Abstract
The cumulative incidence of brain metastasis at 5 years is approximately 5% in patients with breast cancer but is much higher in postmortem autopsy series. Overall breast cancer is the second most common cause of brain metastasis. Leptomeningeal metastatic disease is rarer at less than 1%. Metastatic epidural spinal cord compression preceding death due to breast cancer has been reported at 5.52%. Prompt diagnosis and treatment before the development of paralysis or paresis is associated with a better prognosis. Patients who present with cord compression without paresis can be adequately treated with radiation therapy (RT) alone (with or without corticosteroids). Patients who present with paresis or paralysis are less likely to regain neurologic function and have a worse prognosis. These patients require urgent evaluation and may be best treated with immediate decompression through surgery followed by postoperative RT.
Keywords
central nervous system metastases, brain metastases, metastatic breast cancer
Incidence
In 2016, there was an estimated 246,660 new cases of breast cancer in the United States alone, and 40,450 women died of this disease. A subset of these patients succumbed to brain metastasis as their primary cause of death. Population-based studies support that the cumulative incidence of brain metastasis in 5 years is approximately 5.0% in patients with breast cancer in general. The true incidence of metastatic breast cancer (MBC) to the brain remains to be determined because postmortem autopsy series report higher prevalence. Each year in the United States an estimated 170,000 cancer patients are diagnosed with brain metastastis. Overall breast cancer is the second most common cause of brain metastasis, second only to lung cancer and accounting for approximately 20% of the cases of brain metastases. Breast cancer brain metastases are commonly a late occurrence, with a median time from diagnosis of breast cancer to brain metastasis identification of 2 to 3 years. The median age of diagnosis of metastatic disease to brain is approximately 45 years.
Leptomeningeal metastatic disease (LMD) is rarer than brain parenchyma disease. In one series of 4079 patients with breast cancer and 1809 patients with recurrent disease, LMD was present in 0.86% of patients with breast cancer and 1.9% of patients with recurrent disease. The median age of incidence ranges from 45 to 57.
The 5-year cumulative incidence of metastatic epidural spinal cord compression (ESCC) preceding death of breast cancer was reported at 5.52% among 35,197 cases of breast cancer analyzed in Ontario, which is in harmony with results from other series. At diagnosis its prevalence was estimated at 0.11%. The median age at diagnosis is of ESCC is 51 years.
Risk Factors
Results from a population-based studied conducted in the United States among 51,898 women supports that age less than 40 and African American women correlated with higher risk of central nervous system (CNS) involvement among patients with early-stage breast cancer. Evans and coworkers reported a case series in which 219 patients in which 43% of women diagnosed with MBC would eventually develop brain metastatsis.
Stage at the time of diagnosis is a determinant risk factor for CNS brain metastasis. This is a rare event in women undergoing breast conserving surgery for early-stage breast cancer with 5-year cumulative incidence as low as 1.7% reported in patients with clinical stage I–II tumors. Axillary node metastasis also seems to increase the risk of CNS relapse compared with axillary node–negative disease. Patients presenting with increased number of metastatic sites are at increased risk for occult CNS disease. In addition, in one series multivariate modeling supported a hazard ratio (HR) of 4.3 for CNS metastasis when patients had pulmonary involvement. These findings indicate that tumor burden is most likely a continuous variable, which correlates positively with the risk CNS involvement in either early- or late-stage disease.
Tumor biological variables are also predictive of increased risk of CNS disease in patients with breast cancer. HER2 overexpression, which is observed in up to 25% to 30% of patients diagnosed with breast cancer, confers poor prognosis in the localized and metastatic settings. The advent of targeted therapies against HER2 overexpressing breast cancer amounted to significant improvement in overall survival (OS) and disease-free survival. The median OS for patients presenting HER2-positive MBC and treated with the combination of trastuzumab, pertuzumab, and docetaxel is now estimated at 56.5 months 95% CI (49.3 to not reached) in the first-line setting. In light of improved management and prolonged survival, brain metastasis were found to be a common event in patients with presenting with metastatic HER2-positive breast cancer with a risk ranging from 35% to 50% over the course of their disease. For patients presenting with early-stage disease, HER2-positive breast cancer, CNS-only recurrence is a rare event regardless of trastuzumab adjuvant therapy with cumulative risk less than 3% reported in different studies. Nonetheless, it is important to highlight that late recurrences diagnosed several years from the diagnosis of breast cancer are also more prevalent in HER2-positive/HR-negative tumor compared with other subtypes.
Lack of estrogen receptor (ER) overexpression has also been correlated with increased risk of CNS recurrence in early breast cancer, whereas ER-positive tumors seem to have a lower risk of CNS involvement.
Furthermore, most recently next-generation sequencing has clustered breast tumors according to variation in genomic transcriptional patterns resulting in different prognostic subtypes. In one series of 1434 consecutive patients with stage I–II invasive breast cancer with a median follow-up of 85 months, Arvold and coworkers stratified patients according to immunohistochemistry approximate genomic subtypes; the 5-year cumulative risk of brain metastasis was 0.1% for luminal A, 3.3% luminal B, 3.2% for luminal HER2, and 7.4% for triple-negative breast cancer (TNBC) tumors.
Other pathologic features of the primary breast tumor associated with increased risk of CNS relapses include expression of cytokeratin 5/6 and 14, epidermal growth factor receptor (EGFR), p53, elevated S-phase fraction, DNA ploidy, tumor size, tumor grade, and invasive ductal histology.
LMD is more common among lobular carcinoma of the breast compared with ductal histology. Small series conducted in the pre-HER2 era suggest that LMD may be more prevalent the hormone receptor–negative tumor population. Finally, small case series suggest that ESCC is more common in patients with tumors overexpressing hormonal receptors.
Methods of Spread and Distribution
Among 400 women with breast metastatic to the CNS reported by Kim and colleagues, approximately 80% of patients presented with brain metastases confined to the brain parenchyma, 7.5% had LMD only, and 13% had both. Furthermore, more than 95% of women presented with CNS involvement as a late manifestation of disease. Intracerebral metastases at presentation are five times more common compared with solitary lesions. Brain recurrence as the first site of recurrence is a rare event accounting for up to 12% of cases with CNS metastasis. Patients presenting with locally advanced or inflammatory breast cancer seem to have higher risk of recurrence in the CNS as their first site of disease recurrence. Likewise, LMD is a late complication of breast cancer and usually associated with concomitant systemic disease. The median latency period from breast cancer diagnosis and LMD is 25 to 38 months. In one small series, LMD was the first manifestation of recurrent disease in 4 of 25 patients and another 1 of 44 patients.
Clinical Presentation
Brain Metastasis
Signs and symptoms from brain metastases are varied and are caused by the displacement or destruction of structures within the brain. Possible symptoms include headache, focal neurologic dysfunction, cognitive decline, encephalopathy, seizures, and strokes. A cross-sectional study published by Posner and colleagues evaluated 111 patients with either primary or metastatic tumors to the brain and their presenting symptoms. Headaches were present in up to 48% of patients and equal for primary and metastatic brain tumors. The typical headache was bifrontal but worse ipsilaterally to the metastatic lesion(s) and was the worst symptom in only 45% of patients. Unlike true tension-type headaches, brain tumor headaches were worse with bending over in 32%, and nausea or vomiting was present in 40% of patients. The “classic” early-morning brain tumor headache was uncommon. Nausea, vomiting, an abnormal neurologic examination, or a significant change in prior headache pattern suggests that the headache may be caused by a tumor. The high prevalence of headache in patients with metastatic or primary brain tumor has been observed in other studies. Encephalopathy alone is a rare presentation of metastatic brain tumors and is often secondary to systemic toxic metabolic encephalopathy. Up to 18% of patients present with seizures as the first manifestation of brain metastatic disease. Intracranial bleeding is a recognized complication of metastatic tumors to the brain and clinical presentation depends on location of the event. Although hemorrhage can be seen, anticoagulation is otherwise safe for medical indications in patients with brain metastases as long as patients have not had active bleeding of their metastases.
Leptomeningeal Metastasis
Most commonly LMD presents with headache and involvement of cranial nerves, often particularly to the cranial nerves that control extraocular muscles. In addition symptoms of LMD can be caused by the following pathophysiologic mechanisms: (1) mass effect resulting in cerebrospinal fluid (CSF) obstruction and hydrocephalus and/or increased intracranial pressure, (2) tumor involvement of the cranial nerves or spinal roots, (3) invasion of brain parenchyma via Virchow-Robin spaces, and/or (4) disruption of the blood-brain barrier (BBB). The clinical hallmark of LMD is a constellation of signs and symptoms caused by multifocal involvement of the neuraxis (i.e., cerebrum, cranial nerves, and spinal roots). Because LM is a disease of cancer cells in the subarachnoid space and CSF, there is frequent cranial nerve and spinal nerve involvement along with other classic meningitis signs. In a study of 102 patients with LMD, 33%, 39%, and 13% of patients had signs and symptoms involving one, two, or all three levels of the neuraxis, respectively.
Rarely do patients present with a single neurologic symptom, and often careful neurologic examination reveals other neurologic dysfunction such as ocular muscle paresis, facial weakness, diminished hearing, optic neuropathy, trigeminal neuropathy, hypoglossal neuropathy, blindness, and diminished gag reflex. Other findings include seizures, hemiparesis, cerebellar dysfunction (manifested as difficulty walking), nausea, and vomiting. Another common symptom from cranial nerve involvement is facial numbness, particularly involvement of the third division of the trigeminal nerve. This cranial nerve neuropathy results in numbness of the chin and is referred to as the numb chin syndrome. Spinal root invasion often causes symptoms of weakness, paresthesias, back and neck pain, radicular pain, and/or bowel and bladder dysfunction. Meningeal signs of nuchal rigidity and pain on straight leg raises are common. Symptoms of hydrocephalus and/or increased intracranial pressure, which include headache, nausea, vomiting, and dizziness, occur in 50% of patients.
Epidural Spinal Cord Compression
Epidural Spinal Cord Compression (ESSC) is a medical emergency and should be promptly recognized and treated. In one case series of 70 patients, the most common presenting symptoms with spinal cord compression were pain (94%), muscle weakness (96%), sensory loss (79%), and sphincter dysfunction (61%); at least one of these symptoms was present in 100% of the patients. In one series of 100 patients with known breast cancer presenting with signs/symptoms of radiculopathy or myelopathy, epidural metastasis were diagnosed in 54% of the cases, indicating the diagnostic workup should be initiated with no delay in this patient population.
The most common presentation for ESCC is complaint of severe lower back pain that progressively worsens. The pain is worse when lying supine; this occurs because of distension of the epidural venous plexus. The pain may also result from disruption of the periosteum, the cauda equina, the spinal cord, or paravertebral soft tissue. Over time, the pain may develop into a radicular quality. Abrupt worsening of back pain may be a sign of a compression fracture.
Up to 85% of patients have partial weakness or paresis at presentation. ESCC generally produces symmetric bilateral extremity weakness. A superimposed radiculopathy can occur with lateralized epidural lesions. Associated hyporeflexia is seen with ESCC at the level of the cauda equina, whereas hyperreflexia is seen with a lesion at or above the conus medullaris. If treatment is not initiated promptly, which is common, the weakness will progress to cause difficulty with ambulation and, eventually, paralysis. Most patients (60%–70%) have difficulty with ambulation at the time of diagnosis. Sensory deficits also occur in the majority of spinal cord compression patients on presentation. The most frequent symptom is ascending paresthesias. On physical examination, the sensory deficit corresponds closely to the dermatome level. “Saddle anesthesia” is a common sensory deficit in patients with cauda equina syndrome. Lhermitte sign (an electrical sensation down the spine elicited by neck flexion) is rarely seen with ESCC and more frequently seen in LMD.
Autonomic dysfunctions such as urinary hesitancy, retention, and incontinence are relatively uncommon. These symptoms usually occur as a late manifestation of myelopathy. Isolated painless ataxia secondary to spinocerebellar tract dysfunction caused by ESCC has also been reported.
Unfortunately, the diagnosis of ESCC is often delayed. A prospective study of 301 patients examining the delay in presentation, diagnosis, and treatment of spinal cord compression observed a median delay of 14 days (range, 0–840 days) between onset of symptoms and treatment.
Diagnostic Evaluation
Diagnosis of CNS metastases can often be made based on clinical history, presentation, and/or imaging findings. However, a biopsy and/or CSF sampling may be helpful.
Brain Metastases
The differential diagnosis for a brain lesion includes primary brain tumor, infectious abscess, progressive multifocal leukoencephalopathy, demyelination, cerebral infarction or bleeding, and radiation necrosis (if the patient has had prior radiation therapy [RT] to the brain).
Gadolinium-enhanced magnetic resonance imaging (MRI) is the best method for detecting and localizing brain metastases with increased sensitivity compared with contrast-enhanced computerized tomography (CT) scan. MRI also has increased sensitivity in detecting posterior fossa and cortical lesions. Sensitivity and specificity of 18 fluorodeoxyglucose positron emission tomography (FDG-PET) was tested against MRI as the gold standard in one series of 40 patients with suspected brain metastatis. Twelve patients with brain metastasis were identified on FDG-PET with a sensitivity of 75% and specificity of 83%. Small lesions were particularly difficult to diagnose on FDG-PET. In another cross-sectional study of 50 patients with suspected brain metastasis assessed the accuracy of combining FDG-PET fused with CT imaging. Seventy cerebral metastasis were diagnosed on MRI in 20 patients and FDG-PET/CT’s sensitivity of detection of all 70 lesions was only 20%. These results indicate that MRI should remain the preferred radiologic test for the evaluation of suspected brain metastasis.
Brain metastases are usually located at gray-white junctions and have well-circumscribed boundaries. The majority of patients with diagnosis of cancer present with multiple lesions, and a significant amount of edema may surround a metastasis that is disproportionate to the size of the tumor mass. On a CT scan, breast cancer metastases are usually isodense to the surrounding brain with enhancement after contrast infusion. Calcification within a metastasis is rarely seen. On MRI studies, the metastasis is usually hypointense to the brain on T1-weighted images and hyperintense to the brain on T2-weighted images. After contrast administration, most brain metastases strongly enhance on both CT and MRI. A solid homogeneous enhancement pattern is typical, but a ring-enhancing pattern may be seen. Cystic and heterogeneously enhancing breast cancer brain metastases are seen less frequently with few cases reported in the literature. Newer imaging modalities such as MRI spectroscopy and FDG-PET may prove to be helpful in the diagnoses of brain metastases. Currently, they are used to help differentiate between tumor recurrence and radiation necrosis.
A biopsy is warranted if the diagnosis of brain metastases is ever in doubt, especially if a patient presents with a single brain metastasis. In one prospective study of 54 patients with a history of primary cancer and a single mass lesion seen on both CT and MRI, 6 (11%) of those patients had nonmetastatic lesions, including two glioblastomas, one low-grade astrocytoma, two abscesses, and one nonspecific inflammatory reaction. Therefore, biopsy should be strongly considered before RT if complete surgical resection of a single lesion is not planned or if there is doubt about the diagnosis. Cerebral abscesses are especially problematic because they can mimic metastases clinically and radiographically, and treatment of an abscess with RT would be detrimental. Modern stereotactic cerebral biopsy is a highly effective technique to obtain a tissue diagnosis and can be performed with low morbidity. In one series of 500 cases with predominant target locations within the cerebral centrum-basal ganglia (284 cases) and diencephalic-mesencephalic regions (129 cases) in which stereotactic biopsy was formed for diagnostic and therapeutic objective the observed mortality was 0.2% and morbidity was 1%. Possible complications from the procedure include intracerebral hemorrhage and infection.
Leptomeningeal Metastases
The differential diagnosis for LMD includes infectious etiologies (i.e., bacterial meningitis, Lyme disease), autoimmune disorders (i.e., vasculitis, sarcoidosis), and artifact (i.e., post-RT changes). The diagnosis of LM is based on obtaining a detailed history and identifying neurologic signs on physical examination that suggest multifocal involvement of the neuraxis. Combining contrast-enhanced MRI scans with CSF cytology enhances the diagnostic accuracy of LM. CSF cytology has 100% specificity and a sensitivity of 75% for single analysis. The probability of disease detection with CSF cytology can be affected by not only by tumor dependent variables but also by the amount of CSF collected, site of CSF sampling, and processing time. Sensitivity of CSF analysis correlates with number of collections and has been reported to be 98% with four collections. MRI of symptomatic area (axial imaging of the brain or sagittal imaging of the spine) has been reported to be as sensitive and CSF cytology with sensitivity of 76% and specificity of 77%.
Radiologic evaluation of possible LMD should be performed through MRI brain imaging. CT scan has limited clinical utility in diagnosing LMD insofar as it has been reported to have a sensitivity as low as 40% compared with up to 100% for MRI in a small series of 41 patients with LMD.
Nearly all LMD enhances dramatically after contrast administration for an MRI scan ( Fig. 73.1 ). Typical MRI findings in the brain include thin, diffuse leptomeningeal enhancement, multiple nodular or plaque-like deposits adherent to the dura, and tumor masses with or without hydrocephalus. On MRI, the entirety of the spinal cord, as well as the cauda equina, exhibits linear enhancement. Nodular deposits may be seen on spinal nerve roots and on the cauda equina.
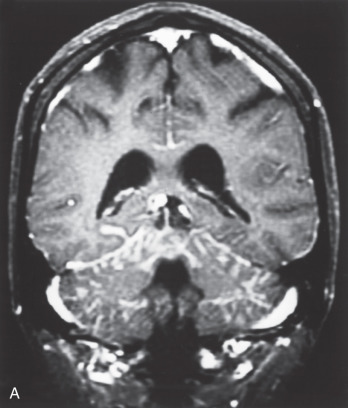
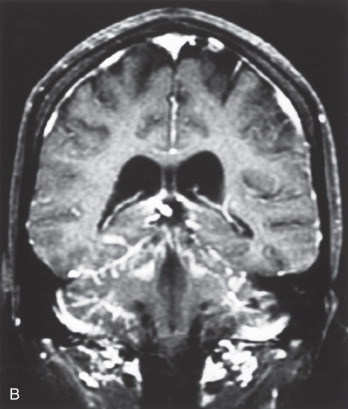
Before a lumbar puncture is performed, increased intracranial pressure should be ruled out via an ophthalmic examination for papilledema. The typical CSF findings for LM are elevated opening pressure, elevated protein content, decreased glucose concentration, elevated white blood cell count, and xanthochromia. Elevation of tumor markers (i.e., CEA, CA-15.3, CA-125, CA19.9) in the CSF may corroborate the diagnosis of LM in breast cancer patients.
Spinal Cord Compression
The differential diagnosis when considering ESCC includes benign musculoskeletal disease, spinal abscess, and radiation myelopathy. To diagnose ESCC, the metastatic tumor must extrinsically compress the thecal sac. Neurologic examination may lead to the clinical suspicion, but ultimately, this should be radiographically confirmed. Plain radiographs are insufficient to observe this compression, so MRI of the spine with and without contrast or myelography are the diagnostic tools of choice. These tests should be ordered promptly in patients with known breast cancer presenting with myelopathy/radiculopathy syndrome given the high prevalence of ESCC in this patient population.
The sensitivity and specificity of radiography for ESCC have been estimated at 91% and 86%, respectively, in patients with presenting with symptoms. False positives can occur, and MRI should be performed once ESCC is suspected for therapeutic planning as well. CT scanning is more sensitive than plain films but is not as sensitive or as specific as MRI.
A CT does not accurately distinguish the epidural space or spinal cord but is commonly used to direct percutaneous biopsies and help plan surgery. Isotope bone scans with Tc 99m are more sensitive than plain films for detecting bony metastases but not for judging the integrity of the epidural space and thecal sac. Thus a bone scan should not be used for diagnosing ESCC.
The best radiographic techniques for visualizing the epidural space and thecal sac, and therefore diagnosing ESCC, are myelography or MRI. Myelography has the following disadvantages: (1) it requires a lumbar puncture, (2) it is contraindicated if there is increased intracranial pressure or coagulopathy, (3) it is contraindicated if the subarachnoid space at the level of the spinal cord is obstructed, and (4) it does not provide soft tissue resolution. However, myelography is quick and thus more tolerable for a patient in pain, and CSF sampling can be performed at the time of lumbar puncture. Myelography followed by CT scanning (i.e., CT myelogram) provides additional information regarding spinal cord and root compression, but such information is not as useful as that provided by MRI, which has become the definitive imaging study for the evaluation of ESCC because of its widespread availability. MRI scans the spinal cord and column in multiple planes, thus providing unsurpassed visualization of intramedullary, intradural, extradural, and paraspinal lesions ( Fig. 73.2 ). Because of its clear superiority in sensitivity and specificity, any patient with known breast carcinoma who develops persistent back, neck, or radicular pain should have an MRI scan.
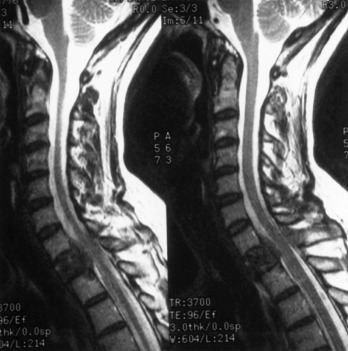
Treatment
Although brain metastases are almost uniformly fatal, several possible treatment modalities can ameliorate symptoms and possibly improve survival. These include corticosteroids, chemotherapy, RT alone or combined with surgical resection, and radiosurgery.
Prognosis
Historically patients with CNS metastases have a poor prognosis with medial OS ranging from 4 to 5.5 months with treatment. Without therapeutic intervention, life median OS is estimated at 1 to 2 months. For patients presenting with CNS recurrence neurologic disease is the cause of death and a major contributing factor to it in 68% of the cases as demonstrated in one series. Patients presenting with solitary lesions have better prognosis compared with patients with multiple brain metastases.
A prognostic prediction rule has been fostered in patients with brain metastases from solid tumors indicating that poor performance status and age over 65 years at diagnosis, along with uncontrolled extracranial disease, are variables that correlate with shorter median OS. Gaspar and coworkers reported data analysis of 1200 patients from three Radiation Therapy Oncology Group (RTOG) trials. Patients who had Karnofsky performance scores (KPS) less than 70 were classified in RPA class 3. To be classified into recursive partitioning analysis (RPA) class 1, a patient has to satisfy the following criteria: age less than 65 years, KPS greater than or equal to 70, primary site under control, and no extracranial metastases. According to this analysis, only 20% of brain metastases patients were classified as RPA class 1, whereas 65% and 15% were RPA classes 2 and 3, respectively ( Table 73.1 ). Other favorable prognostic factors identified by the study included a primary diagnosis of MBC ( p = .001) and having a single lesion ( p = .021). Aggressive treatment with surgery, RT, and chemotherapy should be used in patients with favorable prognoses (RPA class 1). Whole-brain RT (WBRT) alone should be used in patients with poor prognosis. These findings were in independently validated in a different data set. Another and more recent prognostic prediction rule, graded prognostic assessment (GPA), has been analyzed in an even larger data set of database of 1960 patients with brain metastasis from five RTOG clinical trials. The new index, the GPA, was derived from the database after analysis of many possible prognostic factors. These included age, sex, KPS, histologic characteristics, interval from initial diagnosis to time of presentation with brain metastases, and patients with brain and bone-only metastases. GPA classification was able to provide patient prognostic classification comparable to RPA but easier to use. Different data sets used to validate this new index yielded similar results.
RPA Class | Age | KPS | Primary Tumor | Extracranial Disease | Median Survival (Months) |
---|---|---|---|---|---|
1 | <65 | ≥70 | Controlled | Absent | 7.1 |
2 | ≥65 | ≥70 | Uncontrolled | Present | 4.2 |
3 | Any | <70 | Either | Either | 2.3 |
The TNBC subtype also correlated with worse prognosis. In one series of 136 patients with breast cancer brain metastasis treated with stereotactic radiosurgery (SRS), multivariate analysis showed an HR for death of 2.0 ( p = .006) in patients with TNBC even after controlling for number of lesions and activity of extracranial disease. Breast cancer with HER2 positivity and brain metastasis seem to have a better prognosis compared with historical controls. In one series of 377 with HER2-positive MBC and CNS metastasis, the estimated median OS was 26.3 months. Of note 53.9% of patients presented with more than one site of metastasis and 9.6% with LMD. Given that trastuzumab has negligible CNS penetrance, the improved prognosis could be secondary to improved systemic control with HER2-targeted therapy. In addition, retrospective clinical data support that patients with HER2-positive MBC and CNS metastasis, when treated with trastuzumab before or at the time of CNS metastasis diagnosis, live longer compared with patients not treated with trastuzumab. More recently a retrospective analysis of 1552 cases of breast and metastatic disease to brain showed that HER2 status, number of CNS metastasis, age at diagnosis, and performance status all have independent prognostic significance in RPA analysis.
Corticosteroids
Corticosteroids should be the first treatment administered for any patient with symptomatic CNS metastases in brain parenchyma and LMD. For ESCC, higher doses of corticosteroids are a mandatory part of initial management. Corticosteroids reduce vasogenic edema by decreasing capillary permeability and reduce inflammation by suppressing the migration of polymorphonuclear leukocytes, thereby decreasing pain and maintaining or improving neurologic function. A loading dose of 10- to 100-mg intravenous dexamethasone followed by maintenance dosing of 4 to 24 mg orally or intravenously every 6 hours has been commonly used. Steroid therapy is tapered during or after the course of treatment. High-dose corticosteroids have been studied with varied results, and there is no clear advantage to high-dose corticosteroids over standard dosing. Gastric prophylaxis with either an H2-receptor antagonist or a proton pump inhibitor should be given to all patients. Pneumocystis carinii pneumonia prophylaxis should be considered in patients who receive long-term corticosteroids. Corticosteroids may not be needed in asymptomatic patients with CNS metastases discovered by screening or in patients whose only symptoms are seizures, which may be best palliated with anticonvulsants.
Anticonvulsants
Seizures are a common manifestation of brain metastases. In a series of 195 patients with brain metastasis, approximately 18% of brain metastases patients present with seizures and an additional 10% develop seizures after diagnoses of a brain metastasis. The diagnosis of a seizure disorder is usually made clinically, and an electroencephalogram may be helpful if the diagnosis is in doubt. Seizure disorder secondary to brain metastases should be treated with first-line anticonvulsants such as levetiracetam, valproic acid, and/or carbamazepine among others. If the patient is receiving chemotherapy, anticonvulsants that do not induce hepatic cytochrome P450 enzymes should be considered (i.e., levetiracetam, lamotrigine, or topiramate). Treatment should be initiated with a single agent to increase compliance and avoid side effects associated with multidrug treatment. Prophylactic anticonvulsant therapy should not be used in brain metastases patients without seizure disorders. A meta-analysis of randomized trials that assessed the use of prophylactic anticonvulsant therapy in brain tumors found that there was no evidence to support prophylactic anticonvulsant therapy. The American Academy of Neurology recommends that prophylactic anticonvulsant therapy should not be used for brain metastases patients. Perioperative anticonvulsant therapy is often used after neurosurgery for patients with brain metastases without a diagnosis of a seizure disorder because of concerns that surgery increases the risk of seizure. A meta-analysis failed to show a statistically significant benefit to the use of prophylactic anticonvulsant therapy after craniotomy. The American Academy of Neurology recommends that anticonvulsant therapy should be tapered within 1 week after neurosurgery in patients who do not develop a postoperative seizure disorder.
Anticonvulsant therapy may impair neurocognitive function and quality of life and as such should be used only in patients with known seizures. Prophylactic anticonvulsant therapy should not be used in newly diagnosed brain metastases patients who do not have a seizure disorder, and anticonvulsant medications should be promptly discontinued in the perioperative setting.
Whole-Brain Radiation Therapy
WBRT is considered the standard of care in patients with more than three brain metastases. The role of RT in the management of brain metastases has been well established. By the mid-1950s, it was recognized that WBRT improved survival and symptoms in patients with brain metastases. According to one series, WBRT improved neurologic symptoms in 74% of patients treated, and two-thirds of these patients had lasting improvement until death. The complete response rate for brain metastases from breast cancer, assessed by CT, treated with 3000 cGy in 10 fractions has been reported to be 35% among 46 patients, which indicates brain metastases are responsive to palliative radiation therapy. Several retrospective studies have shown that patients with brain metastases from breast cancer typically have a median survival of 4 to 6 months and a 70% to 90% improvement in symptoms with WBRT alone. However, 31% to 68% of the patients die of neurologic complications. Prognostic factors identified in these studies were KPS, radiation dose, and extracranial disease. It is difficult to assess improvement of symptoms after WBRT because the evaluation is subjective and may be influenced by the patient’s general well-being and use of corticosteroids. The RTOG reported a 70% to 80% improvement in the patients’ palliative index (a four-point functional activity index) after radiation. Several retrospective studies and a randomized trial have established the role of adjuvant WBRT after resection of a single brain metastasis in solid tumors including breast cancer. The use of adjuvant WBRT is discussed in the surgical treatment of brain metastases section.
Treatment Technique and Dose Fractionation
Radiation is delivered through opposed lateral fields designed to treat the cerebrum, cerebellum, brainstem, and upper spinal cord (usually down to C1–C2). The most common fractionation regimen used in the United States is 3000 cGy in 10 fractions. However, because brain metastases are almost always associated with incurable disease, many investigators have sought radiation treatment regimens that would ease the burden of therapy in these patients. Using larger fraction sizes and a shorter overall treatment time may decrease the patient’s time away from family and friends, achieve a greater biological effect, and minimize the use of medical resources.
The RTOG published three studies evaluating different fractionation regimens in patients with brain metastases. Patients in these studies were assigned to 4000 cGy in 20 fractions, 4000 cGy in 15 fractions, 3000 cGy in 15 fractions, 3000 cGy in 10 fractions, or 2000 cGy in 5 fractions. Patients with breast cancer accounted for 16% to 18% of the participants in these studies. The overall response rate for symptom palliation (75%–80%) and median survival (15–18 weeks) were similar for all the dose fractionation schedules. Breast cancer patients had a mean survival of 21 weeks. A subsequent study compared 3000 cGy in 10 fractions with 5000 cGy in 20 fractions and saw no survival difference with a median survival of only 17 weeks. Only 7% of these patients had breast cancer. The RTOG has also investigated dose escalation and accelerated fractionation schedules, but to date neither has shown an improvement in survival.
A French study compared WBRT of 1800 cGy in three fractions with or without an additional 2500 cGy in 10 fractions. In this study, 29% of the patients had breast cancer. No significant difference was noted in survival. Another study from the United Kingdom compared 3000 cGy in 10 fractions over 2 weeks with 1200 cGy in 2 fractions separated by 1 week. Although there was a significant survival difference in favor of the longer fractionation regimen (84 vs. 77 days), many prefer the shorter regimen, given the questionable clinical significance of 7 days survival.
On the basis of available data, no dose fractionation schedule is superior. The standard and most often prescribed dose fractionation schedule for WBRT is 3000 cGy in 10 fractions. One may consider shorter (2000 cGy in 5 fractions) or longer dose (4500 cGy in 20–25 fractions) schedules based on the patient’s projected prognosis and/or severity of symptoms.
Toxicity of Whole-Brain Radiation Therapy
During WBRT, side effects are mild and generally tolerable. Possible acute toxicities include alopecia, serous otitis media, radiation dermatitis, nausea and vomiting, and myelosuppression. Radiation dermatitis and partial or complete alopecia are common. A dose-response relationship for the hair follicle has been reported to correlate with permanent alopecia and previous chemotherapy may increase the risk of radiation-induced alopecia. Nausea and vomiting are infrequent, as is myelosuppression. Occasionally, a patient’s neurologic symptoms can worsen during WBRT secondary to worsening cerebral edema. If a patient’s neurologic symptoms should acutely worsen, corticosteroids should be started; if the patient is already on corticosteroids, the dose should be increased in the short term. Acute encephalopathy may occur with fraction sizes larger than 300 cGy. The patient has symptoms of severe headache, nausea, drowsiness, and focal neurologic deficits and fever. The physiologic mechanism is thought to be radiation damage to the BBB resulting in cerebral edema. Death may occur secondary to cerebral herniation. The incidence of acute encephalopathy is low; in one series from 1970s, 6% of patients were dead 48 hours after receiving a single fraction of 1000 cGy with cobalt-60. Diffuse enhancement is often seen on brain MRI scans; it is not correlated with acute toxicity and resolves after WBRT.
Possible late toxicities include somnolence syndrome, radiation necrosis, and neurocognitive impairment. Somnolence syndrome is a subacute effect of cranial irradiation that occurs more commonly in pediatric patients 1 to 6 months after WBRT and is characterized by extreme sleepiness and signs of increased intracranial pressure (i.e., headache, nausea, vomiting, anorexia, and irritability). Somnolence syndrome is rare in adults; it resolves spontaneously and does not result in late effects. Radiation necrosis and neurocognitive impairment are possible late effects (>90 days after completing WBRT). Radiation necrosis is highly unlikely with standard dose fractionation schedules used for palliative WBRT (i.e., 3000 cGy in 10 fractions) and is uncommon with doses less than 5000 cGy in 25 fractions. The risk of radiation necrosis increases with increasing dose and fraction size as well as the use of subsequent chemotherapy. The incidence of radiation necrosis is 5% with doses of 5500 to 6000 cGy, although these doses are not typically used for WBRT. Radiation necrosis is more common after SRS. The rate of necrosis in patients treated with SRS with or without WBRT is 4% to 6%.
Some have argued, based on retrospective data, that neurocognitive function and quality of life decline after WBRT and that therefore SRS is preferable to WBRT. Neurocognitive dysfunction is often multifactorial and distinguishing the cause of a patient’s mental deterioration may be difficult. The brain metastasis(es) itself, progressive disease, neurosurgical procedures, anticonvulsants, steroids, narcotic medications, chemotherapy, and/or RT can all contribute to neurocognitive dysfunction. Several other variables may influence the development of neurocognitive dysfunction, including total radiation dose, fraction size, volume of brain irradiated, concurrent chemotherapy, age, and presence of diabetes mellitus. The pathophysiologic mechanism is thought to be vascular damage and demyelination from RT. A phase III randomized trial that assessed the efficacy of motexafin gadolinium in brain metastases patients receiving WBRT documented that 90% of brain metastases patients have baseline neurocognitive impairment before any therapy. Higher baseline neurocognitive function correlated with improved survival. A subsequent analysis of 208 patients from this trial showed that patients who had a good response (>45% tumor shrinkage) had better neurocognitive function preservation. Those with progressive disease in the brain had worsening neurocognitive function. A question also remains regarding the feasibility and risks of sparing the subgranular zone of the hippocampus during WBRT for brain metastases as a strategy to reduce the risk of neurocognitive decline. Despite the provocative results of small studies using hippocampal sparing WBRT with cancer CNS metastases indicating stable scores of neurocognitive function, further validation of this new approach is warranted.
Furthermore, in the RTOG 0214 patients with stage III non–small cell lung cancer treated with prophylactic WBRT to total dose of 30 Gy/15 fractions once daily compared with observation had no significant differences in global cognitive function (Mini–Mental State Examination [MMSE]) or quality of life after prophylactic WBRT, but there was a significant decline in memory at 1 year.
Hyperfractionated RT has been used to spare normal tissues, and its ability to spare neurocognitive function has been tested. RTOG 94-01 tested whether accelerated hyperfractionation (160 cGy twice daily to 5440 cGy) improved neurocognitive outcome in brain metastases patients receiving WBRT. The patients treated with accelerated hyperfractionation did not have significantly different neurocognitive outcomes compared with the patients treated with 3000 cGy in 10 fractions. Furthermore, the rate of decline in neurocognitive function at 3 months was smaller for those whose brain metastases were radiologically controlled compared with those with uncontrolled brain metastases ( p = .02). Aoyama and colleagues published an analysis of neurocognitive function in patients with one to four brain metastases in a randomized trial comparing WBRT plus SRS with SRS. The 1-, 2-, and 3-year actuarial free rate of a three-point drop in MMSE scores was 76.1%, 68.5%, and 14.7%, respectively, in the WBRT plus SRS arm versus 59.3%, 51.9%, and 51.9%, respectively, in the SRS-alone arm. The average duration until MMSE deterioration was longer in the WBRT plus SRS patients (16.5 vs. 7.6 months, p = .05). Tumor progression was found to be the cause of neurocognitive decline more often in patients treated with SRS alone, meaning that neurocognitive decline is most likely caused by brain metastases progression and not radiation damage to the brain. However, survival is limited in patients with brain metastases, and they may not survive long enough to develop RT-induced neurocognitive impairment.
A smaller randomized trial evaluating SRS alone versus SRS + WBRT was stopped after the first 58 patients. WBRT was prescribed to a total dose of 30 Gy given in 12 daily fractions of 2.5 Gy per day. There was a high probability (96%) that patients randomly assigned to receive SRS plus WBRT were significantly more likely to show a decline in learning and memory function (mean posterior probability of decline 52%) at 4 months than patients assigned to receive SRS alone (mean posterior probability of decline 24%). At 4 months there were four deaths (13%) in the group that received SRS alone, and eight deaths (29%) in the group that received SRS plus WBRT. Seventy-three percent of patients in the SRS plus WBRT group were free from CNS recurrence at 1 year, compared with 27% of patients who received SRS alone ( p = .0003).
Finally, recent meta-analysis of randomized controlled trials comparing surgery or SRS plus WBRT with surgery or SRS alone for treatment of brain metastases failed to capture the potential negative impact for WBRT on neurocognitive function and health-related quality of life.
Surgery
Surgery can rapidly relieve neurologic symptoms from the mass effect of a brain metastasis and provide local control and a histologic diagnosis. Before recommending surgery, oncologists must consider the patient’s age, KPS, status of extracranial disease, location of the brain metastasis, and the number of brain metastases. If surgery is necessary, perioperative anticonvulsant therapy should be considered.
Surgical Intervention for Single Metastasis
There is considerable randomized evidence suggesting that surgical resection of brain metastasis before WBRT improves quality of life and lengthens survival. These data have been confirmed by multiple clinical trials, three which compared WBRT to WBRT plus surgical resection, and one which compared WBRT plus surgery to surgery alone ( Table 73.2 ).
Patient n | Arms | Local Recurrence | Distant Recurrence in Brain | Median OS | Neurologic Death | Median Time to Neurologic Death | |
---|---|---|---|---|---|---|---|
Patchell, 1990 | 48 | WBRT vs. WBRT + surgery | 52% vs. 20% ( p < .02) | 13% vs. 20% ( p = .52) | 14 vs. 40 wk ( p < .01) | NS | 26 vs. 62 wk ( p < .0009) |
Vecht, 1993; Noordijk, 1994 | 63 | WBRT vs. WBRT + surgery | NS | NS | 24 vs. 40 wk ( p = .04) | NS | NS |
Mintz, 1996 | 84 | WBRT vs. WBRT + surgery | NS | NS | 22 vs. 25 wk ( p = .24) | NS | NS |
Patchell, 1998 | 95 | Surgery vs. WBRT + surgery | 46% vs. 10% ( p < .001) | 37% vs. 14% ( p < .01) | 43 vs. 48 wk ( p = .39) | 44% vs. 14% ( p = .003) | 81 vs. 115 wk ( p = .03) |
Patchell and colleagues published the first randomized trial comparing WBRT versus WBRT plus surgery in patients with a single brain metastasis. Adding surgical resection of a single brain metastasis decreased local recurrence and lengthened both OS and time to neurologic death. The percentage of patients who developed a recurrence at the site of the original metastasis was only 20% in the WBRT plus surgery arm versus 52% in the WBRT arm ( p < .02). Of the patients with recurrences at the site of the original metastasis, the length of time from treatment to recurrence was a median of 59 weeks in the WBRT plus surgery arm versus a median of 21 weeks in the group treated with WBRT alone ( p < .001). There was no difference in recurrences elsewhere in the brain (13%–20%, p = .52). When deaths from only neurologic causes were used as end points, the surgical group had a median neurologic survival of 62 weeks, whereas the group treated with WBRT alone had a neurologic survival of only 26 weeks ( p < .0009). The median OS was longer in the WBRT plus surgery arm (40 vs. 14 weeks, p < .01). The surgical group maintained KPS ratings of more than 70% for a median of 38 weeks, whereas the group treated with RT alone maintained a functionally independent level for a median of only 8 weeks ( p < .005). The treatment morbidity and mortality were similar. One caveat to applying the findings of this study to patients with MBC is that only 3 of the 48 patients (6%) studied had breast carcinoma as their primary tumor; 37 (77%) of the patients had non–small-cell lung carcinoma.
A randomized Dutch trial also showed an OS benefit from adding surgery to WBRT. Investigators from this study randomized 63 patients (19% with breast primaries) with a single brain metastasis to either WBRT alone or WBRT plus surgery. The median OS was 24 weeks in the WBRT-alone arm and 40 weeks in the WBRT plus surgery arm ( p = .04). Furthermore, the median OS was 5 months for both arms if the patients had active extracranial disease. A Canadian multicenter randomized trial failed to show a survival benefit from adding surgery to WBRT. Eighty-four patients were randomized, and the median OS was 22 weeks in the WBRT-alone arm and 25 weeks in the WBRT plus surgery arm ( p = .24). The authors suggest that patient selection may have negated the survival benefit seen in the two aforementioned trials. The Canadian trial included patients with a lower baseline median KPS; a higher proportion of them (50%) had extracranial metastases. In addition, 10 of 43 patients randomized to the WBRT-alone arm received surgical resection.
These trials have established that adding surgical resection before WBRT improves survival and quality of life in cancer patients with a single brain metastasis. Patchell and colleagues completed another randomized trial to assess the benefit of WBRT after resecting a single brain metastasis. Ninety-five patients were randomized to surgery alone or WBRT plus surgery. Adding postoperative WBRT reduced local recurrence, distant brain recurrences, and neurologic death. The absolute reduction of any brain failures, either local or distant, was 50% when giving WBRT after surgical resection of a single brain metastasis ( p < .001). Neurologic deaths were also fewer in the WBRT plus surgery arm (14% vs. 44%, p = .003). However, OS was not significantly different between the two arms (43–48 weeks, p = .39). On multivariate analysis, the use of WBRT predicted for better neurologic survival ( p < .009). There was no difference in median time to KPS decline below 70 (35–37 weeks). There were more deaths from systemic disease in the WBRT plus surgery arm, probably because of the higher rates of neurologic death in the surgery-alone arm. Taken together, the results of these trials support that WBRT in conjunction with surgery leads to better clinical outcomes than WBRT alone for good performance patients with solitary metastatic intracranial lesions. With recent advances and concern for quality-of-life detriment with WBRT, SRS has been increasingly used in brain metastases patients including in patients with more than three metastases
Surgery for Multiple Metastases
The role of surgery for multiple brain metastases is controversial, and the data are limited to single-institution retrospective series. Bindal and coworkers conducted a retrospective review of 56 patients who underwent surgery in the setting of multiple cerebral metastases. These patients were divided into two groups: one in which one or more brain metastases were left unresected (n = 30) and another in which all the brain metastases were resected (n = 26). An additional group of patients treated with complete surgical resection of a single metastasis was also evaluated (n = 26). All three groups were matched on the basis of important clinical factors. The group with multiple metastases and at least one metastasis left untreated had a median survival of 6 months, and the two groups with complete resection of their multiple or single metastases each had a median survival of 14 months ( p > .5). Thus the median OS was significantly worse in the patients who had one or more unresected brain metastases ( p < .05). Recurrence of brain metastases was similar for patients who had complete resection(s) of their multiple or single brain metastases (31%–35%, p > .05). The complication rate for patients undergoing multiple craniotomies was 8% to 9%, and it was 8% for patients undergoing a single craniotomy. The 30-day mortality rate was 3% to 4% for patients with multiple brain metastases and 0% for patients with a single brain metastasis. The authors concluded that resection of all lesions in selected patients with multiple brain metastases resulted in a prognosis similar to that of patients undergoing surgery for a single brain metastasis. Of note, only 11 patients in this series had breast cancer.
In another retrospective series, 70 breast cancer patients with brain metastases had surgery as part of their treatment. Fifty-four patients had single brain metastases and 16 had multiple brain metastases; there was no statistical difference in survival between these groups of patients (median survival, 13.9–14.8 months, p = .28). The median survival of 22 patients with positive hormonal receptor ER or progesterone receptor (PR) was significantly longer than the median survival of 20 patients with negative ER/PR (21.9 vs. 12.5 months, p < .05).
These retrospective studies should be interpreted with caution because they may have included highly selected patients. No prospective trial evaluating surgery for multiple metastases has been performed; therefore the role of surgery for these patients is not clearly defined. Furthermore, these studies were not designed to address the fundamental question of breast cancer subtype and the value of surgical approach to CNS brain metastases. Surgical decision should be individualized based on pattern of disease progression, control of systemic disease, number, size and location of brain metastasis (es) and patient performance statues, comorbidities, and expectations.
Stereotactic Radiosurgery
SRS was developed by the Swedish neurosurgeon Lars Leksell in the 1950s and has become increasingly popular for the treatment of single or a limited number of brain metastases. SRS is a noninvasive option to neurosurgery, especially for brain metastases in difficult to access areas or for patients who cannot have traditional surgery, and it can be combined with WBRT. Through the use of multiple convergent beams, SRS delivers a large dose of radiation in one or a few fractionated treatments to a limited target volume. The hallmark of SRS is the rapid dose falloff with increasing distance from the target, which allows for the sparing of nontarget normal tissues in the brain. SRS can be delivered with high-energy x-rays from linear accelerators (i.e., LINAC, CyberKnife), gamma rays from multiple cobalt-60 sources (i.e., Gamma Knife), or via charged particles such as protons. Compared with fractionated RT, SRS has a radiobiological advantage. The delivery of a single high dose of radiation results in a higher proportion of cellular death and cell cycle arrest. Brain metastases must be less than or equal to 3 cm and adequately distant from the optic apparatus for SRS. Doses may range from 1500 to 3500 cGy. Studies have shown that SRS for a single brain metastasis results in a 1-year local control rate of 80% to 95% and median survival of 7 to 13 months.
Stereotactic Radiosurgery Versus Surgery
There are several advantages to using SRS instead of surgery. First, SRS is a noninvasive, outpatient procedure that can be used to treat single or multiple metastases. It can also be used to safely treat surgically inaccessible brain metastases or brain metastases that result in significant neurologic morbidity when surgically resected. Also, SRS has been reported to be more cost-effective than surgery. Mehta and colleagues analyzed the total cost of treatment for WBRT, WBRT plus surgery, and WBRT plus SRS. The average cost per week of survival was $310 for WBRT, $524 for WBRT plus surgery, and $270 for WBRT plus SRS. However, symptomatic patients with surgically accessible lesions are best treated by surgery, because surgery allows for immediate decompression and symptom relief. Also, neurologic complications and local failures increase for brain metastases greater than 3 cm treated with SRS.
There are no randomized trials comparing SRS with surgery, but some retrospective series have suggested that SRS is as effective as surgical resection. Most of these studies analyzed patients treated with WBRT plus SRS. Auchter and colleagues reviewed 122 patients (10% with primary breast tumors) who had only one brain metastasis and were candidates for surgical resection followed by WBRT but were treated with WBRT and SRS. The median follow-up and survival were 123 weeks and 13 months, respectively. The median SRS and WBRT doses were 1700 and 3750 cGy, respectively. The overall local control rate for the areas treated with SRS was 86%. Twenty-seven patients (22%) had distant intracranial recurrences. The median duration of functional independence was 10 months. These results are comparable to WBRT plus surgery arms in the trials published by Patchell and colleagues and Noordijk and colleagues Another retrospective study compared patients with a single brain metastasis who were eligible for surgical resection or SRS. In this investigation, 74 patients who had surgery were compared with 24 patients who had SRS. Also, 82% of the surgery patients and 96% of the SRS patients were treated with WBRT ( p = .172). There was no difference in survival (median survival of 12 months; 1-year survival of 56% for the SRS patients and 62% for the surgery patients, p = .15). There were no local failures for the patients treated with SRS, whereas 19 (56%) patients treated with surgery had local failures. Bindal and colleagues performed a similarly matched comparison between patients treated with surgery versus SRS. The median SRS dose was 2000 cGy. They observed a significantly worse median survival for patients treated with SRS on multivariate analysis (7.5 months for SRS vs. 16.4 months for surgery, p = .0009). The authors stated that the worse survival in patients treated to SRS was the result of a greater progression rate of the radiosurgically treated lesions ( p = .0001). One additional study retrospectively analyzed 206 patients (19% had breast cancer) in RPA classes 1 and 2 who had one or two brain metastases. The authors compared patients treated with SRS alone to those treated with surgical resection and WBRT. Comparison of the two groups showed no significant difference for OS ( p = .19), brain control ( p = .52), or local control ( p = .25).
Most recently, Yamamoto and colleagues conducted a prospective observational study of 1194 patients with untreated multiple brain metastases. Patients with 1 to 10 newly diagnosed brain metastasis (<3 cm in longest diameter) and a KPS score of 70 or higher were enrolled. Ten percent of the patients had MBC. Tumor volumes smaller than 4 mL were irradiated with 22 Gy at the lesion periphery and those that were 4 to 10 mL with 20 Gy. The primary end point was OS. Median OS after stereotactic radiosurgery was 13.9 months (95% confidence interval [CI] 12.0–15.6) in the 455 patients with one tumor, 10.8 months (95% CI 9.4–12.4) in the 531 patients with two to four tumors, and 10.8 months (95% CI 9.1–12.7) in the 208 patients with 5 to 10 tumors. The results suggested that stereotactic radiosurgery without WBRT in patients with 5 to 10 brain metastases is noninferior to that in patients with two to four brain metastases.
These results have supported the use of SRS as an upfront treatment approach for patients with increasing number CNS metastatic disease with less than 3 cm greatest dimension. Per National Comprehensive Cancer Network guidelines, WBRT remains indicated for patients with more than three metastases; however, in clinical practice, SRS is becoming more widely used for more than three lesions. These new parameters are largely determined institutionally until national guidelines change to match this evolving perspective.
Whole-Brain Radiation Therapy Versus Whole-Brain Radiation Therapy Plus Stereotactic Radiosurgery Boost
Given that SRS has a similar efficacy to that of surgical resection, investigators have studied the possible benefit of adding SRS to WBRT. A multiinstitutional retrospective review was conducted comparing patients treated with WBRT alone to those treated with WBRT and SRS, and 502 patients were eligible and stratified according to RPA class. The addition of SRS increased survival. The median survival rates for patients treated with WBRT plus SRS versus WBRT alone stratified by RPA class were 16.1 versus 7.1, 10.3 versus 4.2, and 8.7 versus 2.3 months for classes 1, 2, and 3, respectively ( p < .05).
Two randomized trials have compared WBRT to WBRT plus SRS boost. Kondziolka and coworkers published a randomized trial of 27 patients (14 to WBRT alone and 13 to WBRT plus SRS; Table 73.3 ). Patients had two to four brain metastases. Fifteen percent of the patients in this study had primary breast cancer. The WBRT dose was 3000 cGy in 12 fractions, and the SRS dose was 1600 cGy. The trial was stopped early after the interim analysis, which revealed a significant benefit in the rate of local tumor control after WBRT plus SRS ( p = .0016). The local failure rate at 1 year was 100% in the WBRT-alone arm versus 8% in the WBRT plus SRS arm, with a corresponding median time to local failure of 6 and 36 months ( p = .0005). Survival was not statistically different between the two arms: patients who received WBRT alone lived a median of 7.5 months, whereas those who received WBRT plus SRS lived 11 months ( p = .22).
No. of Patients | Arms | One-Year Local Control | Median Overall Survival | Distant Recurrence in Brain | Neurologic Death | |
---|---|---|---|---|---|---|
Kondziolka 1999 | 27 | WBRT vs. WBRT + SRS | 0% a vs. 91% | 7.5 vs. 11 mo ( p = .22) | NS | NS |
RTOG 9508 | 333 | WBRT vs. WBRT + SRS | 71% vs. 82% ( p = .0132) | 5.7 vs. 6.5 mo ( p = .1356) b | NS | 31% vs. 28% ( p = NS) |
Chougule 2000 | 109 | SRS vs. WBRT + SRS vs. WBRT | 87% vs. 91% vs. 62% ( p = NS) | 7 vs. 5 vs. 9 mo ( p = NS) | 43% vs. 19% vs. 23% ( p = NS) | NS |
JRSOG-9901 | 132 | SRS vs. WBRT + SRS | 72.5% vs. 88.7% ( p = .002) | 8 vs. 7.5 mo ( p = .42) | 63.7% vs. 41.5% ( p = .003) | 19.3% vs. 22.8% ( p = .64) |
a This includes patients who died before 1 year and those still living, all with tumor progression.
b Survival advantage was seen in patients with a single brain metastasis treated with WBRT + SRS (6.2 vs. 4.9 mo, p = .0393).
The RTOG conducted a multiinstitutional phase III trial comparing WBRT alone to WBRT plus SRS boost for patients with one to three unresectable brain metastases. In this study, 333 patients were randomized, 10% of whom had primary breast cancer, and patients were stratified according to the number of brain metastases (one vs. two and three). The WBRT dose was 3750 cGy in 15 fractions, and the SRS doses were 2400 cGy (≤2 cm), 1800 cGy (>2 cm but ≤3 cm), and 1500 cGy (>3 cm but ≤4 cm). The overall median survival for the WBRT group was 5.7 months, and for the WBRT plus SRS group, it was 6.5 months ( p = .1356). The median survival for patients with a single brain metastasis treated with WBRT plus SRS boost was significantly better at 6.5 versus 4.9 months ( p = .0393). On multivariate analysis, RPA class I predicted better survival than RPA class II ( p < .0001). The 1-year local control was better for patients treated with WBRT plus SRS (82% vs. 71%, p = .0132). Local recurrence was 43% more likely in the WBRT-alone arm ( p = .0021). Patients in the WBRT plus SRS arm were more likely to have a stable or improved KPS at 6-month follow-up than were patients allocated to the WBRT-alone arm (43% vs. 27%, respectively, p = .03) and were able to taper steroid use sooner. Acute toxicities of grade 3 or greater were reported in 3% of the WBRT plus SRS patients versus none of the WBRT patients. Late toxicities of grade 3 or greater were reported in 6% versus 3% of WBRT plus SRS and WBRT patients, respectively. The authors concluded that WBRT plus SRS should be the standard treatment for patients with an unresectable single brain metastasis and considered for patients with multiple brain metastases.
Stereotactic Radiosurgery Versus Whole-Brain Radiation Therapy
Some have argued that because of the possible neurocognitive impairment caused by WBRT, SRS alone could be used for selected patients with WBRT reserved for salvage treatment. Numerous retrospective studies have compared SRS alone to WBRT alone. These studies have shown that survival is similar between patients treated with SRS alone versus WBRT alone but that local and distant failures in the brain are higher in patients treated with SRS alone. Two randomized trials have compared SRS alone to WBRT plus SRS. Chougule and colleagues randomized 109 patients with three brain metastases or fewer to Gamma Knife (GK) radiosurgery, GK plus WBRT, or WBRT alone. Currently data are published only in abstract form. The majority of patients in this study had primary breast cancer (62 patients, 57%). The radiation doses for the three arms of the study were as follows: GK-alone arm, 3000 cGy; WBRT plus GK arm, 3000 cGy WBRT and 2000 cGy GK boost; and WBRT-alone arm, 3000 cGy. Of note, 51 patients (47%) had surgical resections of large, symptomatic brain metastases before randomization. The median OS was similar for each treatment arm: 7, 5, and 9 months for the GK, GK and WBRT, and WBRT-alone arms, respectively. Local control appeared to be better in the GK arms: 87%, 91%, and 62% for GK, GK plus WBRT, and WBRT-alone arms, respectively. The incidence of new brain metastases was lower in the two arms that received WBRT: 43%, 19%, and 23% for GK, GK plus WBRT, and WBRT-alone, respectively.
A multiinstitutional phase III randomized trial from Japan (JRSOG-9901) reported results of treating 132 patients with one to four brain metastases (each ≤3 cm) with either SRS alone or WBRT plus SRS. The radiation dose for the SRS-alone arm was 2200 to 2500 cGy for lesions 2 cm or smaller and 1800 to 2000 cGy for lesions greater than 2 cm. In the WBRT plus SRS arm, the SRS dose was 30% lower. The WBRT dose was 3000 cGy in 10 fractions. Only nine (7%) patients in this study had breast cancer. The median follow-up was 7.8 months. The primary objective was survival; however, the trial was terminated early because of the significant difference in brain metastasis recurrence rates. Thus criticisms of this trial are that it was insufficiently powered to observe a potential survival difference and that 805 patients would have to be enrolled to detect a significant difference in survival. There were no differences in median survival (8 vs. 7.5 months) or neurologic death (19.3% vs. 22.8%, p = .64). The multivariate survival analysis revealed that age less than 65 years, stable primary tumor status, stable extracranial metastases status, and KPS score 90 to 100 significantly predicted better survival. The 1-year local control rate was significantly higher for the WBRT plus SRS arm than for the SRS-alone arm (88.7% vs. 72.5%, p < .001). The 1-year distant brain recurrence rate was lower for patients treated with WBRT plus SRS: 41.5% versus 63.7% ( p = .003). On multivariate analysis, receiving WBRT plus SRS, having stable extracranial metastases, and having a KPS score of 70 to 80 predicted fewer distant brain recurrences. The number of brain metastases did not predict for survival or distant brain recurrence. Salvage treatment for progression of brain tumor was required significantly more frequently in the SRS-alone group (29 patients) than in the WBRT plus SRS group (10 patients, p < .001). Neurologic preservation (maintenance of KPS ≥70) was the same for each arm. There was one grade 3 or greater acute neurotoxicity reported in the WBRT plus SRS arm and two in the SRS-alone arm. There were four grade 3 or greater late neurotoxicities in the WBRT plus SRS versus two in the SRS-alone arm.
In another prospective study, 58 patients with one to three newly diagnosed brain metastases were randomly assigned to SRS plus WBRT or SRS alone. The trial was stopped in light of significantly more decline in learning and memory function in the WBRT plus SRS group compared with the SRS only cohort.
These results favor the use of SRS for patients with asymptomatic oligometastatic disease to brain with less than 3 parenchymal lesions compared with WBRT.
Chemotherapy
Historically, the main treatment of brain metastases has been radiation. Chemotherapy was not thought to be effective given the belief that hydrophilic drugs and/or large molecules could not pass the BBB. The multidrug-resistance gene (P-glycoprotein) is known to be highly expressed by the endothelium of brain capillaries. It was typically thought that only small lipid-soluble molecules could pass the BBB, but this theory has been questioned. Metastatic tumors are known to have abnormal vascular supply in both function and anatomy, and thus the BBB may not be functioning normally. Some investigators have used BBB-disruption agents in conjunction with cytotoxic chemotherapy. A list of chemotherapy agents that can or cannot cross the BBB can be found in Table 73.4 . A problem with all of the phase I and II trials assessing chemotherapy in brain metastases patients is that the patient populations in these trials have been heavily pretreated and often the shortcoming of treatment is not necessarily BBB penetrance but rather aggressive disease biology from more resistant cancers.
