Fig. 6.1
Noradrenergic innervation in an oblique longitudinal section of porcine distal colon. (a) Colonic mucosa was triple-labelled for dopamine β-hydroxylase (DBH; red), tyrosine hydroxylase (TH; green), and the tight-junction protein zonula occludens-1 (ZO1; blue) to visualise epithelial cells. Fibres reactive to antibody against DBH and TH were present in the colonic villi and often terminated in close proximity to epithelial cells (small arrowhead). Only very fine fibres could be distinguished in the most apical portion of the villi (small arrow). Colocalisation of DBH and TH (yellow) was evident in submucosal ganglia (large arrowhead) and nerve bundles (large arrow) near crypts (c) at the base of the villi. (b–d) Imaging at high magnification demonstrated reactivity to antibodies against DBH (b) and TH (c) colocalised in varicose nerve fibres (d). Asterisks in a and d indicate the region of a (scale bar 100 µm) presented in b–d (scale bar 10 µm). The image is a projection of six optical sections collected at 1 µm intervals. Reprinted from Green et al. (2004)
Significant spillover of catecholamines from the systemic circulation into the intestines can occur during episodes of acute stress (Aneman et al. 1996; Eisenhofer et al. 1997) and enhanced release of catecholamines by the ENS under stress has been demonstrated experimentally. Intestinal expression of tyrosine hydroxylase is upregulated in response to surgical perforation of the bowel and gut-derived sepsis in rats (Zhou et al. 2004) and catabolic stress induced by partial hepatectomy in mice has been reported to result in elevated levels of NE in faecal pellets compared to those from mice subject to sham-laparotomy (Alverdy et al. 2000). Evidence that such events may be significant in the progression of enteric disease was provided by the finding that NE and DA are able to induce the growth of commensal E. coli in vitro (Freestone et al. 2002). It was subsequently shown that NE stimulates the growth of Gram-negative enteric pathogens of various genera, including Salmonella (Freestone et al. 1999, 2007a, b; Williams et al. 2006), Shigella (O’Donnell et al. 2006), Yersinia (Freestone et al. 1999, 2007a, b; Lyte and Ernst 1992), Vibrio (Nakano et al. 2007b), Campylobacter (Cogan et al. 2007) as well as pathotypes of E. coli such as EHEC and ETEC (Lyte et al. 1997a). Norepinephrine can also resuscitate E. coli and Salmonella from a viable but nonculturable state (Reissbrodt et al. 2002), indicating that it may aid the outgrowth of physiologically-stressed bacteria that may enter mammalian hosts from the environment.
Systemic translocation of gut-derived bacteria and sepsis are well-known complications of surgery (reviewed in Nieuwenhuijzen and Goris 1999), and it is believed that outgrowth of enteric bacteria in response to the release of stress-related catecholamines may be significant in this process. Increased translocation of gut-derived bacteria to inguinal and mesenteric lymph nodes and the liver has also been observed during social conflict and restraint in C57BL/6 mice (Bailey et al. 2006). In addition, mice subject to partial hepatectomy are more susceptible to gut-derived sepsis caused by the opportunistic pathogen Pseudomonas aeruginosa (Laughlin et al. 2000) and exhibit elevated levels of NE in faeces (Alverdy et al. 2000). This was correlated with increased expression of the Ps. aeruginosa PA-I lectin in vivo, which is vital for pathogenesis (Alverdy et al. 2000; Laughlin et al. 2000). Expression of PA-I lectin is sensitive to NE in vitro (Alverdy et al. 2000) suggesting a molecular link between NE release under stress and the activity of bacteria in the intestinal lumen. Soluble factors in filtrates of faeces from mice subject to partial hepatectomy are able to activate PA-I lectin expression in vitro (Wu et al. 2003), though the role of NE in such stimulation has yet to be formally proven. The endogenous opioid dynorphin, which is released from the intestinal mucosa in mice subject to ischemia/reperfusion injury, also enhanced Ps. aeruginosa virulence in this model and virulence gene expression in vitro and in vivo (Zaborina et al. 2007). A detailed review of the ability of Ps. aeruginosa to respond to cues from the host may be found in Chap. 9. The following sections will focus on the impact and mode of action of stress-related catecholamines on the interaction of E. coli and Salmonella with intestinal mucosa.
6.2 Impact of Catecholamines on the Interaction of E. coli with Intestinal Mucosa
As indicated earlier, increased faecal excretion of E. coli has been noted in pigs subject to social stress (Jones et al. 2001) or physical handling (Dowd et al. 2007). Evidence that such events may be correlated with the release of stress-related catecholamines is afforded by the observation that the selective neurotoxin 6-hydroxydopamine rapidly and dramatically increases the number of E. coli in the cecum of mice (Lyte and Bailey 1997). 6-hydroxydopamine destroys noradrenergic nerve terminals without transit across the blood–brain barrier and elicits an immediate systemic release of NE. Within 14 days, during which time noradrenergic nerves regenerate, coliform counts returned to normal (Lyte and Bailey 1997). The effect could be inhibited by prior administration of desipramine hydrochloride, which specifically inhibits catecholamine uptake into noradrenergic nerve terminals, implying that damage to such neurons is required for bacterial outgrowth (Lyte and Bailey 1997). In murine models, stress induced by partial hepatectomy or short-term starvation also caused a profound increase in the number of E. coli adhering to the cecal mucosa compared to control mice (Hendrickson et al. 1999).
In addition to evidence that release of endogenous catecholamines may alter the activities of luminal E. coli, direct instillation of NE into the gut lumen has been reported to enhance adherence of E. coli O157:H7 to the mucosal surface in a bovine ligated ileal loop model (Vlisidou et al. 2004). E. coli O157:H7 can rarely be found in association with the mucosa 12 h post-inoculation of bovine ileal loops (Fig. 6.2a), however, if combined with 5 mM NE immediately prior to inoculation, dense microcolonies of adherent bacteria could be observed (Fig. 6.2b). Adherent bacteria were found to have formed “attaching and effacing” (AE) lesions that are known to be vital for bacterial persistence in the bovine intestines (Fig. 6.2c; Dziva et al. 2004; van Diemen et al. 2005). NE also significantly increased fluid accumulation and the recruitment of 111In oxinate-labelled neutrophils in response to E. coli O157:H7 compared to control loops filled with bacteria and diluent, albeit only when NE used at 5 mM and not 50 μM (Vlisidou et al. 2004). NE alone did not induce enteritis in this model, indicating that the effect was not the result of outgrowth of resident enteric bacteria or damage to intestinal mucosa.
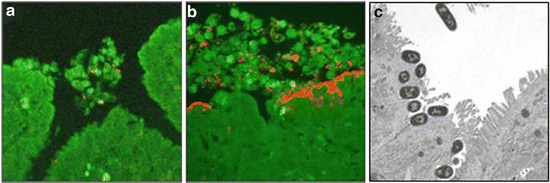
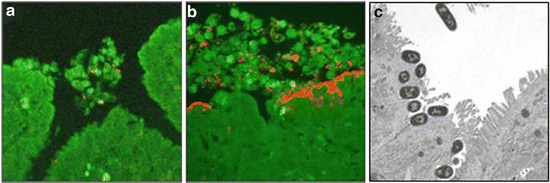
Fig. 6.2
Confocal laser scanning micrographs of bovine mid-ileal mucosa from ligated loops inoculated with E. coli O157:H7 strain 85–170 nalR in the presence of diluent (a), or 5 mM NE (b). F-actin was stained with fluorescein isothiocyanate-conjugated phalloidin (green), and bacteria detected with rabbit anti-O157 typing serum and anti-rabbit Ig-Alexa568 (red). Dense microcolonies of intimately attached bacteria were seen only in the presence of NE. Magnification ×630. Increased recruitment of neutrophils in the presence of NE was confirmed by analysis of γ-emissions associated with 111In-labelled neutrophils in the same tissues as used for microscopy (Vlisidou et al. 2004). (c) Shows a transmission electron micrograph of AE lesions induced by E. coli O157:H7 in the presence of 5 mM NE. Scale bar = 5 μm. Reprinted from Vlisidou et al. (2004)
The relevance of observations in the ligated loop model, where millimolar concentrations of NE were used, to the activities of E. coli in a stressed host is unclear. Short-term starvation prior to surgery and manipulation of the intestines to construct ligated segments does not stimulate extensive adherence of E. coli O157:H7 per se (Fig. 6.2a), and one may therefore question the relevance of the observations. However, the concentration of NE in the intestinal tract of normal and surgically manipulated calves is unknown. Quantification of tissue-associated and free NE in the intestines is difficult for several reasons (Grassi and Esler 1999; Hjemdahl 1993); (1) microdialysis probes used to sample NE would not be suitable for use in the gut owing to blockage of the dialysis membrane and the fact that NE levels in the dialysate may not reach equilibrium with the surroundings over time, (2) high-pressure liquid chromatography is required to quantify NE in gut contents and recovery through purification steps cannot be estimated, (3) breakdown products of NE can be detected in the intestines, and it is not feasible to calculate the loss of NE through the activity of host and/or bacterial enzymes or other processes. Despite these limitations, the model offers the advantage that at least ten strains or treatments can be evaluated in triplicate in the same animal relative to internal positive and negative controls, thereby minimising the impact of inter-animal variation.
Analysis of muscle-stripped intestinal explants clamped between half-tubes in Ussing chambers provides a further tractable model to probe the role of bacterial and host factors in colonization of the mucosal surface (reviewed in Chap. 5). In this system, NE promotes adherence of E. coli O157:H7 to murine cecal mucosa (Chen et al. 2003) and porcine colonic mucosa (Green et al. 2004). NE also promoted uptake of E. coli O157:H7, but not a rodent commensal E. coli strain, into porcine jejunal Peyer’s Patch tissue. EHEC are not widely considered to be invasive pathogens, and uptake by such tissue may reflect the antigen-sampling activity of M cells in follicle-associated epithelium (FAE). It is therefore noteworthy that FAE derived from rats subjected to chronic psychological (water avoidance) stress was found to take up higher levels of fixed fluorophore-conjugated E. coli K-12 as compared to epithelium from control rats (Velin et al. 2004). As inactivated bacteria were used and explants were not treated with exogenous neurochemicals, the data imply that stimulation of host tissues under stress may modulate subsequent interactions with enteric bacteria. Increased bacterial uptake was not observed in villus epithelium from stressed rats, indicating that the effect may be specific to FAE.
It is important to note that NE-stimulated adherence of E. coli O157:H7 to the luminal aspect of explants clamped in Ussing chambers occurred following the application of NE to the contraluminal aspect. Though it is not possible to preclude the possibility that some NE diffused to the luminal side, the amount of diffused NE and time of exposure is considered unlikely to have been adequate to promote a substantial increase in the number of bacteria available for adherence. Measurements of short circuit current and tissue electrical conductance indicated that the mucosa was viable and intact. In addition, pre-treatment of murine cecal explants with either the non-selective α-adrenergic receptor antagonist phentolamine or the β-adrenergic receptor antagonist propranolol prevented the action of NE, indicating that NE-promoted bacterial adherence at least partially reflects alterations in the host tissue (Chen et al. 2003). A similar effect of phentolamine on adherence and entry of E. coli O157:H7 into porcine jejunal Peyer’s patch mucosa has been reported (Green et al. 2003), however in this system, propanolol did not inhibit the effect of NE (Green et al. 2004; Chen et al. 2006). Evidence exists that the ability of NE to stimulate adherence of EHEC to porcine intestinal explants may be more sensitive to the α 2-adrenergic receptor antagonist yohimbine than the α 1-adrenergic antagonist prazosin (Green et al. 2004; Chen et al. 2006). The importance of release of NE from endogenous stores has also been suggested by the use of sympathomimetic drugs. For example, the NE reuptake inhibitor cocaine and α 2-adrenergic receptor agonist UK-14,304 both stimulate adherence of E. coli O157:H7 to porcine colon explants (Chen et al. 2006), as does a combination of the NE reuptake blocker desipramine and pargyline, an inhibitor of monoamine oxidase that influences catecholamine metabolism (Green et al. 2004).
Though most studies with explants have focussed on NE, it has also been shown that DA increases adherence of E. coli O157:H7 to murine cecal mucosa when applied to the contraluminal aspect in a manner sensitive to the DA antagonist haloperidol (Chen et al. 2003). The stress-related peptide adrenocorticotropic hormone (ACTH) also augments adherence of E. coli O157:H7, but not a pig-adapted non-pathogenic E. coli, to porcine colonic mucosa (Schreiber and Brown 2005). Tyramine, which is structurally related to dopamine and abundant in certain dairy products owing to the tyrosine decarboxylase activity of Enterococcus faecalis starter cultures, can promote adherence of E. coli O157:H7 to murine cecal explants (Lyte 2004). In addition, extracts of banana that are rich in NE and serotonin (Waalkes et al. 1958) augment growth of Gram-negative bacteria (Lyte 1997), as do a number of non-catecholamine dietary catechols (Freestone et al. 2007c; reviewed in Chap. 4). Further studies are required to determine if neurochemicals consumed in the diet are able to modulate the outcome of enteric bacterial infections.
6.2.1 Possible Mechanisms of Action of Norepinephrine During E. coli Infection
Though evidence exists that catecholamines partly exert their effects by acting on host tissues (above), it is clear that during culture in vitro they promote the growth of Gram-negative bacteria of several genera and expression of their virulence factors. E. coli O157:H7, Salmonella enterica and Yersinia enterocolitica vary markedly in their ability to grow in response to catecholamines (Freestone et al. 2007b). The response of Y. enterocolitica was limited to NE and DA, and these proved to be more potent inducers of growth than epinephrine in E. coli and Salmonella. The impact of catecholamines on bacterial growth is strictly dependent on inoculum density and media composition, being most prominent in a minimal salts medium supplemented with 30% (v/v) adult bovine serum (serum-SAPI) from inocula of 102–103 colony-forming units (CFU; reviewed in Chap. 3). From low inocula such media are bacteriostatic in the absence of catecholamines and are proposed to mimic the nutrient poor and iron-limited conditions encountered in vivo. The effect of catecholamines on growth is ameliorated during culture in rich media or at high inoculum densities. Growth stimulation is unlikely to be due to provision of a metabolite, as supplementation with the NE derivative normetanephrine, which contains one more methyl group than NE, failed to produce the effect (Lyte et al. 1996a, 1997a, b). Remarkably, α- (but not β-) adrenergic receptor antagonists are able to block NE- and epinephrine-induced growth and dopaminergic receptor antagonists inhibit the growth response to DA in the absence of effects on cell viability (Freestone et al. 2007a). These data imply that Gram-negative bacterial pathogens may possess elements that specifically interact with catecholamines and/or that antagonists can interfere with the ability of catecholamines to liberate factors required for bacterial growth.
Two major hypotheses have been put forward to explain the ability of NE to promote growth and virulence gene expression, and these are not mutually exclusive. The first posits that NE and related catecholamines facilitate the supply of iron to Gram-negative bacteria under iron-limiting conditions. As many virulence genes in Gram-negative bacteria are growth-phase regulated, this may explain downstream effects. The second hypothesis posits that virulence gene expression may result from microbial detection of catecholamines via specific receptors that initiate a signal transduction cascade on receipt of the signal leading to altered gene expression (Fig. 6.3). These hypotheses are considered in more detail subsequently.
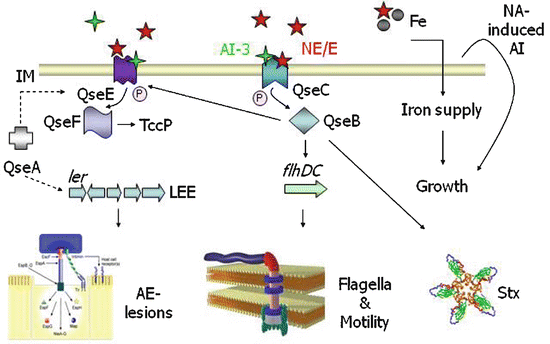
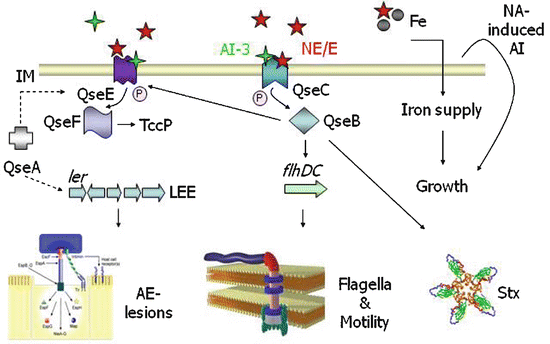
Fig. 6.3
Catecholamine sensing and signal transduction in E. coli O157:H7. QseC is an adrenergic sensor kinase that autophosphorylates on detection of epinephrine/NE/AI-3 and transfers the phosphate moiety to its cognate response regulator QseB, thereby activating transcription of the flagellar regulon (Clarke et al. 2006). The signalling cascade downstream of QseC in E. coli O157:H7 is known in some detail and is discussed elsewhere (Hughes et al. 2009). Transcription of genes encoding a second two-component system (QseEF) is sensitive to epinephrine and QseC (Reading et al. 2007). QseEF influences AE-lesion formation via activation of genes encoding a Type III secretion system and the Tir-cytoskeleton coupling protein (Reading et al. 2007), and recent evidence indicates that it directly senses catecholamines (Reading et al. 2009). Catecholamines also mediate iron supply by an ill-defined mechanism and promote bacterial replication in serum-rich iron-limited media from low inoculum densities by a mechanism that may involve induction of a heat-stable autoinducer (AI). NE stimulates production of Shiga toxins in E. coli O157:H7 however the signal transduction cascade leading to this event is unclear at the time of writing. Adapted from Reading et al. (2007)
The ability to liberate ferric iron from host storage proteins such as lactoferrin (Lf) and transferrin (Tf) is a key requirement in bacterial pathogenesis. Many Gram-negative bacteria secrete low molecular weight catecholate or hydroxamate siderophores to acquire iron, which are then imported via specific receptors. Significantly, NE, epinephrine and DA appear to facilitate iron supply from Lf and Tf, likely via the ability of the catechol moiety to complex ferric ion thereby lowering its affinity with Lf and Tf and releasing iron for siderophores to capture (Freestone et al. 2000, 2002, 2003). Recent data indicate that the formation of NE complexes with Lf and Tf leads to reduction of Fe(III) to Fe(II), for which Lf/Tf have a lower affinity (Sandrini et al. 2010). Siderophore synthesis and transport are required for NE-stimulated growth of E. coli, as strains with mutations affecting enterobactin synthesis (entA) or ferric-enterobactin transport (fepA or tonB) do not respond to NE in iron-limited serum-rich medium from a low inoculum (Burton et al. 2002; Freestone et al. 2003). This implies that NE does not act as a siderophore per se, although tritiated NE is taken into E. coli cells (Freestone et al. 2000; Kinney et al. 2000) and the role played by NE-ferric ion complexes in growth induction and the mechanism of NE import remain unclear. NE has also been found to induce the production of the ferric-enterobactin receptor (FepA), indicating that it may facilitate iron acquisition by multiple mechanisms (Burton et al. 2002).
Iron supply may not be the only mechanism by which catecholamines induce bacterial growth. Supernatants of E. coli cultures collected after induction of growth by NE in iron-limited serum-rich medium from low inocula contain a heat-stable autoinducer of growth that stimulates replication of naïve bacteria to a comparable extent as catecholamines (Lyte et al. 1996b; Freestone et al. 1999). The autoinducer is produced rapidly after exposure to NE and is able to activate growth of Gram-negative bacteria of several genera (Lyte et al. 1996b; Freestone et al. 1999). It has also been implicated in the ability of NE to resuscitate viable but non-culturable E. coli and Salmonella and appears to act in manner independent of iron-supply from Lf and Tf (Reissbrodt et al. 2002).
The ability of catecholamines to promote interactions between pathogenic E. coli and intestinal mucosa may reflect not only bacterial outgrowth, but also the induction of virulence factors. In ETEC O9:K30:H-, NE promotes expression of the K99 pilus adhesin (Lyte et al. 1997a, b). K99 pili are important in the pathogenesis of ETEC-induced diarrhoea in pigs, and such regulation may partially explain the effect of stress on the outcome of ETEC infection in pigs (Jones et al. 2001). Increased expression of Type I pili has also been described in commensal E. coli following stress induced by partial hepatectomy or short-term starvation in mice (Hendrickson et al. 1999). Such fimbriae are vital in the pathogenesis of ascending urinary tract infections by E. coli and were proposed to mediate important interactions with intestinal epithelia following catabolic stress (Hendrickson et al. 1999). However, this does not offer an explanation for the increased adherence of E. coli O157:H7 to bovine intestinal mucosa in the presence of NE (Vlisidou et al. 2004; Fig. 6.2b), as E. coli O157:H7 fail to elaborate functional Type I fimbriae owing to mutations in the fimA promoter (Roe et al. 2001) and FimH adhesin (Bouckaert et al. 2006). Indeed, expression of Type I pili is negatively selected during colonization of the bovine intestines by EHEC O26:H- (van Diemen et al. 2005).
Norepinephrine has also been reported to stimulate the production of Shiga toxins by E. coli O157:H7 during growth from a low inoculum in serum-containing medium (Lyte et al. 1996a, 1997a). Toxin production could also be stimulated by the catecholamine-induced autoinducer of growth, indicating that NE may partially act via this secreted intermediary (Voigt et al. 2006). Enhanced production of Shiga toxins is significant in the context of EHEC pathogenesis in humans, as the toxins may cause acute renal and neurological sequelae via damage to microvascular endothelial cells. Shiga toxins have also been reported to influence persistence of E. coli O157:H7 in the intestines of mice (Robinson et al. 2006) and rabbits (Ritchie et al. 2003), and to deplete a subset of intraepithelial lymphocytes in the bovine intestines (Menge et al. 2004). However, it is unlikely that this would explain the ability of NE to augment adherence and E. coli O157:H7-induced enteritis in bovine ligated ileal loops as a non-toxigenic strain was used and Shiga toxin 1 does not play a significant role in EHEC-induced enteritis in this model (Stevens et al. 2002).
A more plausible explanation for the ability of NE to stimulate adherence of E. coli O157:H7 to mucosa surfaces was afforded by the finding that it increases the production of several factors required for the formation of AE lesions (Sperandio et al. 2003; reviewed in Chap. 12). AE lesions are characterised by intimate bacterial attachment to enterocytes and localised destruction of microvilli (Fig. 6.2c), and their formation relies on a Type III protein secretion system (T3SS) encoded by the locus of enterocyte effacement (LEE). This apparatus injects a set of bacterial proteins into enterocytes, one of which (the translocated intimin receptor, Tir) becomes localised in the apical leaflet of the host cell plasma membrane where it serves as a receptor for the bacterial outer membrane protein intimin. Both intimin, Tir, and the T3SS play pivotal roles in colonization of the bovine intestines by E. coli O157:H7 (Dziva et al. 2004; van Diemen et al. 2005; Vlisidou et al. 2006). A mutant lacking both intimin and Tir did not adhere to the surface of bovine ileal loops in response to NE 12 h after loop inoculation (Vlisidou et al. 2004). However, studies in Ussing chambers have indicated that intimin and the T3SS component EspA are not required for NE-stimulated early non-intimate adherence to porcine intestinal explants (Chen et al. 2006). Production of LEE-encoded proteins is regulated by the growth phase, however induction of the expression and secretion of Type III secreted proteins, as well as induction of transcription of LEE operons, was reported to occur in the absence of effects on growth (Walters and Sperandio 2006). Epinephrine and NE also promote motility of E. coli O157:H7 (Sperandio et al. 2003), by a mechanism that appears to involve induction of the flagella regulon via the master regulators FlhDC (Clarke et al. 2006). In a bovine model, an E. coli O157:H7 flhC mutant was impaired in its ability to persist in the intestines, but a mutant lacking the flagellin subunit FliC was not (Dobbin et al. 2006), indicating that if NE acted via this circuit to promote adherence in the bovine intestines, it may have required flhDC-regulated genes other than flagella genes for the effect.
A key contribution to our understanding of host-microbe communication was the identification of an adrenergic receptor in E. coli O157:H7 (Clarke et al. 2006; reviewed in Hughes and Sperandio 2008). This arose from the finding that the qseBC genes, which regulate flagella-mediated motility (Sperandio et al. 2002), are required for the ability of epinephrine to stimulate motility (Sperandio et al. 2003). QseBC exhibit homology to two-component systems, which typically comprise a sensor kinase (SK) that autophosphorylates at a conserved histidine on receipt of a specific signal and a response regulator (RR) to which the phosphate moiety is transferred from the cognate SK. Phosphorylation of the RR alters its activity, leading to altered expression of genes under its direct or indirect control. It was subsequently proven that QseC binds tritiated NE and that it autophosphorylates in response to epinephrine and NE when reconstituted in lipid micelles (Clarke et al. 2006). Transfer of the phosphate moiety to QseB after epinephrine stimulation of QseC could be detected, and this is believed to modulate transcription of genes under the control of QseB, which include flhDC (Clarke et al. 2006). The signalling cascade downstream of QseC is now known is some detail (Hughes et al. 2009). Catecholamine binding to QseC and autophosphorylation could be blocked by the α-adrenergic receptor antagonist phentolamine, but not by the β-adrenergic antagonist propanolol (Clarke et al. 2006), implying that QseC may structurally mimic eukaryotic adrenergic receptors. QseC also senses a bacterial autoinducer (AI-3) that provides a measure of population density (Sperandio et al. 2003). The interplay between such ‘quorum sensing’ systems and detection of host-derived catecholamines is reviewed in detail in Chap. 12.
The precise role of the QseBC system in activation of LEE genes remains to be determined. Interestingly, NE-stimulated adherence, production of LEE-encoded proteins and AE-lesion formation can be inhibited by the β-adrenergic receptor antagonist propanolol (Chen et al. 2003; Sperandio et al. 2003), even though this treatment does not impair QseC autophosphorylation in the presence of NE (Clarke et al. 2006). This finding, taken together with the fact that some enteric pathogens respond to NE but lack a QseC homologue (e.g. Campylobacter jejuni and Yersinia enterocolitica), may reflect the presence of other bacterial catecholamine receptors or alternative modes of action. In the case of E. coli O157:H7, it has been reported that a second two-component sensory system (QseEF), that is regulated by QseBC and required for AE-lesion formation (Reading et al. 2007), acts as a secondary receptor for epinephrine (Reading et al. 2009). It has been established that QseC is required for the induction of enteritis by rabbit enteropathogenic E. coli in infant rabbits (Clarke et al. 2006). However, mutations in two-component systems often have pleiotropic effects, and it remains to be shown that QseC is required for NE-induced phenotypes of E. coli O157:H7 in vivo. A specific inhibitor of QseC signalling (LED209) has recently been reported to interfere with actin nucleation by E. coli O157:H7 and the transcription of flhDC and stx2A; however, studies of its ability to control infection in infant rabbits were complicated by rapid adsorption of the inhibitor from the gut (Rasko et al. 2008).
In an attempt to define the global transcriptional response of enteric pathogens to catecholamines, microarray studies have been undertaken by several laboratories. Bansal et al. defined the transcriptome of E. coli O157:H7 during biofilm formation on glass wool during 7 h culture in Luria Bertani (LB)-glucose medium supplemented with 50 μM epinephrine or 50 μM NE. A total of 938 and 970 genes were differentially transcribed in response to epinephrine and NE, respectively, with 411 genes exhibiting the same pattern of transcription in response to both catecholamines (Bansal et al. 2007). The fact that epinephrine and NE exert different effects at the level of transcription is consistent with the observation that they do not stimulate the growth of E. coli O157:H7 to the same extent (Freestone et al. 2007a, b). Several genes involved in iron acquisition were upregulated in response to epinephrine and NE, however Shiga toxin and LEE genes were not found to be activated under the conditions used (Bansal et al. 2007), in contrast with observations at the protein level. Following growth in serum-SAPI medium in the presence of 50 mM NE from an inoculum of c. 106 CFU, Dowd detected differential regulation of 101 genes, including induction of genes involved in iron acquisition and the genes for intimin, T3SS components and Shiga toxins (Dowd 2007). Such changes mirror those seen at the protein level (Lyte et al. 1996a, 1997a; Voigt et al. 2006; Sperandio et al. 2003); however, other genes in the same operon as eae and espAB were not observed to be differentially regulated. The fepA gene was found to be repressed, in contrast with other studies (Burton et al. 2002), whereas induction of feoB and fhuD was also reported by Bansal et al. Culture of an E. coli O157:H7 luxS mutant (unable to synthesize autoinducer) in Dulbecco’s modified Eagle’s medium with 50 μM epinephrine has also been reported to induce transcription of LEE, stx2 and flagella genes (Kendall et al. 2007). A key consideration with studies of this nature is the effect of exogenous catecholamines on bacterial growth. If control and treated cultures are collected at different points in the growth cycle, it is difficult to interpret whether altered virulence gene expression is a direct consequence of catecholamine-mediated regulation (e.g. via QseBC and QseEF) or associated with the change of growth phase. A further consideration is that at high concentrations catecholamines may sequester iron leading to indirect activation of iron acquisition genes. Such aspects are reviewed in detail elsewhere (Freestone et al. 2008).
6.3 Impact of Catecholamines on the Interaction of Salmonella with Intestinal Mucosa
In addition to the finding that pigs subject to social stress or transport exhibit increased faecal excretion of S. enterica serovar Typhimurium (Isaacson et al. 1999; Callaway et al. 2006), several studies have addressed the impact of pre-treating Salmonella or the host with catecholamines on the outcome of infection. Culture of S. Typhimurium in serum-SAPI medium containing 2 mM NE increased colonisation of selected tissues following oral inoculation of piglets compared to bacteria grown in Luria Bertani medium in the absence of NE (Toscano et al. 2007). However, it is likely that the physiological status of bacteria grown in serum-rich minimal salts medium is different to that of bacteria cultured in rich media and, though comparable numbers of bacteria were administered, they may have differed in growth phase and gene expression. Indeed, studies in the author’s laboratory failed to reproduce the effect of precultivation in the presence of NE when S. Typhimurium strain 4/74 was grown in LB ± 5 mM NE prior to inoculation of 6-week-old Large White pigs (Stevens MP, unpublished observations).
In relation to the role of stress-related catecholamines during systemic salmonellosis, it has been reported that NE promotes Salmonella encephalopathy in calves challenged orally with clinical isolates of serovars Enteritidis, Montevideo and Saintpaul associated with neurological disease. Calves given 45 μg/kg NE daily via the intramuscular route developed neurological signs following inoculation with these isolates and had evidence of bacterial replication in the brain, whereas control animals given a placebo were only positive for bacteria by faecal culture (McCuddin et al. 2008). In the same study however, daily intramuscular administration of NE did not enhance faecal excretion of S. Typhimurium DT104 and even decreased excretion of S. Dublin (McCuddin et al. 2008), albeit that the relative ability of NE deposited in muscle to act on bacteria at enteric and systemic sites in calves at this dose is ill-defined. Intra-gastric administration of NE to mice the day before inoculation with S. Typhimurium increased cecal colonisation and translocation to the liver in a dose-dependent manner (Williams et al. 2006). Similarly, chicks given twice daily NE by crop instillation exhibited elevated levels of S. enterica serovar Enteritidis in the ceca and liver compared to controls (Methner et al. 2008).
Research in the author’s laboratory has indicated that S. Typhimurium-induced fluid accumulation and 111In-labelled neutrophil recruitment in bovine ligated ileal loops is increased by mixing the bacteria with NE immediately prior to inoculation (Pullinger et al. 2010). However, as with E. coli O157:H7, relatively high concentrations of NE (5 mM) were required to produce a statistically robust phenotype in this model. Using explants of porcine jejunal Peyer’s patch mucosa clamped in Ussing chambers, addition of NE to the contraluminal aspect increased uptake of S. Choleraesuis in a phentolamine-sensitive manner (Green et al. 2003). Whilst this implies that NE may facilitate S. Choleraesuis invasion by a mechanism dependent on host adrenergic receptors, NE did not significantly enhance internalisation of S. Typhimurium DT104 by porcine jejunal Peyer’s patch explants or ileal non-follicular mucosa in Ussing chambers (Schreiber et al. 2007). Indeed treatment of such explants with sympathomimetic drugs even decreased recoveries of S. Typhimurium DT104 (Brown and Price 2008). S. Typhimurium invades porcine intestinal mucosa more efficiently than S. Choleraesuis in Ussing chambers (Brown and Price 2008) and ileal loops (Paulin et al. 2007), and the effect of exogenous catecholamine on invasion may therefore be less pronounced if a finite level of uptake is possible under the assay conditions. Further studies are required to determine if there are strain- or serovar-specific mechanisms by which catecholamines may modulate the outcome of S. enterica infections.
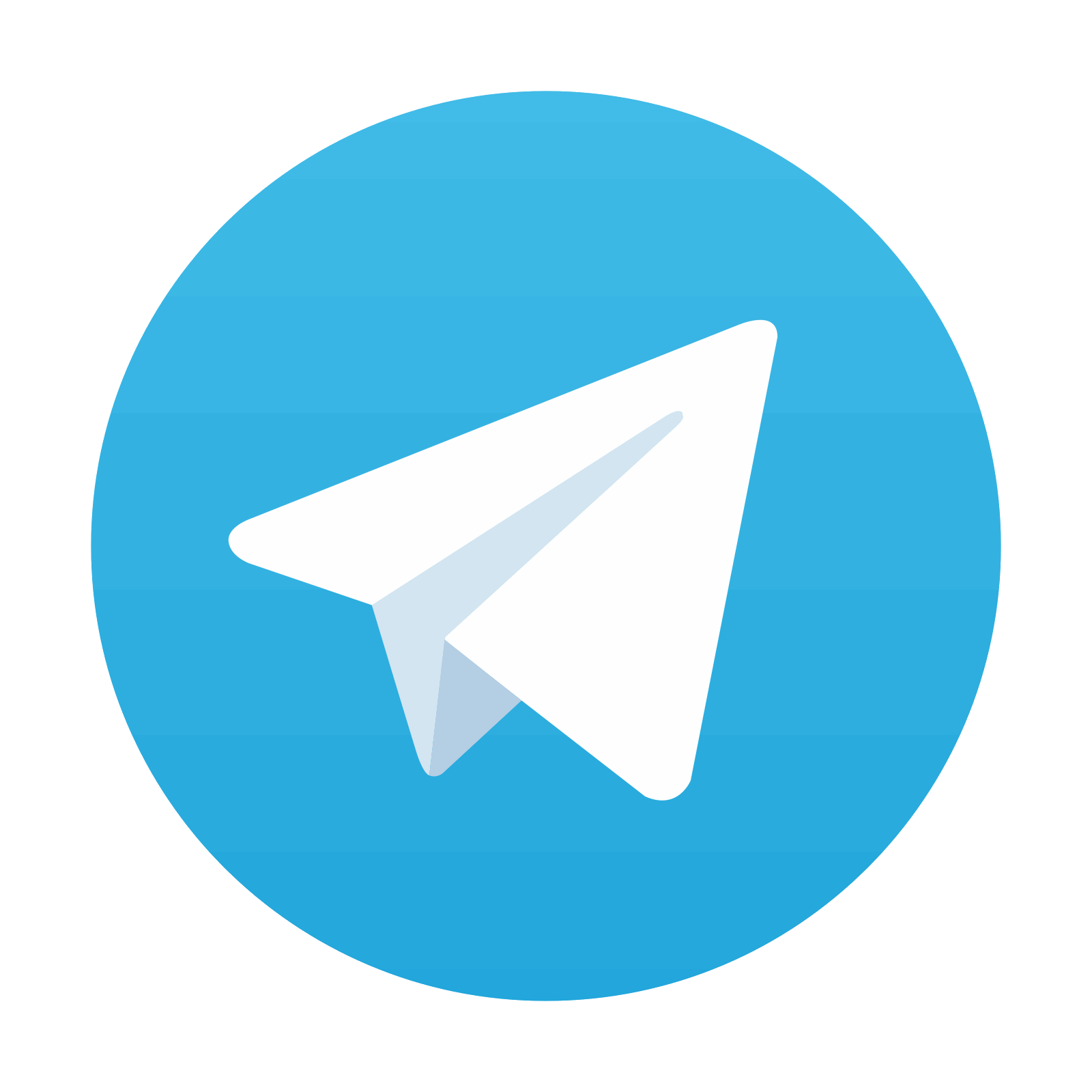
Stay updated, free articles. Join our Telegram channel

Full access? Get Clinical Tree
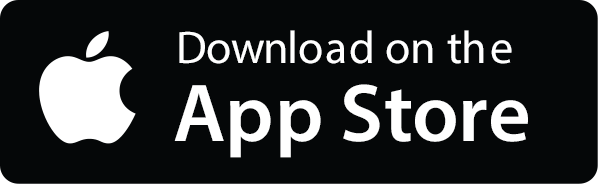
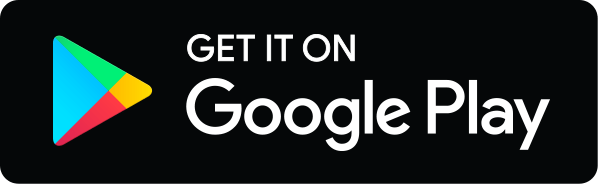