Neoplastic meningitis, commonly referred to as carcinomatosis meningitis, leukemic meningitis, or lymphomatous meningitis, is the third most common central nervous system metastatic complication of systemic cancer and is the most morbid of central nervous system metastases. The disease is challenging to treat for a variety of reasons, including challenges in making a diagnosis of neoplastic meningitis and lack of standardized treatment due to the paucity of clinical trials addressing treatment of neoplastic meningitis.
- •
Neoplastic meningitis (NM) is a disease that is under-recognized and consequently undiagnosed and treated.
- •
NM involves the entire neuraxis and as such any element of the central nervous system (CNS) (brain, cranial nerves, spinal cord, or exiting nerve roots) may be involved.
- •
In a patient with cancer with otherwise unexplained CNS dysfunction, consider NM.
- •
NM may be diagnosed clinically, radiographically, or pathologically (cerebrospinal fluid [CSF] flow cytometry or cytology).
- •
Epidural spinal cord compression (ESCC) essentially always presents with pain, and treatment is most effective with there is little to no neurologic compromise.
Neoplastic meningitis
Introduction
Carcinomatous meningitis (CM), or meningeal carcinomatosis, is a term that defines leptomeningeal metastases arising as a result of metastases from systemic solid cancers. Similarly, lymphomatous meningitis and leukemic meningitis result from CSF dissemination of lymphoma or leukemia. All 3 entities are commonly referred to as NM or leptomeningeal metastasis due to involvement of both the CSF compartment and the leptomeninges, comprised of the pia and arachnoid. Although NM is the third most metastatic complication of the CNS, NM is comparatively uncommon with 7000 to 9000 new cases diagnosed annually in the United States. The most common sources of systemic cancer metastatic to the leptomeninges are breast cancer, lung cancer, melanoma, aggressive non-Hodgkin lymphoma, and acute leukemia in that order. The majority of patients with NM present with neurologic dysfunction as well as evidence of other sites of systemic metastases. Neurologic dysfunction may involve the entire neuraxis although most commonly spinal cord symptoms and cranial neuropathies dominate. In some patients, cerebral hemisphere symptoms, such as confusion, may predominate. Because any site in the CNS may be involved, clinical manifestations may be challenging in patients with cancer and associated comorbidities. Consequently, a high clinical index of suspicion needs to be entertained so as to recognize the disease and determine the most appropriate therapy. Clinical syndromes that suggest NM include cauda equina syndrome (ie, asymmetric lower extremity weakness and dermatomal sensory disturbance), communicating hydrocephalus presenting with symptoms of raised intracranial pressure, and cranial neuropathies, most often involving oculomotor function and presenting with diplopia.
Diagnosis
Establishing a diagnosis of NM requires careful consideration of clinical findings such that any patient with cancer and a neurologic disturbance should be considered as potentially having NM ( Fig. 1 ). Neuroradiographic imaging (ie, MRI of brain or relevant spine) may suggest NM based on focal or diffuse leptomeningeal enhancement, subarachnoid or ventricular tumor nodules, and the frequent (30%–40%) coexistence of brain parenchymal metastases in instances of nonhematologic cancers (see Fig. 1 ). The reported rates of negative CSF imaging in patients with NM range from 30% to 70%, suggesting normal CNS imaging does not exclude a diagnosis of NM. Also, CSF analysis is crucial to diagnosing NM and in all patients some abnormality of CSF is apparent (ie, elevated opening pressure or protein, depressed glucose, or mild pleocytosis). Tumor assessment by CSF cytology in patients with solid cancers may be positive although the test has a high false-negative rate (approximately 50%) notwithstanding multiple sampling (2–3 samples), large volume (>10 mL) sent for analysis, and prompt presentation of the CSF to the laboratory. Rarely are CSF markers of cancer (ie, carcinoembryonic antigen) useful in diagnosing or managing patients with CM, except in instances of germ cell tumors where CSF markers (α-fetoprotein and β-human chorionic gonadotropin) often are useful. Because of the frequent absence of corroborating laboratory studies (ie, negative CSF cytology and neuroimaging), a diagnosis of NM may be presumed based only on clinical findings in a patient with cancer and nonspecific CSF abnormalities. Of greater use in patients with hematologic cancers is the increased sensitivity of CSF flow cytometry in demonstrating NM, and that requires comparatively small volumes of CSF for analysis (approximately 2 mL) ( Table 1 ).
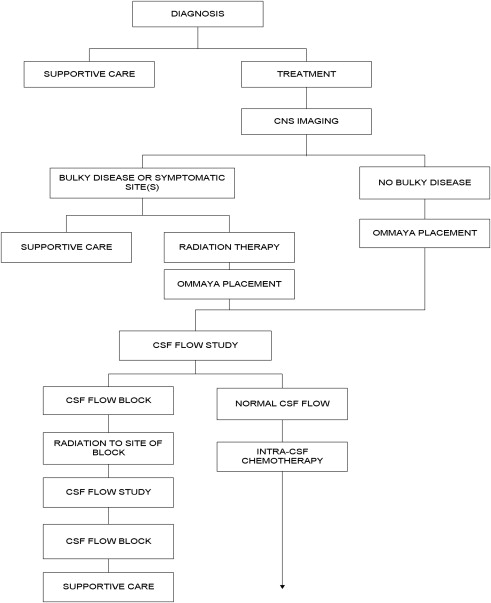
Negative CSF Cytology | Positive CSF Cytology | Total | |
---|---|---|---|
Negative flow cytometry | 287 (72%) | 21 (5%) | 308 (76%) |
Positive flow cytometry | 63 (16%) | 28 (7%) | 91 (23%) |
Total | 350 (88%) | 49 (12%) | 399 (100%) |
Treatment
Two challenges arise with respect to the treatment of NM: determining whom to treat, and, if CM-directed treatment is believed warranted, how to treat. Patients assessed as candidates for treatment include those with low tumor burden as reflected by independence in a performance and lack of major neurologic deficits, no evidence of bulky CNS disease by neuroimaging, absence of CSF flow block by radioisotope imaging, expected survival greater than 3 months, and limited extraneural metastatic disease. Many of these parameters require laboratory investigation, including the performance of neuraxis imaging (most commonly MRI with contrast) and a radioisotope CSF flow study (see Fig. 1 ). CSF flow studies, although recommended in guidelines, are infrequently used; however, they may assist in determining whether intra-CSF chemotherapy, if administered, distributes homogenously throughout the CSF or whether intra-CSF chemotherapy likely is confined to a single CSF compartment, resulting in failure to treat all sites of leptomeningeal disease as well as increasing the risk for treatment-related neurotoxicity. If, after clinical and laboratory assessment, intra-CSF chemotherapy treatment is believed warranted, a decision is made whether to treat by lumbar administration (intrathecal) or by way of a surgically implanted subgaleal reservoir and intraventricular catheter (ie, an Ommaya or Rickham reservoir system). Intralumbar treatment is convenient but suffers from the time required to perform, patient discomfort from the procedure, frequent need for performance by interventional radiology, failure to deliver drug to the thecal sac (10%–12% of intralumbar treatments do not enter the CSF compartment), limited distribution within the cranial CSF compartments when administering short half-life intra-CSF chemotherapy agents (methotrexate and cytarabine), and the apparent diminished survival in patients with CM treated by intralumbar drug administration compared with intraventricular treatment. Treatment by intraventricular intra-CSF chemotherapy administration results in improved drug dose and distribution in the CSF and is more convenient, especially for treatment that is often 2 or more times per week ( Table 2 ). Surgical implantation of a device and the risk of complications, however, especially iatrogenic bacterial meningitis, need to be balanced against the benefits of intraventricular intra-CSF drug administration.
Drug | Induction Regimens | Consolidation Regimen | Maintenance Regimen | |||
---|---|---|---|---|---|---|
Bolus Regimen | Concentration × Time Regimen | Bolus Regimen | Concentration × Time Regimen | Bolus Regimen | Concentration × Time Regimen | |
α-Interferon | 1 × 10 6 U 2 times weekly (total 4 wk) | 1 × 10 6 U 3 times weekly every other wk (total 4 wk) | 1 × 10 6 U 3 times weekly 1 wk per mo) | |||
Cytarabine | 25–100 mg 2 Times weekly (total 4 wk) | 25 mg/d for 3 d Weekly (total 4 wk) | 25–100 mg Once weekly (total 4 wk) | 25 mg/d for 3 d Every other wk (total 4 wk) | 25–100 mg Once a mo | 25 mg/d for 3 d Once a mo |
DepoCyt | 50 mg Every 2 wk (total 8 wk) | 50 mg Every 4 wk (total 24 wk) | ||||
Etoposide | 0.5 mg/d for 5 d Every other wk (total 8 wk) | 0.5 mg/d for 5 d Every other wk (total 4 wk) | 0.5 mg/d for 5 d Once a mo | |||
Methotrexate | 10–15 mg Twice weekly (total 4 wk) | 2 mg/d for 5 d Every other wk (total 8 wk) | 10–15 mg Once weekly (total 4 wk) | 2 mg/d for 5 d Every other wk (total 4 wk) | 10–15 mg Once a mo | 2 mg/d for 5 d Once a mo |
Rituximab | 25 mg 2 Times weekly (total 4 wk) | 25 mg 2 Times weekly every other wk (total 4 wk) | 25 mg 2 Times weekly once a mo | |||
Thiotepa | 10 mg 2 Times weekly (total 4 wk) | 10 mg/d for 3 d Weekly (total 4 wk) | 10 mg Once weekly (total 4 wk) | 10 mg/d for 3 d Every other wk (total 4 wk) | 10 mg Once a mo | 10 mg/d for 3 d Once a mo |
Topotecan | 0.4 mg 2 Times weekly (total 4 wk) | 0.4 mg 2 times weekly every other wk (total 4 wk) | 0.4 mg 2 Times weekly once a mo | |||
Trastuzumab | 20–100 mg 1 Time weekly (total 4 wk) | 20–60 mg 1 Time every other wk (total 4 wk) | 20–60 mg 1 Time every 4 wk |
High-dose systemic chemotherapy, in particular methotrexate and cytarabine, may obviate intra-CSF chemotherapy. High-dose therapy achieves cytotoxic CSF levels, as, for example, reported in patients with lymphomatous meningitis or breast cancer–related CM. The majority of systemic chemotherapy and some targeted therapies (ie, imatinib, rituximab, and trastuzumab), however, do not achieve adequate CNS penetration and consequently do not treat the CSF (water) compartment, which does not imply that systemic chemotherapy has no role in the treatment of NM but that for the majority of patients with NM, systemic chemotherapy is an adjunct treatment useful to treat extraneural disease and bulky subarachnoid disease.
Intra-CSF chemotherapy in NM is based on limited studies with comparatively small numbers of patients ( Table 3 ). Consequently, the role of intra-CSF chemotherapy in the treatment of NM has never been established in a prospective randomized trial. Nonetheless, several statements can be made regarding intra-CSF chemotherapy. Among the 3 most common intra-CSF chemotherapy agents used (ie, methotrexate, cytarabine, and thiotepa), there does not seem to be an advantage of one agent versus another nor does there seem to be an advantage for combining agents as is commonly prescribed for lymphomatous and leukemic meningitis (see Table 3 ). Liposomal cytarabine, an intra-CSF chemotherapy with a long half-life (approximately 140 hours), has been shown in 2 small randomized trials to be superior to methotrexate or cytarabine, although potentially with increased neurotoxicity (see Table 3 ). Due to increased efficacy (cytologic response, time to neurologic disease progression, non-neurologic cause of death, and improved quality of life), an argument has been made for considering liposomal cytarabine as the agent of first choice in patients with NM unless an investigational trial is available. Small trials with alternative intra-CSF chemotherapies (ie, topotecan, etoposide, Interferon alfa, and trastuzumab) have been used in adults with NM, although the appropriate role for these agents is unclear. Suggestions, including treatment of melanoma-related CM with a-interferon, germ cell cancer and small cell lung cancer CM with etoposide, Her2/neu-positive breast cancer–related CM with trastuzumab, and non–small cell lung cancer–related CM with topotecan, seem reasonable but are not evidenced based. All intra-CSF chemotherapy is associated with side effects such as fatigue associated with whole-brain radiotherapy and the induction of a chemical meningitis with intra-CSF chemotherapy. Intra-CSF chemotherapy commonly causes transient (<5 days) aseptic chemical meningitis that may be mitigated by administration of concurrent oral steroids. Chemical meningitis often manifests as headache, nausea/vomiting, fever, photophobia, meningismus, CSF pleocytosis, and occasionally delirium. Likely, the majority of patients manifest laboratory evidence of intra-CSF chemotherapy–related chemical meningitis; however, only a minority are symptomatic and in most are easily managed with oral medications. The major differential diagnosis is with respect to iatrogenic infectious meningitis wherein skin contaminants are introduced at the time of intra-CSF chemotherapy.
Study | Design | Response | Toxicity |
---|---|---|---|
Boogerd et al | N = 35 Breast cancer IT vs no IT a | IT vs no IT: improvement or stabilization: 59% vs 67% TTP: 23 vs 24 wk Median survival: 18.3 vs 30.3 wk | IT vs no IT: neurologic complications: 47% vs 6% |
Glantz et al | N = 28 Lymphoma DepoCyt vs Ara-C | DepoCyt vs Ara-C: TTP b : 78.5 vs 42 d OS b : 99.5 vs 63 d RR: 71% vs 15% | DepoCyt vs Ara-C: headache: 27% vs 2%; nausea: 9% vs 2%; fever: 8% vs 4%; pain: 5% vs 4%; confusion: 7% vs 0%; somnolence: 8% vs 4% |
Glantz et al | N = 61 Solid tumors DepoCyt vs MTX | DepoCyt vs MTX: RR b 26% vs 20% OS b 105 vs 78 d TTP 58 vs 30 d | DepoCyt vs MTX: sensory/motor: 4% vs 10%; altered mental status: 5% vs 2%; headache: 4% vs 2% |
Grossman et al | N = 59 Solid tumors and lymphoma (in 90%) IT MTX vs thiotepa | IT MTX vs thiotepa: neurologic improvements: none Median survival: 15.9 vs 14.1 wk | IT MTX vs thiotepa: Serious toxicities similar between groups. Mucositis and neurologic complications more common in MTX group. |
Hitchins et al | N = 44 Solid tumors and lymphomas IT MTX vs MTX + Ara-C | IT MTX vs MTX + Ara-C: RR b : 61% vs 45% Median survival b : 12 vs 7 wk | IT MTX vs MTX + Ara-C: N/V: 36% vs 50%; septicemia, neutropenia: 9% vs 15%; mucositis: 14% vs 10%; pancytopenia: 9% vs 10%. AEs related to reservoir: blocked Ommaya: 17% vs 0%; intracranial hemorrhage: 11% vs 0% |
Shapiro et al | Solid tumors (n = 103) DepoCyt vs MTX Lymphoma (n = 25) DepoCyt vs Ara-C | DepoCyt vs MTX/Ara-C: PFS b : 35 vs 43 d DepoCyt vs MTX: PFS: 35 vs 37.5 DepoCyt vs Ara-C: CR b : 33.3% vs 16.7% PFS: 34 vs 50 d | DepoCyt vs MTX/Ara-C: Drug-related AEs: 48% vs 60% Serious AEs: 86% vs 77% |
a Appropriate systemic chemotherapy and/or radiotherapy given in both arms.
At present there is an enormous unmet need for investigational trials of novel treatments for NM; however, there are several reasons why these are unlikely, at least in the immediate future. Neuro-oncology collaborative groups are gliomacentric with limited interest in studying NM. Because of the low incidence of the disease and limited survival after diagnosis, there is little business incentive for pharmaceutical companies to invest resources in NM, and a degree of therapeutic nihilism exists among oncologists in managing patients with NM that serves as a disincentive for development of clinical trials.
Symptomatic treatment of NM is directed at alleviating pain, which is most often headache and secondary to raised intracranial pressure that is best managed by either CSF shunting or treatment with whole-brain radiotherapy. Similarly, nausea and vomiting usually related to raised intracranial pressure are managed in a similar manner. As a general principle, there is little benefit from steroid administration in managing CM-related symptoms, with 2 exceptions. Patients with treatment-related chemical meningitis often benefit from steroid administration (described previously). In addition, patients with coexisting parenchymal brain metastases also derive benefit from steroids when peritumoral edema is extant. Antiepileptic drugs are rarely indicated because fewer than 10% of patients with NM manifest seizures and there is no benefit to prophylactic anticonvulsant use. A candid discussion regarding potential treatment side effects and expected treatment outcome is important because many patients may conclude that treatment is ineffective and, consequently, decline therapy. In patients proceeding with NM-directed therapy, preparing patients and families for a neurologic death and the expected sequential loss of neurologic function may help alleviate stress and provide an improved understanding of the dying process.
Epidural spinal cord compression
Introduction
The vertebral column is the most frequent site of bony metastases. Typically, the vertebral body is affected first, with posterior elements (pedicles and lamina) involved only one-seventh as often as anterior elements. Several factors probably contribute to the high incidence of metastatic deposition and growth in vertebrae. These include the valveless epidural venous plexus of Batson with bidirectional flow and direct communication with thoracic and pelvic venous system. In addition, the vertebrae contain vascular marrow (red marrow) throughout life unlike bones of the peripheral skeleton, which contain avascular marrow (yellow marrow). It is suggested the microenviornment of red marrow is more conducive to establishment of metastases due in part to increased vascularity. Cancer–bone interactions in part involve the osteoprotegerin (OPG) and receptor activator of nuclear factor κB (RANK) signaling pathway. OPG negatively regulates bone resorption by inhibiting osteoclasts vis-à-vis functioning as a false decoy receptor for RANK ligand (RANKL). Consequently, OPG is osteoprotective. RANK is expressed on osteoclasts and, on binding to RANKL, stimulates osteoclastogenesis and bone resorption. RANKL is the key stimulator of bone resorption and stimulates osteoclast formation and activation. The balance between OPG and RANKL seems to regulate bone resorption. RANKL is overexpressed in bone metastases whereas OPG serum levels are decreased in patients with bone metastases.
In an autopsy study of metastatic cancer, 70% of patients had vertebral body metastases, of which one-half had symptomatic pain requiring treatment. It is estimated that 5% of patients with cancer develop ESCC based on autopsy studies and approximately 80,000 new cases of ESCC occur annually in the United States. The most common sources of ESCC are breast cancer (20%), lung cancer (13%), lymphoma (11%), and prostate cancer (9%). Fifteen percent of all ESCC is localized to the cervical spine, 68% occurs in the thoracic spine, and 16% in the lumbar spine. The over-representation of thoracic involvement reflects the large size of the thoracic spine as well as the comparatively small diameter of the thoracic spinal canal.
Pathologically 3 stages of ESCC are seen. In the earliest stage, axonal swelling and edema of the cord white matter are observed with preserved spinal cord blood flow (SCBF). In the midstage, mechanical compression of the spinal cord is added to the increasing white matter edema, and early disturbances of SCBF are seen. In the late stage, SCBF decreases to critical levels, producing irreversible ischemic injury. In this stage, white matter hemorrhage and necrosis also are seen. The neurologic deficit of ESCC may be produced by direct mechanical compression of the spinal cord, cauda equina, or nerve root by tumor itself; by interruption of the vascular supply to the spinal cord by tumor; or by direct vertebral compression or collapse due to pathologic fracture (so-called spinal instability).
Several modes of spread to the epidural space are evident. Most often there is direct extension from an involved vertebra, as in carcinoma of the breast, lung, or prostate and plasma cell dyscrasias. Lymphoma more commonly grows through the intervertebral foramina into the epidural space from adjacent prevertebral lymph nodes. Hematogenous spread from Batson plexus or spinal radicular arteries rarely occurs.
Diagnosis
There is a striking similarity in the clinical presentation of patients with ESCC regardless of the site of origin of the primary tumor. Pain is almost always the earliest and most significant symptom (95% of all patients) ( Box 1 ). The pain is gradual in onset, is progressive over weeks to months, and may be focal, radicular, or referred. It is often most marked at night and aggravated by movement. Focal pain is localized, aching and continuous. Radicular pain is often intermittent, shooting in quality, and unilateral or bilateral. Referred pain occurs in a distal site without a radiating component. The pain may be exacerbated by movement, recumbency, neck flexion, straight leg raising, or a Valsalva maneuver. Usually a pain syndrome precedes neurologic disturbance but occasional patients present with sudden neurologic deficits. Because invasion of the spinal canal begins in the vertebra, motor functions of the anterior spine are compromised first with sensory disturbances afterwards. The sensory level on examination is not a reliable indicator of the level of ESCC because it is often documented several segments below by MRI. Loss of autonomic function (ie, incontinence) is usually a late sign. Presentation of incontinence only without pain or other neurologic deficits is essentially never a sign of ESCC. Presentation of ESCC most often reveals weakness that may range from paraparesis to paraplegia.
