribavirin remains crucial to antiviral therapy with the trials employing telaprevir and boceprevir to date showing that even in the era of potent DAAs, SVR and virological relapse rates were suboptimal without the use of full-dose ribavirin (Kwo et al. 2010; McHutchison et al. 2009a). Although potent inhibition of HCV replication is achieved with these agents, the rapid development of drug-resistant HCV variants has also been noted, with the potential to confer cross-resistance to other protease inhibitors (Halfon and Locarnini 2011). As such, these agents should not be used as monotherapy.
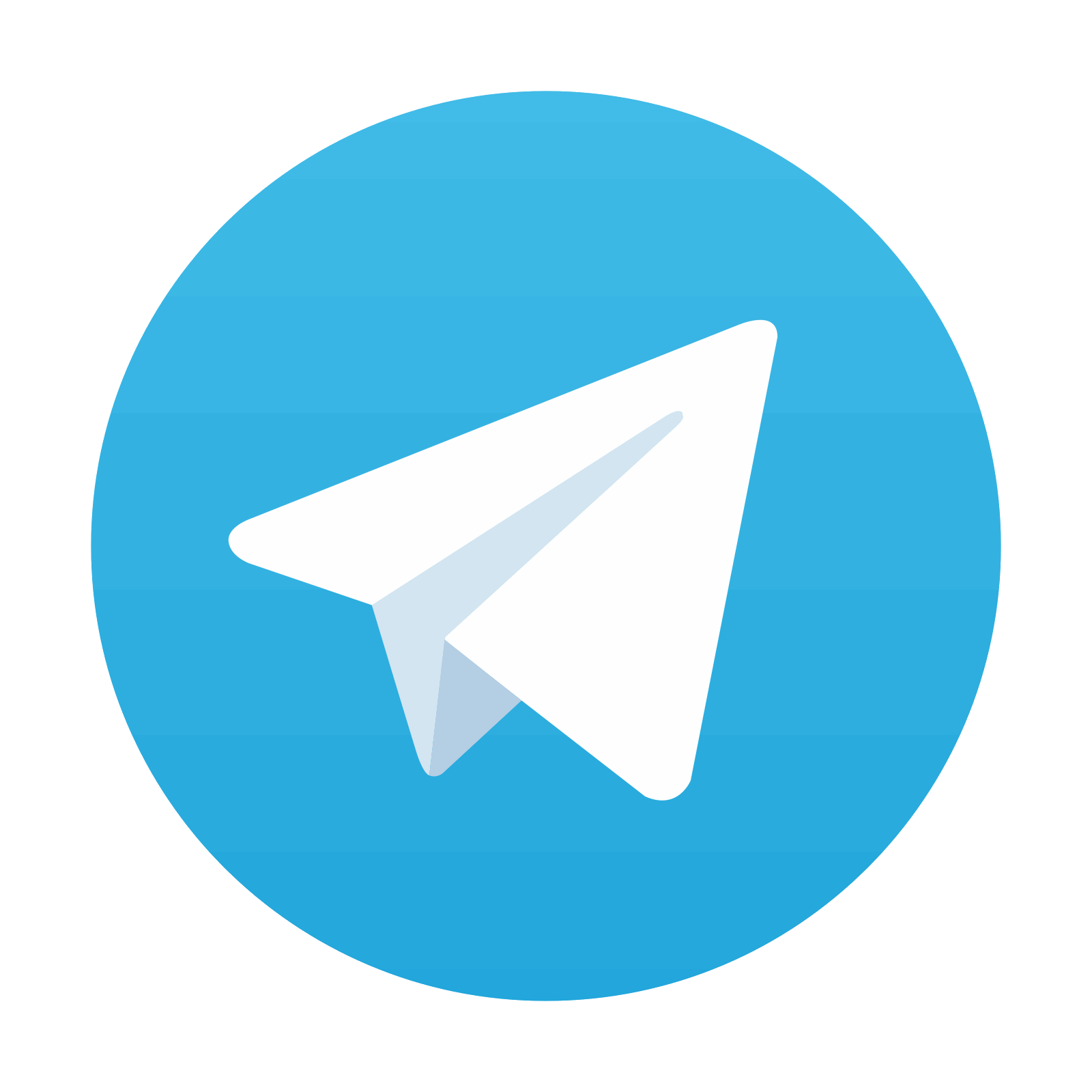
Stay updated, free articles. Join our Telegram channel

Full access? Get Clinical Tree
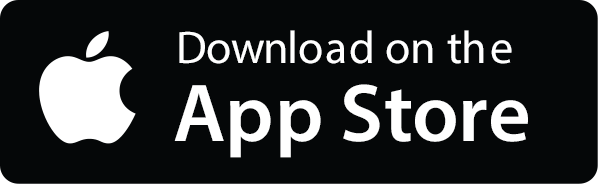
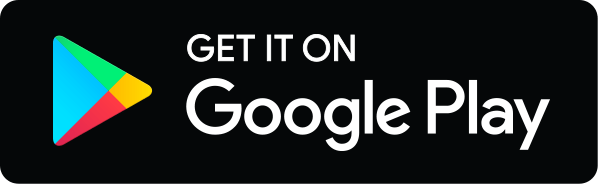