Type
Advantage
Disadvantage
Developmental stage
References
Live vector-based
Highly immunogenic customizable
Vector-specific neutralizing antibodies or pre-existing vector-specific immunity, safety concerns, flu-like adverse effects
ADXS11-001 in phase II Trial
MVA-E2 in phase II trial
Corona Gutierrez et al. (2004)
TA-HPV completed phase I/II trial
Kaufmann et al. (2002)
TG4001-R3484 completed phase II trial
Brun et al. (2011)
Peptide-based
Safe, easy to produce, stable
HLA restriction,
Poorly immunogenic
HPV-16 E7 for HLA-A*0201 completed phase 1 Trial
Overlapping peptides
Clinical trials
Protein-based
Safe, easy to produce, stable
Poorly immunogenic
HspE7 completed
phase I and II trials
TA-CIN completed
phase II trials
Daayana et al. (2010)
DC-based
Highly immunogenic
Individualized and labor intensive, potentially oncogenic
HPV-16 and -18 E7 pulsed DC vaccine completed phase 1 trials
Wang et al. (2009)
DNA-based
Easy to produce, stable, prolonged expression of antigens, capacity for repeated administration
Poorly immunogenic, potentially oncogenic
ZYC101a completed phase II trial
VGX-300 in phase II trials
Inovio (2012)
pNGVL4a-Sig/E7/HSP70 completed phase I trial
Trimble et al. (2009)
Data regarding efficacy of each of the two vaccines have recently been published in 4 year follow-up studies of women from each vaccine’s phase 3 clinical trials. In a 4-year trial with 17,622 women aged 16–26, the quadrivalent vaccine was found to be effective in preventing the development of lesions caused by HPV-6, 11, 16, and 18: 96 % for CIN1 (cervical intraepithelial neoplasia 1), 100 % for VIN1 and VAIN1 (vulva and vaginal intraepithelial neoplasia 1), and 99 % for condyloma (Dillner et al. 2010). However, protection against HPV strains not covered by the quadrivalent vaccine was less robust; efficacy against any lesion regardless of HPV type was 30, 75, 48, and 83 % for cervical, vulvar, vaginal intraepithelial neoplasia, and condyloma, respectively, suggesting that the quadrivalent vaccine has low cross-reactivity for other HPV strains. The bivalent vaccine also had its own four-year follow-up study. In a trial enrolling 18,644 women ages 15–25, the bivalent vaccine was found to be 100 % effective in preventing CIN3 and AIS caused by HPV strains 16 and 18 and 93.2 % effective in protecting against all CIN3 lesions regardless of HPV type. However, in a concurrent study analyzing the same data, the cross-protective efficacy of the bivalent vaccine in preventing CIN2 + lesions caused by non-HPV-16 and -18 strains was variable among HPV naïve patients who received all 3 doses of vaccine: 84.3 % effective for HPV-31, 59.4 % effective for HPV-33, and statistically insignificant for HPV-39, 45, 52, 58, 59, and 68 (Wheeler et al. 2012). Therefore, the cross-reactivity of the bivalent vaccine, like that of the quadrivalent vaccine, is also limited in nature. The observation that the bivalent vaccine is more effective in preventing AIS and CIN3 + lesions caused by any HPV infection but not as effective in preventing CIN2 + lesions caused by non-HPV-16 and -18 strains suggests that a significant proportion of higher grade lesions are caused by HPV-16 and -18 relative to other strains (Wheeler et al. 2012). Efficacy of the bivalent vaccine in preventing CIN2 + and CIN3 + lesions in both studies was found to be highest in the 15–17 age group and declined with increasing age, supporting the current recommendation of vaccinating at younger ages (Lehtinen et al. 2012; Wheeler et al. 2012).
Both commercially available vaccines are currently marketed as a 3-dose regimen. However, one recent randomized trial involving 960 subjects compared the bivalent vaccine at either 2 doses or the standard 3-dose regimen. The 3-dose regimen was considered superior if there was more than a twofold difference in the ratio of geometric mean antibody titers (GMTs). Results showed that 2 doses of the bivalent vaccine elicited antibody responses both at one month and 24 months after vaccination that were non-inferior to those of the same concentration vaccine given in the standard 3-dose regimen. This suggests that 2 doses of the current bivalent vaccine would yield equivalent protection to the standard 3-dose regimen up to 24 months post-vaccination (Romanowski et al. 2011); however, the longevity of protection beyond this has yet to be determined.
Although the primary purpose of commercial HPV vaccines is to prevent cervical cancer in women, the quadrivalent vaccine may be used to prevent condyloma and anogenital lesions, caused by HPV 6 and 11, in males. Males may also benefit from protection against HPV-16 and -18 that can cause cancers of the penis, anus, and oropharynx (Giuliano et al. 2011). In a randomized, placebo-controlled, double-blind trial of 4065 males age 16–26, the efficacy of the quadrivalent vaccine in preventing external genital lesions was tested. In the intention to treat group, comprised of subjects who may or may not have been seropositive for HPV before the trial and received at least one dose of either vaccine or placebo, 36 external genital lesions were seen in the HPV-vaccinated group compared to 89 lesions in the placebo group, yielding an overall efficacy of 60.2 % (95 % CI, 40.8–73.8). In the per-protocol population of males, who were seronegative before vaccination and completed all 3 doses of quadrivalent vaccine, the efficacy against HPV-6, 11, 16, and 18 lesions was 90.4 % (95 % CI, 69.2–98.1), suggesting that the quadrivalent vaccine is effective in preventing HPV-associated genital lesions in males (Giuliano et al. 2011).
3 Second Generation of Preventive Vaccine Development
The clinical results offered by available HPV vaccines are excellent for the types targeted, but issues remain. Eighty-five percent of the burden of cervical cancer is in developing countries (Jemal et al. 2011). Not only are most residents in these developing countries unable to afford the current commercial vaccines, many do not even live in areas which have the capability to store and distribute such vaccines (through cold chains). Another limiting factor of the available preventive vaccines is their limited cross-reactivity to many oncogenic HPV strains. Patients vaccinated with available vaccines will be protected from HPV-16 and -18, which cause more than 70 % of cervical cancers, but there are a dozen other oncogenic strains against which patients have limited or no defense. Broader coverage against oncogenic HPV strains is a desirable attribute in future generations of HPV vaccines. Therefore, efforts to improve upon the already available HPV vaccines have much merit.
There have been several approaches for the next generation of preventative HPV vaccines, which include as follows: (1) more multivalent VLP-based vaccines, (2) L1-based capsomers, (3) L2-based vaccines, and (4) chimeric L1-L2 vaccines. Figure 1 summarizes the various strategies in the current as well as the next generation of preventive HPV vaccines. Multivalent VLP vaccines build upon the original idea used to make commercially available bivalent and quadrivalent vaccines (Cervarix™ and Gardasil®). If VLPs of high-risk strains of HPV-16 and HPV-18 are protective, then why not add more VLPs in order to broaden the coverage? Currently, there are ongoing trials to compare nonavalent VLP vaccines to the quadrivalent vaccine (Merck 2011). The addition of more VLPs will likely increase the costs of production; however, there are several efforts to cost-effectively produce HPV VLPs inside of bacteria.
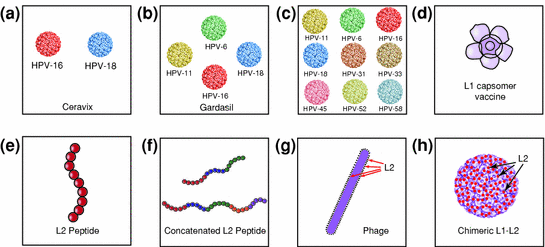
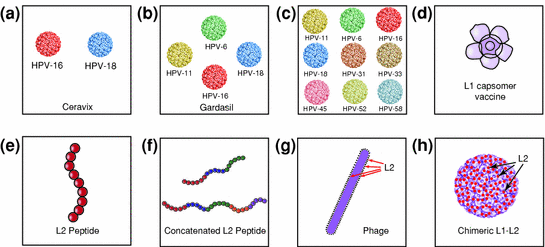
Fig. 1
Schematic diagram to depict the next generation of preventive HPV vaccines. a Cervarix composed of HPV-16 and HPV-18 VLPs. b Gardasil composed of HPV-6, HPV-11, HPV-16, and HPV-18 VLPs. c Multivalent VLP vaccines composed of HPV-6, HPV-11, HPV-16, HPV-18, HPV-31, HPV-33, HPV-45, HPV-52, and HPV-58 VLPs. d L1 capsomer vaccine. e L2 peptide vaccine. f Concatenated L2 peptide vaccine. g Phage vaccine with L2 on the surface of the phage. h Chimeric L1-L2 VLP vaccine with L2 on the surface
Another potential avenue for new preventive vaccines involves the creation of L1 capsomer vaccines, a potential cost effective alternative to VLP vaccines. Capsomers are basic structural components of viral capsids, whereas HPV L1 VLP is composed of 360 copies of the L1 protein, only five L1 monomers are needed to assemble a pentavalent capsomer. Additionally, these capsomer proteins can be made in bacteria and thus are cheaper to produce than VLPs made in insect or yeast cells. L1 capsomer proteins made in E. coli, recombinant measles virus, or recombinant Salmonella enterica serovar Typhimurium have successfully induced protective antibodies in preclinical models and these genetically modified live vaccines might be another approach to lower costs and perhaps reduce the number of immunizations (Chen et al. 2000; Fraillery et al. 2007; Li et al. 1997; Rose et al. 1998).
Another approach for second generation preventive vaccines involves the use of L2 instead of L1 VLPs. L2 is highly conserved among different HPV types and the L2 from one strain of HPV can possibly induce broader protection through cross-neutralizing antibodies, even across species. It has been shown that immunization of animals with the amino-terminal peptide of L2 produced in E. coli elicited neutralizing antibodies that protect against challenge with cognate papillomavirus types in vivo (Embers et al. 2002; Gaukroger et al. 1996) cross-neutralize heterologous types in vitro (Kawana et al. 1999; Pastrana et al. 2005; Roden et al. 2000), and confer cross-protection in vivo (Gambhira et al. 2007).
L2 has also been used in other forms. In a preclinical experiment, concatenated multitype L2 fusion proteins were used in different combinations (L2 residues 11–200 from 3 HPV types (6, 16, and 18), L2 residues 11–88 from 5 HPV types (1, 5, 6, 16, and 18), or L2 residues 17–36 of five cutaneous, two mucosal low-risk, and 15 oncogenic HPV types) (Jagu et al. 2009). Vaccination in both mice and rabbits with the concatenated multitype L2 with different adjuvants elicited higher neutralizing antibody titers than in animals vaccinated with only HPV-16 amino-terminal L2 polypeptides, HPV-16 L1 VLP, Gardasil®, or the negative control (Jagu et al. 2009). Mice vaccinated with these concatenated multitype L2 fusion proteins also had immunity against challenge with HPV-16 pseudovirions 4 months later. Furthermore, the HPV-16 L2 peptides were able to generate antisera that neutralized HPV-18, 31, 45, and 58, thus confirming the cross-reactivity of L2 (Jagu et al. 2009). Generally, L2 is less immunogenic than L1, and this limitation prompted Tumban et al. 2011 to create a vaccine consisting of PP7 bacteriophage VLPs that displayed the neutralizing epitope from L2 proteins of 8 different HPV types on their surfaces (Tumban et al.). Mice vaccinated twice with HPV-16 L2 peptide displaying PP7 and HPV-18 L2 peptide displaying PP7 produced GMTs ranging from 104 to 105 of broadly reactive anti-L2 IgGs. Additionally, these anti-L2 antibodies protected against vaginal challenge with HPV-16 or -18 pseudovirions and cross-reacted with synthetically engineered HPV L2 peptides (Tumban et al. 2011). Although they have the potential to confer greater cross-reactivity, the immunogenicity of L2 proteins is low and more potent adjuvants and display technologies are currently being explored.
The advantages of highly immunogenic L1 vaccines and the broad cross-protection mediated by L2 can potentially be combined in the form of chimeric L1-L2 VLPs. Since L2 is a less abundant protein that is found predominantly in the interior of the viral particle, replacing some regions of the VLP surface normally occupied by L1 immunodominant epitopes with a neutralizing epitope of L2 may generate more immunogenic, cross-protective immune responses against multiple HPV genotypes. The surface expression of the neutralizing epitope of L2 on L1 VLP in chimeric models is essential since VLPs with L1 and L2 s in their normal positions do not readily generate L2 antibodies. Immunization of rabbits with chimeric L1-L2 VLPs with L2 peptides on their surface was shown to induce not only antibodies neutralizing HPV-16 but also antibodies cross-neutralizing HPV-18, 31, 52, 58 pseudovirions (Kondo et al. 2008). Additionally, vaccination with a chimeric HPV-16 L1-L2 VLP vaccine in both rabbits and mice also exhibited cross-neutralization to high-risk and low-risk HPV types evolutionarily divergent from HPV-16 (Schellenbacher et al. 2009). The inclusion of the immunodominant neutralizing epitopes of L2 into the L1 VLPs represents a promising direction for the next generation of preventative HPV vaccines to induce broad-spectrum neutralizing antibodies against HPV.
4 Strategies of Therapeutic Vaccines in Clinical Development
4.1 Concept and Goals of Therapeutic Vaccines
HPV preventive vaccines, though effective in blocking HPV infections, cannot eliminate established HPV infections or HPV-associated lesions and thus, have no therapeutic activity (Lin et al. 2010). Given the fact that almost all cervical cancers are caused by high-risk HPVs, therapeutic vaccines targeting HPVs offer great potential for highly specific eradication of HPV-infected cells and HPV-associated tumors through immunotherapy. As discussed in the preceding chapter, the progression of latent HPV infections into invasive cancer takes many years and fortunately, most women clear their infections. However, if women with high-grade CIN lesions suffer few symptoms and are not screened, they may be unaware that cancer is progressing inside of their cervix. In a prolonged chronic infection, there is a considerable window for secondary preventive treatment for infections caught by cytologic screening/HPV DNA testing. Effective therapeutic HPV vaccines that can actively attack HPV-infected cells during this long phase of tumor progression are greatly needed to prevent the development of and to treat advanced cancer. If therapeutic vaccines can eradicate transformed cells early, the morbidity and mortality associated with HPV-associated malignancies and invasive treatments may dramatically diminish.
Many different platforms have been used in the development of therapeutic HPV vaccines, each in various phases of testing and clinical trials. In order to compare the potential of various candidates, one has to keep in mind the characteristics of an ideal therapeutic vaccine. These qualities include: (1) safety, (2) the ability to trigger effective HPV-antigen-specific cytotoxic T-cell-mediated responses that target the lesion, (3) specificity for tumor cells, (4) long duration of efficacy, (5) cheaper mass production and storage, and (6) fewer number of required doses. The ability of a therapeutic vaccine to trigger specific T-cell-mediated killing is essential to a vaccine’s efficacy in targeting transformed cells vs. healthy cells. Reduced cost of production and storage is a highly desirable trait that will increase access to cancer treatments for patients worldwide. Currently, the compliance rate of patients who start and complete all three shots of either available bivalent or quadrivalent vaccine is roughly 25 % [for review, see Trimble and Frazer (2009)]. Thus, simplicity of delivery will greatly increase the compliance rates of a therapeutic vaccine.
As aforementioned in Sect. 1, E6 and E7 are ideal targets for therapeutic vaccines. There has been much research and development in the field of therapeutic HPV vaccines, including live-vector-based, peptide-based, protein-based, dendritic cell-based, DNA-based, and combination vaccines targeting E6 and/or E7 antigens.
4.2 Live Vector-Based Therapeutic HPV Vaccines
The concept of live vector vaccines encompasses the use of bacterial and viral vectors, which effectively infect host cells and provide a source of E6 and/or E7 antigens for antigen-presenting cells. Several bacterial vectors have been explored for therapeutic HPV vaccines, and among these, L. monocytogenes, a gram-positive intracellular bacterium, has generated significant interest since it is able to invade macrophages and evade phagocytosis within the phagosome using the pore-forming toxin, listeriolysin O (LLO). Antigens from L. monocytogenes infection can be processed in both MHC class I and II pathways. L. monocytogenes-based HPV E7 vaccines have been shown to stimulate a greater number of E7-specific CD8+ T cells and resolve solid tumors in both transgenic and wild type mice (Gunn et al. 2001; Hussain and Paterson 2004; Sewell et al. 2004; Souders et al. 2007). There has been translation of this research into clinical trials. ADXS11-001, formerly known as Lovaxin C or Lm-LLO-E7, is a live, attenuated L. monocytogenes bacterial vector secreting HPV-16 E7 fused to LLO. In phase I trials, ADXS11-001 was found to be safe and well-tolerated with a dose limiting toxicity linked to flu-like symptoms (Maciag et al. 2009; Radulovic et al. 2009). Currently, ADXS11-001 is being studied in two clinical trials with active enrollment. The primary goal of one randomized, single blind, placebo-controlled phase 2 study is to determine whether 3 doses of ADXS11-001 can safely reverse disease in subjects with CIN2/3 in whom surgery is indicated (NCI 2012d). Another ongoing phase 2 trial involves testing the safety and efficacy of ADXS11-001 in treating and increasing the one-year survival rate of subjects with persistent or recurrent carcinoma of the cervix (NCI 2012b).
In addition to bacteria, live viral vectors have been investigated due to their high immunogenicity. There have been several viral vectors used to deliver HPV E7 antigens, but the Vaccina virus, an enveloped, double-stranded DNA virus within the Poxviridae family, represents a particularly promising viral vector of interest because of its large genome and high infectivity. A recombinant Vaccinia virus expressing HPV-16 and HPV-18 E6/E7 antigens (TA-HPV) has been evaluated in phase I/II clinical trials in patients with early-stage cervical cancer (Kaufmann et al. 2002), late-stage cervical cancer (Borysiewicz et al. 1996), vulvar intraepithelial neoplasia (Davidson et al. 2003), and vaginal intraepithelial neoplasia (Baldwin et al. 2003). TA-HPV was found to be safe and potent in stimulating vaccinia-specific antibody and HPV-antigen-specific CTL responses (Adams et al. 2001; Borysiewicz et al. 1996; Kaufmann et al. 2002).
Another vaccinia-based therapeutic HPV vaccine is MVA-E2, an attenuated, recombinant vaccinia virus encoding bovine papillomavirus type 1 (BPV-1) E2 (Corona Gutierrez et al. 2004). In a phase I/II clinical trial, four intrauterine doses of MVA E2 led to significant effects in patients with CIN lesions. It was reported that 34 of 36 subjects in the trial showed complete CIN lesion regression, while the other 2 subjects had a reduction in the lesion grade from CIN3 to CIN1 (Corona Gutierrez et al. 2004). Several possible explanations may account for the observed therapeutic effects. First, in the early stage of infection, for example CIN lesions when the HPV genome in infected cells has not yet been integrated into the host genome, infected cells may still express the E2 antigenic peptide on their surfaces—enabling E2-specific CTLs, generated through treatment, to hone in and attack. However, it is not clear whether the BPV-1 E2-specific CTLs generated through treatment with MVA-E2 are capable of recognizing HPV E2 targets on the infected cells of the CIN lesions. Second, the E2 from MVA-E2 may act as a transcriptional repressor that inhibits the expression of E6 and E7. Lastly, local administration of a highly immunogenic live vector, such as MVA-E2, may stimulate stronger immune responses in the microenvironment of HPV-associated lesions. While the initial observation is encouraging, further validation is required to establish this approach for the control of CIN lesions.
Another modified vaccinia-based vaccine uses the Ankara vector to express HPV-16 E6 and E7 antigens and adjuvant IL-2 (TG4001/R3484). In phase II clinical trials, TG4001/R3484 was found to be both safe and encouraging in generating clinical responses in women with HPV-16-positive CIN 2/3. Ten of 21 women in the trial no longer had detectable levels of CIN 2/3 six months after vaccination. At the twelve-month follow-up, no relapse or HPV-16 persistence was observed in these women (Brun et al. 2011). Although live vector vaccines have shown promising results, they inherently pose a potential safety risk, particularly to immunocompromised individuals. Live vectors may also face limited capacity for repeated administration due to induction of vector-specific neutralizing antibodies and/or pre-existing vector-specific immunity.
4.3 Peptide-Based Therapeutic HPV Vaccines
Short peptides of HPV antigens along with adjuvant can be delivered to dendritic cells, which then present those antigens onto MHC class I molecules and thus activate antigen-specific T-cell immunity. Production of these short-peptide vaccines involves the prior identification of specific CTL and CD4+ T helper epitopes of HPV antigens. Peptide-based vaccines are advantageous in that they are stable, easy to produce, and have a high safety profile. Current research focuses on addressing the main limitations of these vaccines—namely low immunogenicity and MHC restriction.
The immunogenicity of HPV peptide-based vaccines can be enhanced with adjuvants including immunoglobulin G fragment (Qin et al. 2005), streptavidin fused to the extracellular domain of murine 4-1BBL (Sharma et al. 2009), dendritic cell stimulatory cytokine bryostatin (Yan et al. 2010) and toll like receptor (TLR) agonists (Daftarian et al. 2006; Wu et al. 2010; Zhang et al. 2010; Zwaveling et al. 2002). For example, a prime boost regimen of HPV-16 E7 (a.a. 43–77), which contains both CTL and Th epitopes, adjuvanted with TLR9 agonist CpG oligodeoxynucleotide, was shown to enhance priming of E7-specific CD4+ and CD8+ T cells and produce potent antitumor effects in vaccinated mice (Zwaveling et al. 2002). MHC restriction is another problem that has to be overcome. Since HLA-A*0201 is the most common human MHC class I molecule, peptide-based therapeutic HPV vaccine studies have focused on HPV-16 E7 HLA-A*0201 CTL epitopes. A phase I clinical trial found that a vaccine with HPV-16 E7 peptide specific for HLA-A*0201 along with adjuvant was able to stimulate an immune response in 10 of 16 HLA-A2-positive patients with cervical or vulva intraepithelial neoplasia (CIN/VIN) stage 2/3 and complete regression of CIN lesions in 3 of 18 patients (Muderspach et al. 2000). However, these peptide vaccines are only effective in patients with HLA-A*0201.
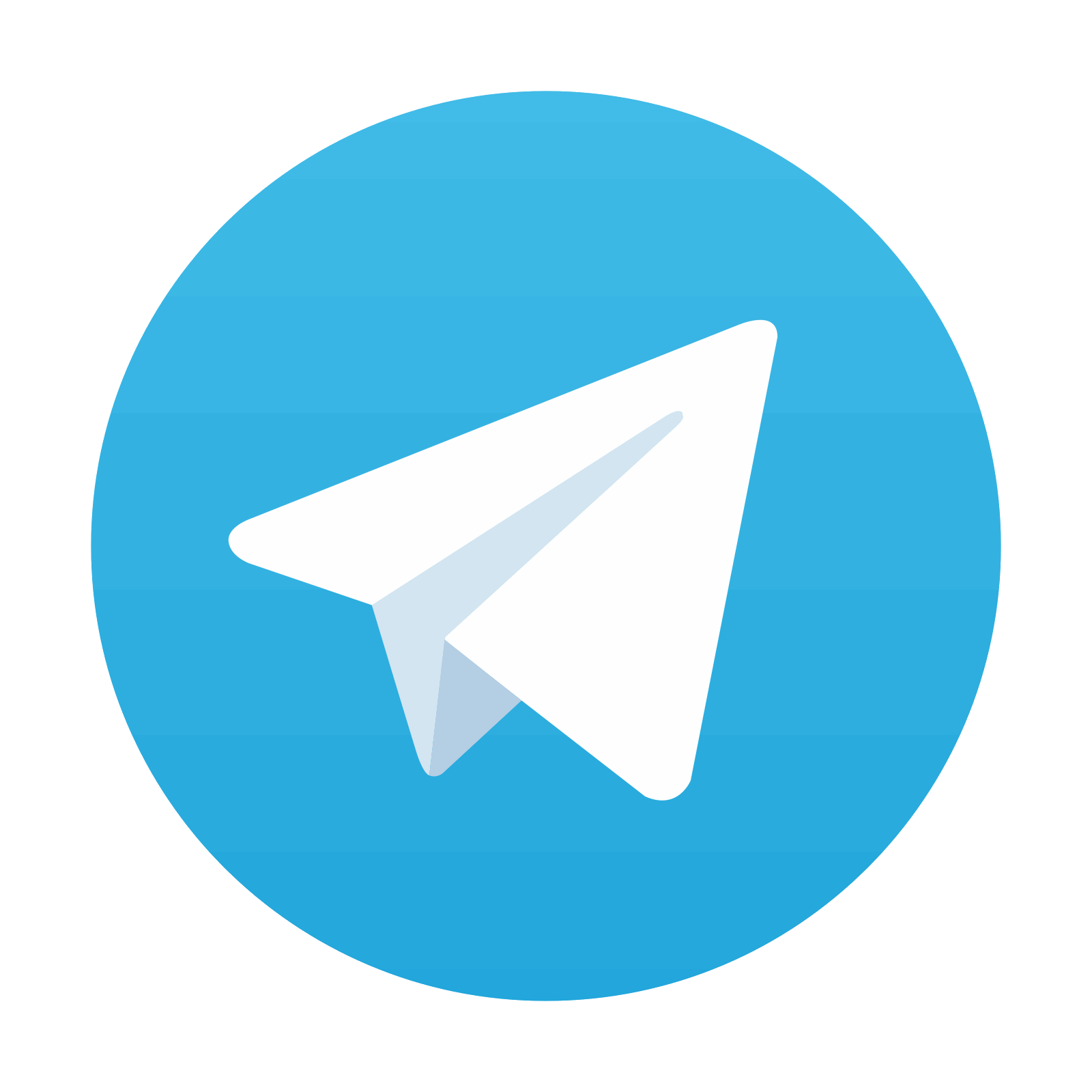
Stay updated, free articles. Join our Telegram channel

Full access? Get Clinical Tree
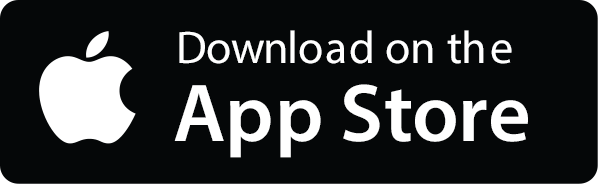
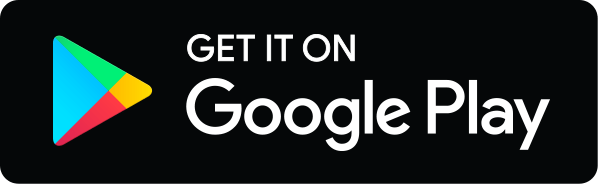