BOTULISM
JAMES D. MARKS
Botulism is a rare but life-threatening disease caused by spore-forming bacteria of the Clostridium genus, including Clostridium botulinum, Clostridium baratii, and Clostridium butyricum (1). The disease results from bacterial secretion of botulinum neurotoxin (BoNT), the most poisonous substance known (2). Approximately 7 pg of pure neurotoxin is the median lethal dose (lethal to 50% of the test group) (LD50) for a mouse, and it has been estimated that the human LD50 is approximately 0.09 to 0.15 µg intravenously, 0.7 to 0.9 µg inhalationally, and 70 µg orally (3–6).
Botulism is characterized by prolonged paralysis, which if not immediately fatal requires prolonged hospitalization in an intensive care unit and mechanical ventilation. The potent paralytic abilities of the neurotoxin have also resulted in its development as a biowarfare and biothreat agent (7), as well as a medicine to treat a range of overactive muscle conditions including cervical dystonias, cerebral palsy, posttraumatic brain injury, and poststroke spasticity (8). The toxin is also used cosmetically, for example, to treat wrinkles (9).
Clostridial organisms produce eight neurotoxins that differ significantly from each other in their amino acid sequences, resulting in the elicitation of different antibody responses. The different antibody responses allow the neurotoxins to be classified into different serotypes; antibodies that recognize one serotype do not recognize other serotypes. Of eight neurotoxin serotypes (A, B, C, D, E, F, G, and H) (10–12), five (A, B, E, F, and H) cause naturally occurring human botulism (7,12).
Naturally occurring botulism can result from ingestion of preformed toxin (food botulism) or from toxin produced in situ due to wound infection (wound botulism) or colonization of the gastrointestinal tract (infant or intestinal botulism). Botulism can also occur in exposed laboratory workers or from an overdose of therapeutic neurotoxin. In addition, the BoNTs are classified by the Centers for Disease Control and Prevention (CDC) as one of the six highest risk threat agents for bioterrorism, because of their extreme potency and lethality, ease of production and transport, and need for prolonged intensive care (7). Intoxication can occur via oral ingestion of toxin or inhalation of aerosolized toxin (13,14). Although only five of the neurotoxin serotypes cause natural human disease, aerosolized neurotoxin serotypes C, D, and G produce botulism in primates by the inhalation route (13) and would most likely also affect humans. Thus, any one of the eight neurotoxin serotypes can be used as a biothreat agent. Because of the severity of illness and the potential for outbreaks, both food-borne and biothreat botulism are public health emergencies.
ETIOLOGY
History and Types of Botulism
Each type of human botulism (food-borne, wound, infant, intestinal, inadvertent, or bioterror) is associated with different epidemiology and pathogenetic mechanisms. The name of the disease is derived from the Latin botulus (sausage), a food responsible for many early outbreaks of botulism. “Sausage poisoning” was recognized in Europe as early at the eighteenth century (15), with van Ermengem clearly describing the bacteriologic and toxicologic basis of the disease in 1897 in an outbreak in Belgium resulting from inadequately cured ham (16). The first recognized case of botulism in the United States occurred in 1899 and was caused by a beef tamale (15). Food botulism was the most common form of botulism in the United States prior to 1980.
Infant (or intestinal) botulism was first described in 1976 by two groups (17,18) and is now the most frequently reported type of botulism in the United States (http://www.cdc.gov/nationalsurveillance/PDFs/Botulism_CSTE_2011.pdf). Wound botulism was first described in the United States in 1951, with initial cases primarily due to traumatic wounds of the extremities (19). More recently, the incidence of this form of botulism has increased and has been associated with injection drug users injecting black tar heroin (20). An adult variant of infant botulism, varyingly called botulinal autointoxication, hidden, adult intestinal, or adult infectious botulism, was first described in 1979 (21,22). Inadvertent botulism results from unintentional exposure and typically occurs in laboratory workers (23) and in patients receiving therapeutic BoNT (24). Although successful use of neurotoxin as a bioterror agent has not occurred, the Japanese cult Aum Shinryko unsuccessfully released BoNT on at least three occasions (7).
Clostridial Bacteriology
C. botulinum can be classified into at least four genetically and phenotypically diverse groups (I through IV) (25,26). Although these groups are different enough to be classified as separate species, they have all been classified as C. botulinum because they share the common feature of neurotoxin production. The organisms in group I are referred to as proteolytic and the organisms in group II as nonproteolytic, based on their ability to digest complex proteins. All serotype A strains are group I, serotype B and F can be produced by either group, and serotype E is produced by group II strains. Serotypes C and D are both produced by group III organisms. Type C is found in avian species, occurring in domestic flocks and massive outbreaks in wild waterfowl (27–29). Type C also occurs in other animals such as dogs, mink, and cattle. Type D outbreaks are rare and associated with cattle (30). A single human outbreak of type C and type D food botulism have been reported (31,32). Group IV was created to accommodate an organism isolated from a soil sample in Argentina that produces a unique neurotoxin (type G) that causes a flaccid paralysis in mice (33,34). No human cases of type G botulism have been reported, although it has been isolated from autopsy specimens (35). Recently, the name Clostridium argentinense has been proposed for group IV clostridia (36). Two additional clostridial species, C. butyricum and C. baratii produce neurotoxins E (37,38) and F (39,40), respectively. Finally, rare stains of clostridia have been reported that cause human clinical disease and secrete more than one toxin, for example, A and B (Ab), A and F (Af), B and F (Bf), B and A (Ba), and B and H (Bh) (12,26,41–44).
Neurotoxin Structure and Function
The protein neurotoxin is secreted as a single polypeptide chain of approximately 150 kDa, which is nicked by proteases to form a 100-kDa heavy chain and a 50-kDa light chain connected by a single disulfide bond. The sequences of the genes encoding neurotoxin serotypes A (45–47), B (48,49), C (50), D (51), E (52), F (53,54), and G (55) have been determined. Although these toxins differ by as much as 65% at the amino acid level (Table 36.1), it is likely that they share the same general protein structure (11). Significant sequence variability has also been observed within toxin serotypes, the so-called subserotypes (45,47–49,56–58). The most diverse subserotypes are those for serotype A (up to 16% different at the amino acid level) and serotype F (up to 36% different at the amino acid level) (57,58). Such changes account for the reported differences in the ability of monoclonal and polyclonal antibodies to bind and neutralize BoNTs from different strains (56,59–62).
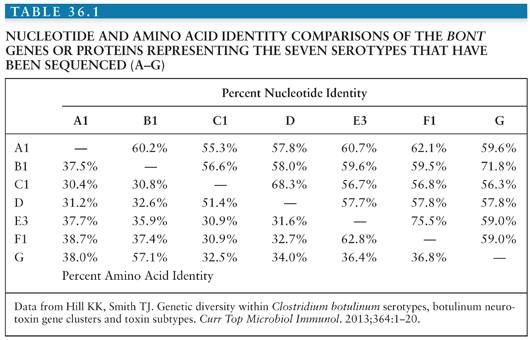
The x-ray crystal structures of types A, B, and E neurotoxins have been solved at high resolution (Fig. 36.1) (63–66). The structural studies, combined with functional studies, provide clear insight into how the BoNTs interfere with normal release of the neurotransmitter acetylcholine resulting in flaccid paralysis (Fig. 36.2). The C-terminal portion of the heavy chain (HC) comprises the receptor binding domain, which binds to cellular receptors on presynaptic neurons, resulting in toxin endocytosis (67,68) (Figs. 36.2A and B). Specific cellular receptors have been identified for the BoNTs, with cellular binding and entry requiring two co-receptors, a protein and a sialoganglioside such as GD1b or GT1b (68–71) (Fig. 36.3). The binding domain consists structurally of an N-terminal subdomain (HCN) consisting of a jelly roll motif and a C-terminal subdomain (HCC) consisting of a β-trefoil motif. The HCC of the binding domain comprises the protein receptor-binding site as well as the ganglioside-binding site (63) (Fig. 36.3). In BoNT/A, B, E, F, and G, ganglioside binding occurs in a conserved binding pocket in the HCC (Fig. 36.3) (65,72,73). BoNT/C and BoNT/D have two ganglioside-binding sites, one of which is located in the conserved binding pocket used by the other BoNTs. After ganglioside binding, a second binding event occurs to one of several synaptosomal proteins. BoNT/A, BoNT/D, BoNT/E, and BoNT/F bind synaptic vesicle 2 (SV2), whereas BoNT/B and BoNT/G bind synaptotagmin-1 and -2 respectively (64,74–79).
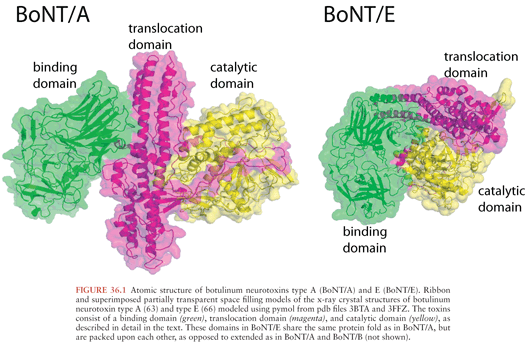
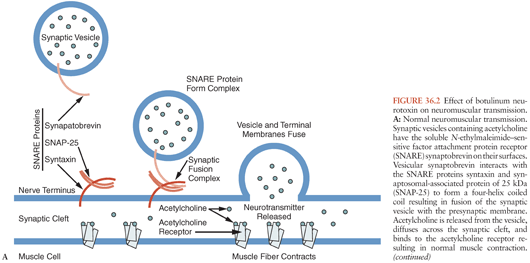
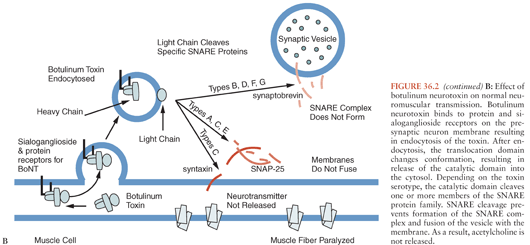
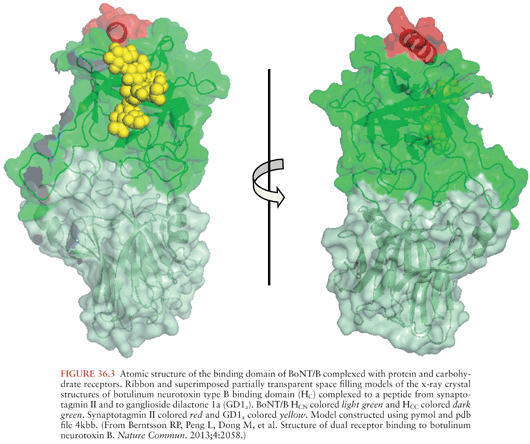
The N-terminal portion of the heavy chain (HN) (Fig. 36.1) comprises the translocation domain, which consists of alpha helices and is involved in pore formation. It is hypothesized that the lower pH level of the endosome induces a conformational change in this domain, which creates a pore allowing the light chain to escape the endosome (Fig. 36.2). The light chain (Fig. 36.1) is a zinc endopeptidase that, depending on serotype, cleaves different members of the soluble N-ethylmaleimide–sensitive factor attachment protein receptor (SNARE) family of proteins, resulting in blockade of neuromuscular transmission (80,81) (Fig. 36.2). The SNAREs are essential for normal fusion of the synaptic vesicle and acetylcholine release (Fig. 36.2). Toxin serotypes A and E cleave distinct sites within SNAP-25 (synaptosomal-associated protein of 25 kDa) (81–84), serotypes B, D, F, and G cleave distinct sites within vesicle-associated membrane protein (VAMP, also known as synaptobrevin) (80,81,85–88) and serotype C cleaves syntaxin and SNAP-25 (Fig. 36.2B) (89,90). These three SNARE proteins (syntaxin, SNAP-25, and synaptobrevin) interact to form a four-helix coiled coil in a step that precedes synaptic fusion (91) (Fig. 36.2). Cleavage of any one of these proteins blocks fusion and acetylcholine release leading to a flaccid paralysis.
BoNTs are secreted from clostridial species as progenitor toxin complexes (PTCs) ranging in size up to 900 kDa (92,93). These complexes consist of the neurotoxin and a number of proteins collectively called neurotoxin-associated proteins (NAPs). The NAPs include three proteins classified as hemagglutinins (HA70, HA17, and HA33) (94,95), because of their ability to agglutinate red blood cells, and other proteins termed nontoxin nonhemagglutinins (NTNH) (53,96). Recently, the x-ray crystal structure of NTNH complexed to BoNT/A was solved showing that NTNH has the same general structure as BoNT/A, packs tightly against BoNT/A in a manner like a “handshake,” and protects it from low pH denaturation and proteolysis in the gastrointestinal (GI) tract (Fig. 36.4) (97–99). The structure of a 760-kDa PTC has recently been solved showing a bimodular structure that the authors liken to an Apollo lunar module (Fig. 36.4). The “ascent stage” is a 280-kDa complex of BoNT/A and NTNH that protects BoNT from GI destruction, and the “descent stage” is a 470-kDa module composed of HA17, HA33, and HA70 that mediates absorption of BoNT by binding to host carbohydrate receptors in the GI tract (100,101).
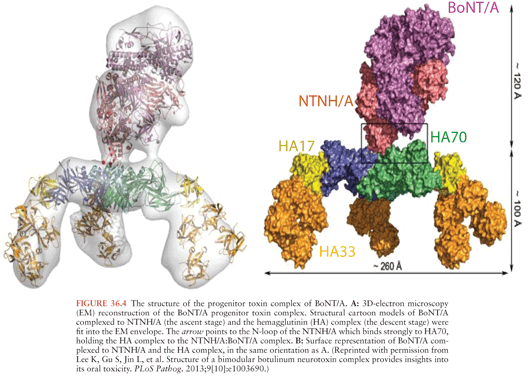
DIFFERENTIAL DIAGNOSIS
Botulism is underdiagnosed and often misdiagnosed, often as Guillain-Barré or Miller-Fisher syndrome, myasthenia gravis, poliomyelitis, intoxications, or disease of the central nervous system (CNS) (Table 36.2) (7,102). Common and uncommon misdiagnoses are listed in Table 36.2, along with features that distinguish botulism from these diseases. Common signs and symptoms of botulism are listed in Table 36.3 and discussed further in the section on clinical diagnosis. Botulism is much more likely to be associated with outbreaks (cluster of cases) than other diseases with which it may be confused. This fact emphasizes the importance of prompt reporting of suspected botulism cases to the public health department. Botulism differs from other flaccid paralyses in (a) its prominent cranial nerve involvement disproportionate to weakness below the neck, (b) the symmetry of the weakness, and (c) the absence of sensory changes, although approximately 14% of patients report paresthesias (Table 36.3).
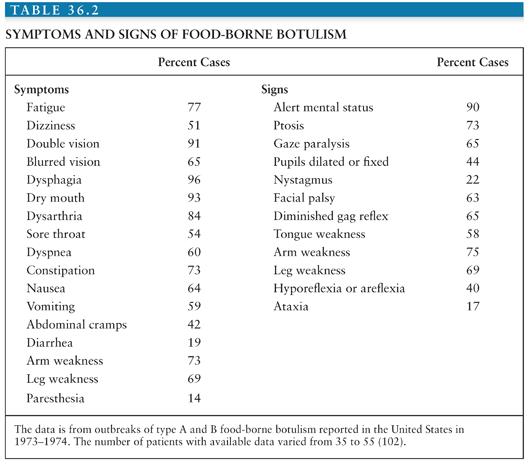
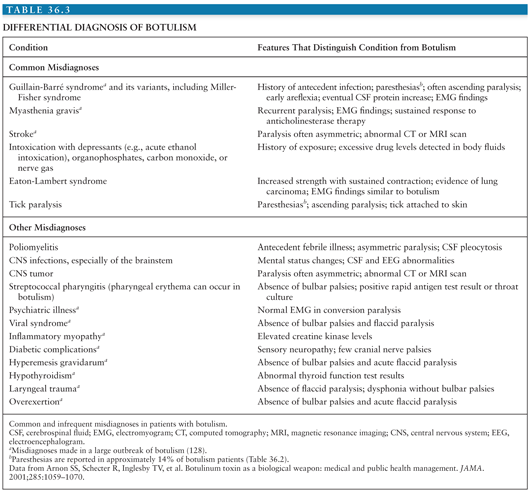
EPIDEMIOLOGY
In the most recent report from 2011 of the types of U.S. botulism cases, the CDC documented a total of 140 confirmed cases of botulism; 73% of cases were infant botulism, 20% food-borne, 13% wound, and 4% of unknown etiology (http://www.cdc.gov/nationalsurveillance/PDFs/Botulism_CSTE_2011.pdf).
Food-Borne Botulism
Food-borne botulism is usually associated with ingestion of preformed toxin in home canned products, most frequently foods low in acid such as vegetables, fish or marine mammals, condiments, and meat products. Fruits are rarely involved, because of their high natural acidity. Outbreaks have also been reported from commercially prepared products (103,104) and from food prepared in restaurants (105,106). In the United States, the incidence of food-borne botulism is highest in Alaska, where the vehicle is typically native Alaskan foods consisting of fermented or salted fish or marine mammal products (107). In other countries, different types of foods predominate (108,109). In Germany, Italy, France, and Poland, meats such as home-cured hams are the most frequently implicated foods. In Canada, Japan, and Scandinavia, outbreaks are usually associated with fish products.
Food-borne botulism usually occurs in outbreaks where multiple individuals ingest contaminated food. From 1899 to 1996, 921 outbreaks of food-borne botulism were reported to the CDC, with a relatively constant incidence of approximately 9.5 outbreaks per year, with an average of 2.5 cases per outbreak (1). The largest number of cases in a single food-borne outbreak was 59. From 1899 to 1996, 2,368 cases of food-borne botulism were reported; of these, 1,281 were reported between 1899 and 1949, and 1,087 were reported from 1950 to 1996. Between 1899 and 1949, the case-fatality ratio was approximately 60%. Since 1950, the mortality rate has decreased significantly to approximately 5.7% between 1990 and 1996. This decrease is attributed to improvements in respiratory intensive care and early administration of antitoxin.
Food-borne botulism is typically caused by type A, B, and E neurotoxins. Of the 1,087 cases of food-borne botulism reported between 1950 and 1996, the toxin type could be determined for 786; of these, 52% were type A, 22% were type B, 25% were type E, and less than 1% were type F (1). Type A botulism is most common west of the Mississippi River, type B is most common east of the Mississippi, and type E botulism predominates in Alaska (1,110). This distribution corresponds to the distribution of C. botulinum spores in the soil (111–114). Three outbreaks of type F botulism have been reported in the United States, with one of these due to home-prepared venison jerky (1). In 2011, 70% of the 20 reported food-borne botulism cases were of type A, 25% type E, and 5% type F (http://www.cdc.gov/nationalsurveillance/PDFs/Botulism_CSTE_2011.pdf).
Improvements in food-processing techniques have significantly reduced, but not eliminated, food-borne outbreaks from commercially prepared foods. Those that do occur are associated with inadequate pasteurization or breaks in the refrigeration chain (115). Outbreaks from home canning still occur and can best be prevented by adhering to the recommended canning techniques. C. botulinum spores are not killed by heating at 100°C, so pressure cooking is essential. BoNT, unlike spores, is heat labile and can be destroyed by heating to 80°C (176°F). Thus, thoroughly heating home-canned foods before eating them can reduce the risk of botulism.
Infant Botulism
Infant botulism was first reported in 1976 (17,18) but likely existed as a clinical entity before this date (116). This form of botulism is now the most frequent type of botulism in the United States. Infant botulism is distinct from food-borne botulism, being caused by the colonization (infection) of the intestines with clostridial strains and the subsequent in situ production of toxin, rather than the ingestion of preformed toxin. Between 1976 and 1996, 1,442 cases were reported to the CDC (1). The mean age at onset was 13 weeks, with a range of 1 to 63 weeks, and males and females were equally affected. The incidence of infant botulism has been essentially stable since 1980, with an average annual incidence of approximately 1.9 per 100,000 live births. Since 1976, the highest incidence of infant botulism has been in Delaware, Hawaii, Utah, and California, with almost half of reported cases from California. Reasons for the geographic variation are unknown.
Infant botulism has been reported from at least 14 countries worldwide including Argentina, Australia, Japan, Canada, Italy, the United Kingdom, Chile, the former Czechoslovakia, France, Spain, Switzerland, Sweden, and Taiwan (117; S.S. Arnon, personal communication, May, 2013). In the United States in 2011, 40% of the 102 reported infant botulism cases were type A, 60% were type B, and one case resulted from infection with a bivalent Ba strain. There have been rare reports of infant botulism caused by other serotypes and strains, including one case in Japan caused by type C toxin (118), multiple cases in the United States caused by C. baratii–producing type F toxin (26,39), and multiple cases in Italy caused by C. butyricum–producing type E toxin (37,38). Cases have also occurred due to production of multiple toxins; infant botulism cases have been reported from the United States and the United Kingdom where both types B and F are produced (Bf) (26,42) and from the United States where types B and A are produced (Ba) (41,43).
The primary source for clostridial spores is the environment. Clustering of some cases of infant botulism has been reported in the eastern United States and in small towns and rural areas of the western United States (119–122). In approximately 20% of cases, there was a history of honey ingestion prior to the development of botulism, and spores of the same type could be cultured from the honey (119,123,124). Infants with botulism are more likely to have been breast-fed (124–127), which in experimental models can alter the fecal flora differently than formula feeding and increase susceptibility to C. botulinum colonization (128).
A tentative link exists between infant botulism and some cases of sudden infant death syndrome (SIDS). C. botulinum spores could be found in 5% of 211 necropsy specimens from SIDS cases in California, with toxin detected in 2 of 10 culture-positive cases (129,130). Toxin or clostridial organisms have been reported in a number of SIDS cases from Switzerland (131), Italy (132), and Germany (133), but not from Australia (134). Thus, infant botulism may account for a small percentage of SIDS cases, perhaps especially in North America and Europe.
Wound Botulism
Wound botulism, first described in 1951, is a rare disease caused by the growth of C. botulinum in contaminated wounds with in situ toxin production. Through 1985, 33 cases of wound botulism were reported in the United States; 25 of these cases were laboratory confirmed, with 17 cases type A and 7 cases type B (1). One case was a mixture of type A and B. Eighty-one percent of the cases occurred in males with a mean age of 21 years. Wounds were typically deep with necrotic areas and associated compound fractures. The median incubation period in trauma cases was 7 days, with a range of 4 to 21 days (135
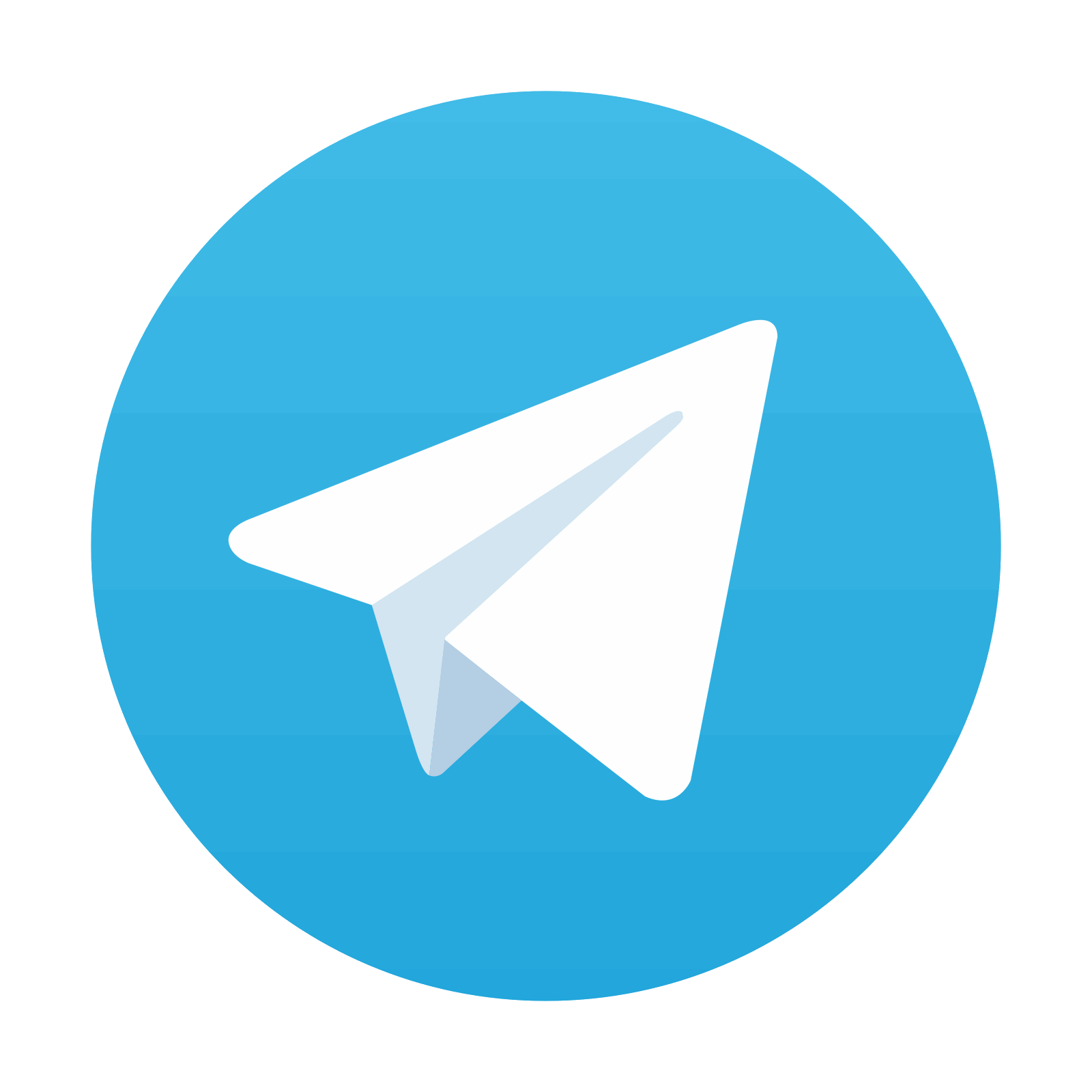
Stay updated, free articles. Join our Telegram channel

Full access? Get Clinical Tree
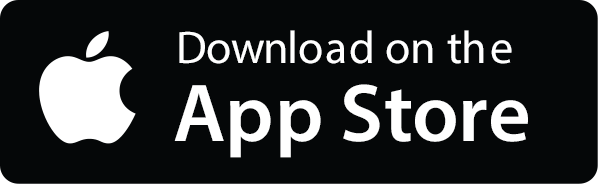
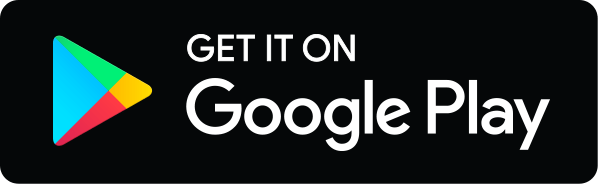