- Regular exercise increases insulin sensitivity in both individuals with and without diabetes.
- In individuals without diabetes, plasma insulin levels decrease during low to moderate intensity exercise to compensate for increases in insulin sensitivity. Glucose production and glucose disposal increase in parallel in order to maintain blood glucose homeostasis.
- In individuals with type 1 diabetes (T1DM), low to moderate intensity exercise can result in hypoglycemia, as insulin levels cannot be regulated physiologically.
- During and after high intensity exercise, glucose production can exceed glucose disposal, causing hyperglycemia in individuals both with and without diabetes. In T1DM, hyperglycemia can be more marked and prolonged, as insulin cannot increase in response.
- It is recommended that individuals with T1DM adjust their insulin dose and carbohydrate consumption prior to, during and/or after exercise to accommodate the type, intensity and duration of exercise performed.
- While regular exercise has not conclusively been found to improve glycemic control in T1DM, it is associated with decreased long-term morbidity and mortality in this population.
- Structured supervised diet and exercise interventions can reduce the risk of developing type 2 diabetes mellitus (T2DM) by about 60% in individuals with impaired glucose tolerance.
- Regular exercise improves glycemic control significantly in T2DM.
- Individuals with T1DM and T2DM with moderate or high aerobic fitness have long-term mortality that is 50–60% lower than individuals with diabetes and low cardiorespiratory fitness.
- Resistance training is a safe and effective means of improving glycemic control in individuals with T2DM of all ages. To maximize the impact of lifestyle measures on glycemic control, combined aerobic and resistance exercise is more effective than either type of exercise alone.
Glossary of terms
The definitions in Table 23.1 are adapted from those found in the Physical Activity Guidelines Advisory Committee Report, 2008 produced by the US Department of Health and Human Services [1].
Table 23.1 Glossary of terms.
Physical activity The expenditure of energy above that of resting by contraction of skeletal muscle to produce bodily movement |
Exercise A type of physical activity that involves planned, structured and repetitive bodily movement performed for the purpose of improving or maintaining physical fi tness |
Cardiorespiratory fitness/cardiorespiratory endurance/aerobic fitness Refers to the circulatory and respiratory systems’ ability to supply oxygen during sustained exercise |
Aerobic exercise Exercise that uses primarily aerobic energy-producing systems and involves the repeated and continuous movement of the same large muscle groups for extended periods of time (at least 10 minutes at a time). If performed with suffi cient intensity and frequency this type of exercise increases cardiorespiratory fitness. Aerobic activities include walking, cycling, jogging, swimming, etc. |
Anaerobic exercise Short, high intensity exercise involving anaerobic energy-producing systems. If performed with suffi cient intensity and frequency this type of exercise can increase the body’s ability to tolerate acid-base imbalance during high intensity exercise |
Intensity of aerobic exercise Generally described in relation to an individual’s maximal aerobic capacity ( ![]() ![]() ![]() |
Muscular fitness Includes the force a muscle can exert (strength) and the ability of the muscle to perform continuously without fatigue (endurance) Resistance exercise Also known as strength training or weight training. Resistance exercise involves the use of muscular strength to work against a resistive load or move a weight. Examples include lifting free weights, or using weight machines. Regular resistance exercise at suffi cient (moderate to high) intensity increases muscular fitness |
Intensity of resistance exercise The intensity of resistance exercise is often considered “moderate” if the resistance provided is 50–74% of the maximum that can be lifted a single time (1 repetition maximum [1 RM]). High intensity resistance exercise involves resistance ≥75% of 1 RM |
Repetition The number of times a resistance exercise is repeated during each set |
Set A grouping of repetitions of a specifi c resistance exercise |
Types of exercise training and their effects on healthy individuals
Aerobic exercise
Effects of regular aerobic exercise training
Regular aerobic training generally leads to improved lung function and cardiac output, allowing for the provision of more oxygen for working muscles. Higher plasma volume and greater capillary density in the muscles allow increased muscle blood flow during peak exercise. Eventually, a shift in the fuels being used to supply the energy demands of activity is seen as the oxidative metabolic system becomes more efficient. In general, changes become more noticeable when training is of higher volume and intensity.
Fuel metabolism during acute aerobic exercise
During the first 5–10 minutes of exercise, muscle glycogen is the main source of energy. As exercise continues, the bloodborne substrates, glucose and non-esterified fatty acids become increasingly important. If exercise of moderate intensity continues for several hours, the contribution of glucose diminishes and non-esterified fatty acids become the major fuel (Figure 23.1) [2]. During moderate intensity aerobic exercise, blood glucose levels remain virtually unchanged. Insulin secretion is reduced while the release of glucagon is promoted, encouraging a two- to fourfold increase in hepatic glucose production (controlled by the glucagon: insulin molar ratio at the portal vein) [3] to meet the needs of the exercising muscle. Glucose production and utilization fall rapidly and in parallel to baseline during the post exercise “recovery” phase, as insulin levels rise and glucagon secretion decreases. If moderate intensity aerobic exercise lasts for several hours without caloric intake, hepatic glucose production can no longer keep pace with increased utilization, and the blood glucose level begins to decline, eventually resulting in hypoglycemia [4].
Figure 23.1 The relative contributions of muscle glycogen breakdown and uptake of blood-borne glucose and non-esterified fatty acids (NEFA) to fuel utilization at various stages of prolonged low-intensity exercise in a healthy individual. The exercise intensity corresponds to 30% of maximal. Data from Ahlborg et al. [2].
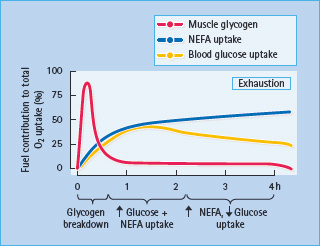
Effects of regular training on fuel metabolism
Aerobically trained athletes without diabetes have low fasting plasma insulin levels and reduced insulin responses to a glucose challenge in the face of normal glucose tolerance, and increased insulin-mediated glucose uptake under glucose clamp conditions [5]. Physical training is also known to increase muscle and hepatic insulin sensitivity in previously untrained individuals [6]. Lipid and lipoprotein profiles become less atherogenic with regular training: serum high density lipoprotein (HDL) cholesterol levels may increase, while total and low density lipoprotein (LDL) cholesterol levels may remain unchanged or decline [7,8]. Some studies have found that serum triglyceride levels decline after training [7,8]. Even when LDL cholesterol concentrations remain unchanged with aerobic exercise training there is an increase in mean LDL particle diameter, reflecting more buoyant and less atherogenic LDL cholesterol particles [9].
Anaerobic exercise
Effects of regular anaerobic exercise training
Regular anaerobic training improves the body’s ability to provide the fuels necessary for the production of anaerobic power. Adenosine triphosphate (ATP) and phosphocreatine levels (the main fuels for short powerful bursts) increase within muscle tissue, allowing the individual to sustain higher absolute exercise intensities for longer periods of time.
Fuel metabolism during anaerobic activity
With very intense exercise (more than 80% of – a level sustainable for no more than 15 minutes by most individuals), hepatic glucose production may exceed the rate of glucose utilization, leading to a 5- to 10-fold increase in blood glucose [10–12]. During recovery from very intense exercise, hyperglycemia often occurs in fit individuals without diabetes, as glucose utilization decreases more quickly than glucose production [10–12]. In response to the hyperglycemia, plasma insulin levels increase and glycemia is restored to baseline within about 45 minutes.
Resistance exercise
Effects of regular resistance exercise training
Declines in muscle strength of approximately 12–15% per decade have been reported after the age of 50 years [13,14], with muscle mass decreasing as much as 6% per decade [5,16]. With regular heavy resistance training, increases of greater than 30% in muscle strength [15,17] and gains in muscle mass ranging from 3 to 12% [18,19] have been found within the first couple of months of training, with relative increases typically being higher in elderly subjects [16,18]. Initial strength gains during the first 6 weeks are generally as a result of peripheral nervous system adaptations (improved muscle recruitment) and are not accompanied by muscle hypertrophy. Resistance training can be of additional benefit to older adults as improvements in postural stability and dynamic balance may decrease the risk of fall-related injuries [20].
When muscles are forced to perform for a given period of time at or near their maximal strength and endurance capacity (either by lifting weights or working against some other form of resistance), it will result in an increase in muscle strength, endurance and hypertrophy. Performing more repetitions (up to 2 minutes per set of each exercise) with lower resistance tends to increase endurance, while lifting heavier weights for fewer repetitions will favor strength gains [21].
Fuel metabolism during resistance exercise
High intensity, short duration resistance exercise is fueled by energy generated from the breakdown of stored intramuscular high-energy phosphates, ATP and phosphocreatine. During such exercise, adequate rest periods (2–5 minutes) between sets are necessary to allow regeneration of phosphocreatine stores. Glycolytic anaerobic energy production (with concomitant blood lactate accumulation) becomes more important as intensity decreases, the number of repetitions per set increases, and rest periods between sets become shorter [22]. This can result in local muscle lactate accumulation even after a single set. Where multiple sets are performed, longer sets and shorter rest periods between sets are associated with greater increases in blood lactate (which can reduce muscle contractility) and declines in muscle glycogen [23,24].
Metabolic and hormonal effects of exercise in diabetes
Type 1 diabetes
Higher amounts of habitual physical activity are associated with decreased incidence of diabetes-related complications and reduced mortality in individuals with type 1 diabetes mellitus (T1DM) [25]. In a prospective study, 548 patients with T1DM were followed for 7 years to ascertain the prevalence of complications and mortality [25]. The risk of microvascular complications varied inversely with self-reported activity levels at baseline. Sedentary male patients were three times more likely to die than the active ones after adjusting for age, body mass index, smoking and diabetic complications (Figure 23.2) [25]. A similar, although statistically non-significant, relationship was also seen in females.
Figure 23.2 Proportion of men who died during a 7-year follow-up period among 548 patients with type 1 diabetes, stratifi ed according to the physical activity quintile. Quintile cut-offs were < 398; 398–1000; 1000–2230; 2230–4228 and < 4228 kcal/week. The difference among groups is highly significant. Data from Moy et al.[25].
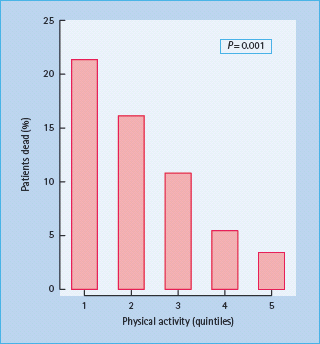
Studies have also shown an inverse association between physical activity and the severity of several complications in T1DM [26–29]. A longitudinal study of 1680 individuals with T1DM showed that self-reported leisure time physical activity levels were inversely correlated with measures of glycemic control and insulin sensitivity [29]. Women with diabetes in the study with low levels of leisure time physical activity tended to have poor glycemic control when compared with those with higher activity levels. Estimates of insulin sensitivity were also higher among more active men and women [29]. A follow-up study involving 1945 individuals with T1DM reported that those involved in either little leisure time physical activity or low intensity activity were more likely to have impaired renal function, a higher degree of proteinuria, as well as greater rates of retinopathy and cardiovascular disease when compared with more frequently and more vigorously active counterparts [28]. More recently, a randomized trial showed that the onset of diabetic peripheral neuropathy can be prevented and its progression delayed over a 4-year period by regular exercise [26].
General metabolic considerations
The intensity and duration of exercise, the patient’s level of blood glucose control, the type, dose and site of pre-exercise insulin injections, and the timing of the previous insulin injection and meal relative to the exercise can all affect the response of an individual with diabetes to physical activity (Figure 23.3). Accordingly, blood glucose concentrations can decline (the most common response in moderate aerobic exercise), increase (particularly in very intense exercise) or remain unchanged (Table 23.2). Whereas insulin secretion declines during moderate intensity aerobic exercise in individuals without diabetes, compensating for exercise-induced increased muscle insulin sensitivity, this physiologic decline in insulinemia cannot occur in T1DM because all insulin is exogenous. Insulin attenuates the appropriate rise in hepatic glucose production and further accelerates the exercise-induced stimulation of glucose uptake into the contracting muscle.
Table 23.2 Factors determining the glycemic response to acute exercise in people with type 1 diabetes.
Blood glucose tends to decrease if :
|
Figure 23.3 Plasma insulin levels may vary widely during exercise in patients with insulin-treated diabetes, whereas healthy subjects (as well as patients with type 2 diabetes) show a steady decline during moderate intensity aerobic exercise. Hyperinsulinemia and hypoglycemia may occur when the peak action of a short-acting insulin analog (1), conventional soluble (regular) insulin (2) or intermediate-acting insulin (3) occurs during exercise, or if exercise itself accelerates the absorption from the injection site (4). Steady-state but elevated plasma insulin concentrations may occur if intermediate or long-acting insulin has been injected a few hours before exercise, or if the patient is using an insulin pump (5). Declining levels (6) or hypoinsulinemia (7) occur when the previous injection depots are exhausted.
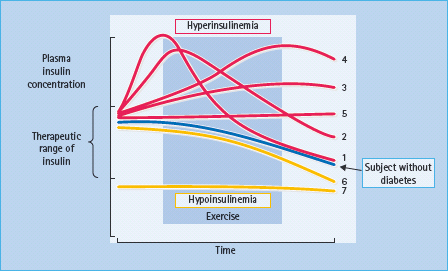
Hyperinsulinemia may occur for several reasons (Figure 23.4). Regular insulin injected a few hours previously may exert its peak action during exercise. This effect is exaggerated if the previously injected limb is exercised, as insulin absorption is accelerated by exercise [30]. Moreover, the use of intermediate or long-acting insulin generally produces higher peripheral insulin levels than would be found in an individual without diabetes of similar body composition. The result is often hypoglycemia, unless the insulin dose prior to exercise is decreased or extra carbohydrate is consumed. Hyperinsulinemia can also prevent the normal increase in lipid mobilization during exercise, leading to reduced availability of non-esterified fatty acids as a fuel.
Figure 23.4 Insulin concentrations during exercise depend on the type of insulin and the time interval between the injection and exercise. Serum insulin concentrations during early exercise are higher after injection of the lispro (Humalog) insulin analog (A) than after conventional soluble insulin (S), whereas the opposite situation applies during delayed exercise. Soluble insulin was injected 30-min and lispro insulin 5-min after breakfast, and exercise was started either 40-min (early) or 180-min (late) after breakfast. *P < 0.05. Data from Tuominen et al. [163].
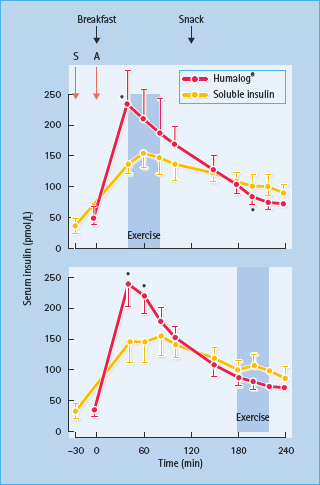
Conversely, if insulin levels are too low, the inhibitory effect of insulin on hepatic glucose production and its stimulatory effect on glucose uptake are both reduced. In addition, the counter-regulatory response (catecholamines, glucagon, growth hormone, cortisol) to exercise is higher than normal under conditions of insulin deficiency [30]. The overall result is markedly increased hepatic glucose production and diminished glucose utilization by the exercising muscle, thus leading to hyperglycemia.
Aerobic exercise in type 1 diabetes
Effects of regular aerobic exercise training in type 1 diabetes
Insulin sensitivity is reduced to varying degrees in patients who have had T1DM for several years [31]. Participation in a regular exercise training program improves whole-body insulin sensitivity in these patients, as it does in those without diabetes, which may help to induce and prolong remission in newly presenting T1DM [32]. It has been difficult to ascertain whether or not regular aerobic exercise improves glycemic control in T1DM, as outcomes of exercise intervention have been inconsistent. While some studies have demonstrated improvements, albeit not statistically significant, in blood glucose control as measured by decreases in HbA1c [33,34]. most studies have either not shown any changes [35] or have produced increases in HbA1c [36]. The main reason for this is probably excessive reductions of insulin dose or disproportionate carbohydrate consumption before exercise in an effort to avoid hypoglycemia. It is important to note that participants in several studies still experienced many exercise-related benefits including lower body fat percentage [34]. improved muscle mass [37], increased aerobic capacity [34,36,37], improved lipid profiles [34,36,37] and greater insulin sensitivity (with a consequent lower need for exogenous insulin) [36].
Fuel metabolism during aerobic activity
Under ideal conditions of low insulin and euglycemia, fuel selection during mild to moderate exercise can be very similar in people with T1DM as those without diabetes, with a gradual shift towards lipid oxidation with increasing exercise duration [38]. Conversely, if the levels of circulating insulin are too high, muscle, adipose and hepatic cells will continue to take up glucose for storage rather than leaving it available for working muscles. While glucagon can still be produced in individuals with T1DM [39], the glucagon: insulin molar ratio at the portal vein may not reach the level necessary for adequate levels of hepatic glucose production to occur. Compared with individuals without diabetes, those with T1DM may demonstrate a lower reliance on hepatic glycogen stores during low intensity aerobic activity [40]. If the rate of glucose appearance in the system is not high enough to supply the demand, blood glucose levels will drop, thereby increasing the potential for hypoglycemia [39].
Levels of insulinemia and glycemia will also affect the fuels used during aerobic activity. Hyperinsulinemia can lead to a greater reliance on exogenous glucose utilization during moderate aerobic exercise, without sparing glycogen stores [41]. In a study where insulinemia was kept constant, the contribution of carbohydrates to overall energy metabolism during exercise was greater in hyperglycemia than in euglycemia [38]. The shift towards greater carbohydrate metabolism during exercise in hyperglycemia was accompanied by a blunted cortisol and growth hormone response. Insulin, glucagon and catecholamine levels were comparable between conditions [38]. Overall, individuals with T1DM seem to have a greater reliance on muscle glycogenolysis and gluconeogenesis to produce fuel during physical activity than their non-diabetic counterparts [40,42].
Despite the complexities of glucoregulation, well-motivated people with T1DM can successfully undertake several hours of exhaustive aerobic exercise if appropriate insulin and dietary adjustments are made [43–45]. After prolonged exercise, patients may be prone to hypoglycemia for many hours, even extending overnight and to the following day. This can be explained by persistently enhanced glucose uptake by the exercised muscles to replenish the depleted glycogen stores [43]; however, after certain types of prolonged exercise, such as a marathon run, increased lipid oxidation can persist, as occurs in healthy individuals [44]. In this case, the use of glucose as a fuel and insulin sensitivity are reduced after exercise, thereby decreasing the risk of post-exercise hypoglycemia [44]. It should also be noted that individuals who experience frequent bouts of hypoglycemia (whether exercise-related or not), may have further blunting of counter-regulatory responses to exercise, potentially creating a vicious cycle of hypoglycemic events [46–50].
Effects of regular anaerobic exercise training in type 1 diabetes
There is currently a gap in the literature as to how physical and metabolic adaptations to regular anaerobic training in individuals with T1DM differ from those of non-diabetic individuals. People with T1DM who participate in competitive sports may find that their glycemic control worsens, probably secondary to an irregular schedule of very vigorous exercise, insulin dose reduction and high carbohydrate consumption [51]. In spite of the elevation in physical fitness and , these individuals may not have increased insulin sensitivity, but instead show enhanced use of non-esterified fatty acids as a fuel [51]. It is likely that many individuals with T1DM will have to compromise some tightness of control to be able to participate effectively in competitive sports and high intensity activities, to avoid the fear of hypogly-cemia during exercise. Whether or not this affects their physical and metabolic adaptations to these types of activities requires further research. More details on carbohydrate and insulin adjustments for exercise can be found later in this chapter.
Fuel selection during high intensity exercise
Extremely strenuous acute exercise may cause hyperglycemia even in the presence of normal or high insulin levels. High counter-regulatory hormone action (especially catecholamines) can stimulate glucose production beyond the limits of peripheral utilization [52–56]. In addition, in T1DM the normal physiologic doubling of insulin concentration after cessation of such intense exercise cannot occur, resulting in more prolonged hyperglycemia [52,55,56]. Augmented lipid mobilization and ketogenesis in the liver increase blood ketone-body concentrations; the hypoinsulinemic individuals may thus become hyperketonemic and ketonuric after exercise.
Several recent studies have shown that such high intensity exercise is effective in increasing blood glucose levels and thereby delaying or preventing hypoglycemia related to moderate exercise even when performed in extremely short bouts [57–61]. Bussau et al. [57,58] showed that a 10-second sprint performed either prior to [58] or at the completion of [57] 20 minutes of low intensity cycling significantly attenuated post-exercise drops in blood glucose, when compared with exercise sessions where no sprint was performed. Similar results were found by Guelfi et al. [59,60] where post-exercise blood glucose levels remained higher after cycling exercise sessions where 4-second sprints were performed every 2 minutes versus those where the participants cycled continuously at constant moderate intensity (Figure 23.5). The post-exercise window in these studies, however, was limited to 120 minutes. One small study using continuous glucose monitoring systems (CGMS) found a trend towards late onset post-exercise hypoglycemia after high intensity activity [62]; however, further studies with larger sample sizes will be necessary to confirm this.
Figure 23.5 Effect of 30 min of intermittent high intensity exercise (IHE) or moderate continuous exercise (MOD) on normalized blood glucose levels. Data from Guelfi et al. [59].
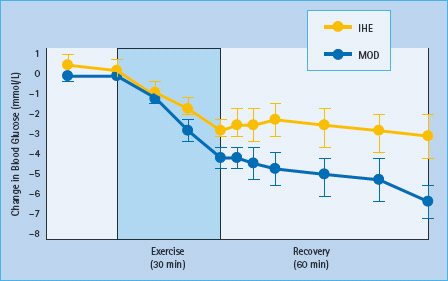
Resistance exercise
Effects of regular resistance exercise training in type 1 diabetes
There is a paucity of information regarding the outcomes of training programs consisting of only resistance exercise in individuals with T1DM. The one study completed to date involved a cross-over design with 10 weeks of heavy resistance exercise three times a week followed by a 6-week period with no resistance training, or vice versa [63]. Mean HbA1c was 6.9 ± 1.4% (52 ± 15mmol/mol) at the end of the non-resistance exercise period and 5.8 ± 0.9% (40 ± 10mmol/mol) at the end of the 10-week resistance exercise training period. Serum cholesterol and self-monitored glucose levels were also lower at the end of the resistance training period.
One study has compared the effects of 12 weeks of resistance training with those of a comparable period of aerobic training in individuals with T1DM [64]. While both groups showed decreases in waist circumference, reduced insulin dosage and lower self-monitored blood glucose after the training period, these variables only reached significance in the aerobically trained group. However, HbA.c levels showed a statistically significant increase in the aerobic training group (from 8.7 ± 1.6 to 9.8 ± 1.8%; 72–84mmol/mol), while a non-significant decrease (from 8.2 ± 2.9 to 7.6 ± 1.6%; 60–66 mmol/mol) was found in the resistance training group.
A handful of pre–post studies have also examined the effects of combined aerobic and resistance training programs in individuals with T1DM [65–67]. Outcomes of these studies were generally positive, with benefits including lower HbA1c [65,67], decreased blood pressure [65,66], improved nerve conduction [65], higher cardiorespiratory fitness [67], greater muscular strength [67], higher lean body mass [65,67] and improvements in lipid profiles [67]. Nevertheless, it should be noted that the interpretation of these studies is limited by their lack of concurrent diabetic control groups. All studies involving resistance exercise interventions in individuals with T1DM (alone or in combination with aerobic exercise) published by March 1, 2009 are outlined in Table 23.3.
Table 23.3 Clinical trials involving resistance exercise with people with type 1 diabetes mellitus.
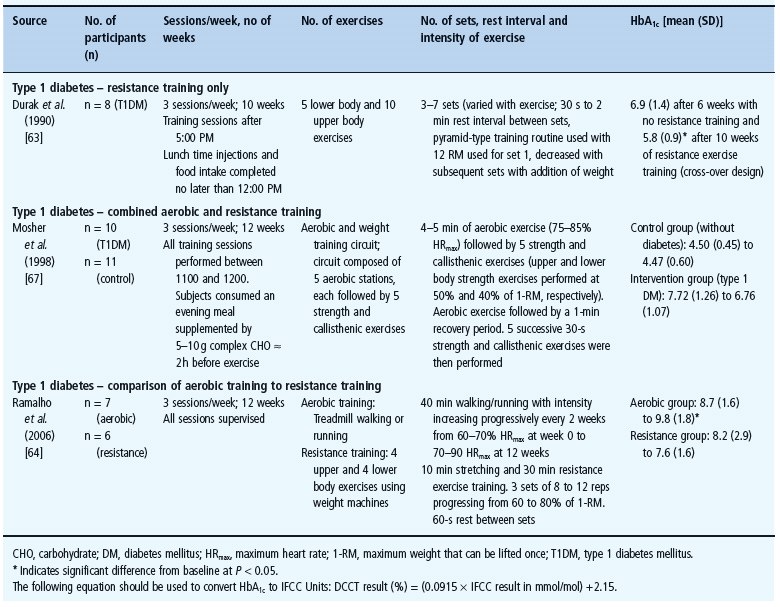
Fuel selection during resistance exercise
Very little is known about the acute hormonal and resulting blood glucose responses to resistance exercise in individuals with T1DM. Resistance exercise in individuals without diabetes produces elevated epinephrine levels, which enhance hepatic glucose production, resulting in an increase in blood glucose levels [68]. As studies involving high intensity exercise demonstrate that catecholamine responses are similar in young fit individuals with and without T1DM. 55,57,59]. it is likely that resistance-type activities are associated with increases in blood glucose during and after exercise. However, the authors are unaware of any published research examining the glycemic response during and after this type of exercise in people with T1DM.
Type 2 diabetes
Exercise and type 2 diabetes prevention
In large prospective cohort studies, higher levels of physical activity and/or cardiorespiratory fitness have consistently been associated with reduced risk of developing T2DM [69–81]. After adjustment for confounding variables, participants who exercised the most had a 25–60% lower risk of subsequent diabetes compared to those who were most sedentary. This was true regardless of the presence or absence of additional risk factors for diabetes such as hypertension, parental history of diabetes and obesity in most studies. Comparable magnitudes of risk reduction are seen with walking compared to more vigorous activity when total energy expenditures are similar [77].
Several clinical trials have demonstrated that supervised structured physical activity programs, with or without concomitant dietary interventions, reduce the risk of developing T2DM in individuals with impaired glucose tolerance (IGT) [82–85]. In the non-randomized Malmö trial [82,86,87], 260 men aged 47–49 years with IGT were identified through population screening and were offered a 6–12-month intervention including supervised exercise programs and dietary counseling. Among those attending a 5-year follow-up examination, 21% of the control participants (those who had declined the intervention) had developed diabetes, compared to only 11% of those receiving the intervention. Over 12 years, mortality among the controls was 14.0 per 1000 person-years, but only 6.5 per 1000 person-years in the intervention group [87].
In the Da Qing IGT and Diabetes Study [83], 577 people with IGT from 33 clinics were cluster-randomized by clinic to diet only, exercise only, diet and exercise or control, and followed for 6 years. The cumulative incidence of T2DM was 68% in controls, but only 44%, 41% and 46% in the diet, exercise, and diet and exercise groups, respectively. Li et al. [88] recently published a follow-up paper to the Da Qing Study [83] assessing the long-term effect of the 6-year intervention on the risk of diabetes. They found that, 14 years after the end of the trial, incidence of diabetes remained significantly lower in those originally randomized to one of the intervention groups versus controls.
In the Finnish Diabetes Prevention Study [89], 523 Finnish adults with IGT were randomized to an exercise and diet intervention or control. Patients in the control group had one meeting per year with a physician and dietitian, at which they received standard advice on diet and exercise. The intervention group received individualized exercise plans, thrice-weekly supervised facility-based aerobic and resistance exercise, and seven 1-hour meetings with a dietitian focusing on weight reduction, reduced fat intake and reduced total caloric intake. At 4 years, 22% of the control group and only 10% of the intervention group had developed diabetes – a 58% risk reduction. Three years after the end of the study, participants originally randomized to the intervention group continued to have much higher levels of physical activity than those originally in the control group [90]. Incidence of diabetes in the individuals who were previously in the intervention group remained 43% lower than in those who were previously in the control group [90].
In the US-based Diabetes Prevention Program [85], 3234 American men and women with IGT were randomly assigned to placebo, metformin or a lifestyle modification program. The goals of the lifestyle modification program included a 7% weight loss and at least 150 minutes of exercise per week. The lifestyle intervention included 16 lessons in the first 24 weeks, covering diet, exercise and behavior modification (delivered one-on-one by a case manager). A minimum of two supervised exercise sessions per week and at least monthly contact with the study personnel were maintained thereafter. The study was ended after a mean of 2.8 years of follow-up, 1 year earlier than originally planned, because of clear-cut superiority of the lifestyle arm. Cumulative incidences of T2DM were 11.0 per 100 person-years in the placebo group, 7.8 per 100 person-years in the metformin group and only 4.8 per 100 person-years in the intensive lifestyle group. The risk of T2DM was 58% lower in the lifestyle group than in the placebo group, and 39% lower than in the metformin group. An epidemiologic analysis of the data by Hamman et al. determined that, after adjusting for changes in self-reported diet and physical activity, there was a 16% reduction in diabetes risk for every kilogram of weight loss among the lifestyle intervention participants [169]. Increased physical activity was a significant predictor of weight loss and had an important role in weight maintenance. In addition, participants who met the goal of more than 150 minutes of moderate exercise per week had a 46% reduction in diabetes risk.
Aerobic exercise in type 2 diabetes
Regular exercise is associated with reduced morbidity and mortality in T2DM. A 10-year follow-up of a large prospective cohort study including 347 individuals with diabetes and 1317 individuals without diabetes) found that the lowest aged- adjusted all-cause death rate was among those with diabetes who walked a mile or more daily [91]. Similarly, a prospective study of 1263 men with diabetes followed over 12 years found that, compared with the least fit men (bottom 20% as determined by maximal treadmill testing), those with moderate cardiorespiratory fitness had a 60% lower risk of cardiovascular and overall mortality [79]. The effect of fitness on mortality was considerably greater than the effect of body mass index. Further follow-up of this cohort confirmed these findings [92,93]. Greater habitual exercise was also associated with a lower subsequent risk of cardiovascular disease among women in the Nurses Health Study [94].
Effects of regular aerobic exercise training in type 2 diabetes
Exercise-induced stimulation of glucose uptake may involve many factors (Figure 23.6), as reviewed by Ivy et al. [95]. They include increased post-receptor insulin signaling [96]. increased glucose transporter protein and mRNA [97], increased activity of glycogen synthase [98] and hexokinase [99], decreased release and increased clearance of free fatty acids [95], increased muscle glucose delivery as a result of increased muscle capillary density [99–101], changes in muscle composition favoring increased glucose disposal [99,102,103] and changes in adipose tissue mass and distribution [104]. Decreases in visceral fat result in decreased concentrations of tumor necrosis factor a [105] and free fatty acids [106], leading to decreased insulin resistance.
Figure 23.6 Mechanisms by which exercise training may improve insulin action and the control of blood glucose in type 2 diabetes. FFA, free fatty acid; GLUT, glucose transporter; HGO, hepatic glucose output; Rd, glucose uptake; TNF-α, tumour necrosis factor-α. Reproduced from Ivy et al. [95], with permission from Lippincott Williams & Wilkins.
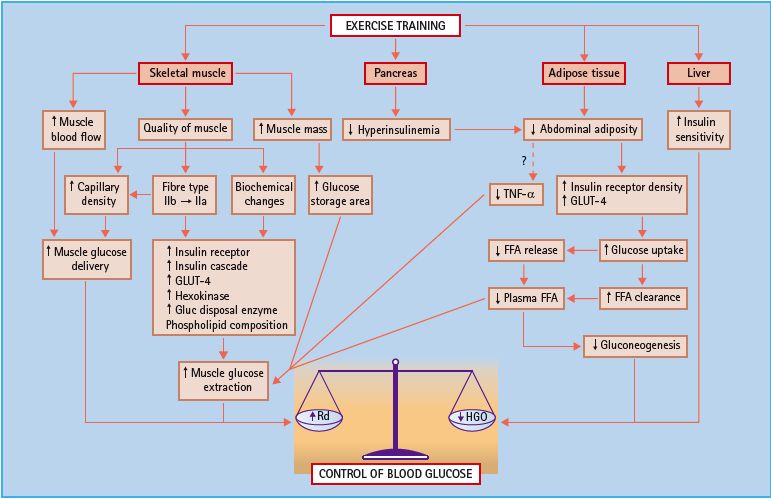
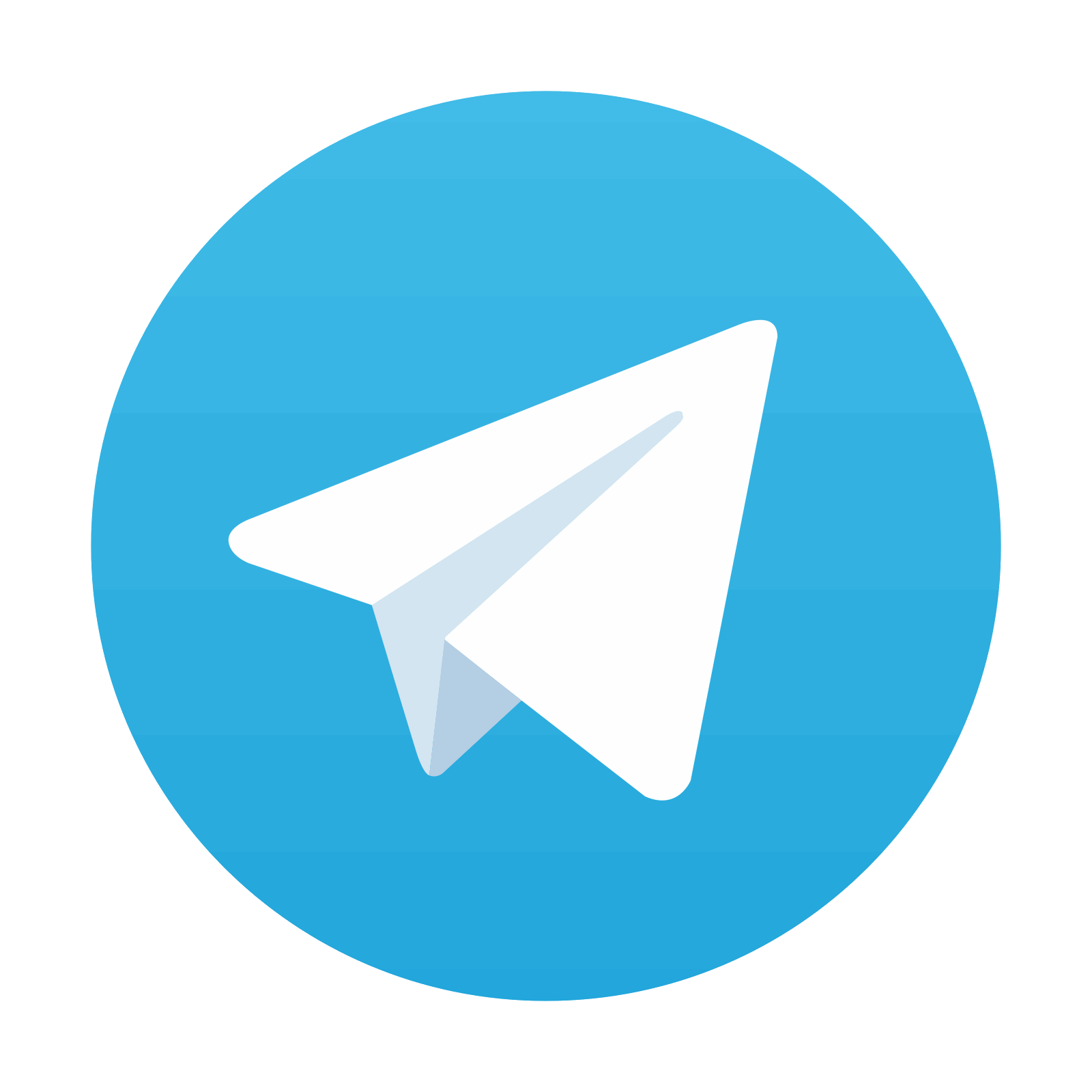
Stay updated, free articles. Join our Telegram channel

Full access? Get Clinical Tree
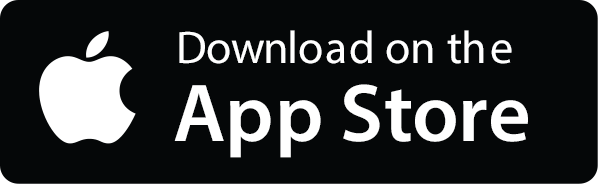
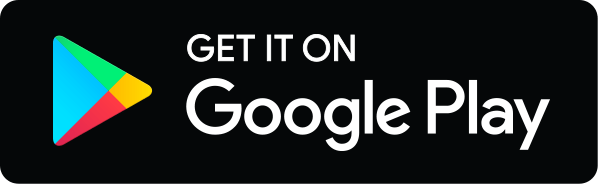