INTRODUCTION
SUMMARY
Iron deficiency and iron-deficiency anemia are common nutritional and hematologic disorders. In infants and young children, iron deficiency is most commonly caused by insufficient dietary iron. Rarely, it can result from mutations in TMPRSS6, a gene encoding a membrane protease that serves normally as a transcriptional suppressor of the primary negative regulator of iron absorption, hepcidin. In young women, iron deficiency is most often the result of blood loss in menstruation or as a result of blood loss during pregnancy, childbirth, and lactation. In older adults, bleeding is often the cause of iron deficiency, and may originate from the gastrointestinal tract, as from hemorrhoids, peptic ulcer, hiatus hernia, colon cancer, or angiodysplasia; from the genitourinary tract; from uterine leiomyomas or carcinoma, or a renal tumor; or from the pulmonary tree, through chronic hemoptysis caused by infection or malignancy, or as a result of idiopathic pulmonary hemosiderosis. Iron deficiency in infants can result in impairment of growth and intellectual development. The hematologic features of iron deficiency are nonspecific and too often confused with other causes of microcytic anemia such as thalassemias, chronic inflammation, and renal neoplasms. A low serum ferritin concentration is a good indicator of iron deficiency, but ferritin levels are increased by inflammation and can be particularly high in cancer, macrophage activation syndromes, hepatitis, or chronic kidney disease, which may mask the detection of iron deficiency coexisting with the anemia of chronic inflammation. The plasma iron is decreased and the iron-binding capacity increased in severe iron deficiency, but these alterations are not uniformly present in mild iron deficiency, and low plasma iron levels are also characteristic of the anemia of inflammation. Other laboratory tests that are useful include assays for serum transferrin receptor, reticulocyte hemoglobin content, percent hypochromic erythrocytes and erythrocyte zinc protoporphyrin. Diagnosis of iron deficiency, particularly in an adult, obliges the clinician to determine the site and cause of blood loss, and to rectify it whenever possible. Ferrous salts, in doses of 100 to 200 mg of elemental iron daily, are the initial treatment in most patients with iron deficiency. Enteric-coated and prolonged-release preparations should be avoided. Complete correction of anemia is expected in 8 to 12 weeks, depending on patient’s age. If this response is not achieved, the patient and the diagnosis require reevaluation. Administration of iron should be continued for 12 months after correction of anemia, or for as long as bleeding continues. Parenteral iron is used in patients who need more iron than can be delivered by the oral route, patients who do not tolerate oral iron salts, patients with gastrointestinal disease or following certain forms of bariatric surgery, noncompliant patients, and patients undergoing renal dialysis. All current parenteral iron preparations are much less likely to cause serious adverse events than was the case for high-molecular-weight iron dextran used in the past.
At the opposite end of the iron disorder spectrum, iron storage disease (hemochromatosis) can be the result of mutations of genes that are involved in regulation of iron homeostasis or transport, including the genes encoding HFE, transferrin receptor 2, ferroportin, hemojuvelin, and hepcidin. Because iron is not substantially excreted, iron overload commonly results from chronic erythrocyte transfusions for those anemias that are not caused by blood loss or iron deficiency.
Alternatively, iron overload resembling hereditary hemochromatosis can be the result of hyperabsorption of iron induced by ineffective erythropoiesis, including in β-thalassemias, dyserythropoietic anemias, pyruvate kinase deficiency, congenital dyserythropoietic anemias and some sideroblastic anemias. Here iron overload can develop even in the absence of erythrocyte transfusions or the (ill-advised) administration of medicinal iron, but is further aggravated by these events.
The diagnosis of systemic iron overload depends, in large part, upon increased serum ferritin levels accompanied by increased transferrin saturation, which tend to reflect increased iron stores. However, ferritin levels are also increased in patients with chronic inflammation or neoplasia or with the hyperferritinemia cataract syndrome, a disorder caused by mutations in the iron-responsive element of the ferritin light chain. The transferrin saturation is usually increased in patients with hereditary hemochromatosis even when the ferritin level is normal.
Many subjects with genetic hemochromatosis never develop organ dysfunction, those who do, their clinically significant hemochromatosis is characterized by cirrhosis of the liver, darkening of the skin, diabetes, cardiomyopathies, and possibly by arthropathies. Iron deposition is primarily in hepatocytes, with macrophages and intestinal mucosal cells being relatively iron poor. The most common causes of genetic hemochromatosis are mutations of the HFE gene. Two common mutations are involved: the c.854G→A (C282Y) and c.187C→G (H63D) substitutions. Increased transferrin saturation values, serum ferritin levels, and iron stores were found in a majority of homozygotes for the C282Y mutation and in many compound heterozygotes for C282Y/H63D or rarely in homozygotes for H63D. However, clinical manifestations even among homozygotes for the C282Y mutation are rare, in contrast to biochemical and/or histologic manifestations of the increased iron levels, which are common. Only a few percent of C282Y homozygous patients develop clinically significant disease, and cofactors including male gender and alcohol intake potentiate disease development. Juvenile hemochromatosis, an earlier onset and more severe type of hemochromatosis with high penetrance is the result of mutations of the hemojuvelin or the hepcidin gene. Ferroportin mutations produced two types of autosomal dominant iron overload. In one of these, the iron is deposited chiefly in macrophages; the other is similar to classical hereditary hemochromatosis with iron deposition in hepatocytes and other parenchymal cells.
Iron can be removed from patients with hereditary hemochromatosis by serial phlebotomy, but in patients with iron-loading anemias iron chelation therapy with either parenteral desferrioxamine infusions or the oral chelators deferiprone or deferasirox is required.
Acronyms and Abbreviations:
BMP, bone morphogenetic protein; cDNA, complementary DNA; DMT, divalent metal transporter; HFE, high iron (high Fe)—a mutated protein associated with common hereditary hemochromatosis; HLA, human leukocyte antigen; IL, interleukin; IRE, iron-responsive element; IRP, iron-regulatory protein; MCV, mean corpuscular volume; MRI, magnetic resonance imaging; RDA, recommended daily allowance; RDW, red cell distribution width; TfR, transferrin receptor; TIBC, total iron-binding capacity; UIBC, unsaturated iron-binding capacity.
IRON DEFICIENCY
Iron deficiency is the state in which the content of iron in the body is less than normal. Iron depletion is the earliest stage of iron deficiency, in which storage iron is decreased or absent but serum iron concentration, transferrin saturation, and blood hemoglobin levels are normal. Iron deficiency without anemia is a somewhat more advanced stage of iron deficiency, characterized by absent storage iron, usually low serum iron concentration and transferrin saturation, but without frank anemia. Iron-deficiency anemia, the most advanced stage of iron deficiency, is characterized by absent iron stores, low serum iron concentration, low transferrin saturation, and low blood hemoglobin concentration.
Chlorosis, or “green sickness,” was well known to European physicians after the middle of the 16th century. In France, by the middle of the 17th century, iron salts and other remedies (including, oddly enough, phlebotomy) were used in its treatment. Not long thereafter, iron was recommended by Sydenham as a specific remedy for chlorosis. For the 100 years preceding 1930, iron was used in the treatment of chlorosis, often in ineffective doses, although the mechanism of action of iron and the appropriateness of its use were highly controversial. By the beginning of the 20th century, it had been established that chlorosis was characterized by a decrease in the iron content of the blood and by the presence of hypochromic erythrocytes, but it was not until the classic 1932 studies by Heath, Strauss, and Castle1 that it was shown that the response of anemia to iron was stoichiometrically related to the amount of iron given and that chlorosis was, indeed, iron deficiency. The history of iron deficiency has been reviewed in greater detail elsewhere.2,3
Iron-deficiency anemia is the most common anemia worldwide, and is especially prevalent in women and children in regions where meat intake is low, food is not fortified with iron, and malaria, intestinal infections, and parasitic worms are common.4,5,6 Women with frequent pregnancies may be particularly susceptible. In the United States, iron deficiency is most common in children 1 to 4 years old and in adolescent, reproductive age, or pregnant women.7,8,9
Iron deficiency may occur as a result of chronic blood loss, diversion of iron to fetal and infant erythropoiesis during pregnancy and lactation, inadequate dietary iron intake, malabsorption of iron, intravascular hemolysis with hemoglobinuria, diversion of iron to nonhematopoietic tissues like the lung, genetic factors, or a combination of these factors. Of these, gastrointestinal or menstrual blood loss are the most common. As discussed in Chap. 42, the average adult male has approximately 1000 mg of iron in stores, but on average, women have less than half of this amount. The average daily dietary intake of iron is 10 to 12 mg, but much of this is not absorbed, even when absorption is maximal. Blood loss of each milliliter of packed erythrocytes represents 1 mg of iron. Thus chronic daily blood loss greater than 5 mL of erythrocytes will deplete iron reserves over weeks to months, and even if bleeding stops completely, the repletion of lost iron, including the restoration of iron stores (around 1000 mg in the average adult man), will take many months.
In men and in postmenopausal women, iron deficiency is most commonly caused by chronic bleeding from the gastrointestinal tract. Table 43–1 lists the causes of such blood loss. After history and physical examination rule out an obvious bleeding source in the genitourinary or respiratory tracts, evaluation of the gastrointestinal tract10 is necessary because of the potential that the pathologic process causing the blood loss is life-threatening. In the adult, the most common causes are peptic ulcer, erosion in a hiatal hernia, gastritis (including that caused by alcohol or aspirin ingestion), hemorrhoids, vascular anomalies (such as angiodysplasia), and neoplasms.
ALIMENTARY TRACT |
Esophagus |
Varices |
Stomach and duodenum |
Ulcer Hiatus hernia Gastritis Carcinoma Varices Angiodysplasia Hemangioma Leiomyoma (Ménétrier disease) Mucosal hypertrophy Hypergastrinemia Antral vascular ectasia “Watermelon stomach” |
Small intestine |
Vascular ectasia Tumors Ulceration Meckel’s diverticulum |
Colon and anorectal |
Hemorrhoids Carcinoma Polyp Diverticulum Ulcerative colitis Angiodysplasia Hemangioma Telangiectasia Amebiasis |
BILIARY TRACT |
Intrahepatic bleeding Carcinoma Cholelithiasis Trauma Ruptured aneurysm Aberrant pancreas |
GENITOURINARY TRACT |
Menorrhagia Uterine fibroids Endometriosis Carcinoma Vascular abnormalities |
RESPIRATORY TRACT |
Epistaxis Carcinoma Infections Telangiectases Idiopathic pulmonary hemosiderosis |
Gastritis as a result of drug ingestion is a common cause of bleeding. Aspirin, indomethacin, ibuprofen, and other nonsteroidal antiinflammatory drugs cause gastritis, but may also cause bleeding by inducing gastric or duodenal ulcers, or lesions in the small intestine11 and even the colon. Gastritis caused by alcohol ingestion12 can also cause significant blood loss. Chronic blood loss is often the cause of anemia in rheumatoid arthritis (perhaps because of the use of nonsteroidal antiinflammatory medications) and in inflammatory bowel disease.
Chronic blood loss from esophageal or gastric varices can lead to iron-deficiency anemia. Hemorrhoidal bleeding may lead to severe iron-deficiency anemia. Chronic blood loss may result from diffuse gastric mucosal hypertrophy (Ménétrier disease).13 Peptic ulcers of the stomach or duodenum are common causes of iron deficiency, and an association between infection with Helicobacter pylori and iron-deficiency anemia has been documented in numerous studies.14 Some of these iron-deficient patients who are infected with H. pylori do not respond to oral iron alone, but do respond to eradication of H. pylori.15
Gastric ulceration and bleeding can also occur in disorders of hypergastrinemia, as in Zollinger-Ellison syndrome and pseudo–Zollinger-Ellison syndrome. Although concerns were raised that long-term medical therapy of these disorders with proton pump inhibitors would also cause iron deficiency by raising gastric pH and making iron less soluble, this does not seem to be the case.16 Anemia that follows subtotal gastrectomy is usually attributed to reduced absorption of dietary iron (see “Malabsorption of Iron” below), but occult intermittent gastrointestinal bleeding from gastrointestinal lesions may also be a contributory factor, and requires endoscopic evaluation.17
Diaphragmatic (hiatal) hernia is often associated with gastrointestinal bleeding.18,19,20 The frequency of anemia ranges from 8 to 38 percent. Bleeding is much more likely to occur in patients with paraesophageal or large hernias than in those with sliding or small hernias. Mucosal changes cannot always be demonstrated by esophagoscopy or gastroscopy in patients who have had blood loss from hiatus hernia. However, a linear gastric erosion, also called a “Cameron ulcer,” commonly occurs on the crests of mucosal folds at the level of the diaphragm, and appears to be the site of bleeding.21
Hookworms are a major cause of gastrointestinal blood loss in many parts of the world.22
The lesions of angiodysplasia may occur in any part of the gastrointestinal tract.23 These tiny vascular anomalies may be the cause of significant blood loss. Endoscopy is usually required for diagnosis, and often needs to be repeated as bleeding can be intermittent. Gastric antral vascular ectasia24 exhibits a characteristic endoscopic appearance (“watermelon stomach”), and is another cause of blood loss. Hemorrhage into the biliary tract is a rare cause of chronic iron-deficiency anemia.25
Tortuous, dilated sublingual venous structures, the cherry hemangiomas commonly seen in the elderly, and the spider telangiectases of chronic liver disease are usually easily distinguished from the lesions of hereditary hemorrhagic telangiectasia. Bleeding from intestinal telangiectases has also been observed in scleroderma26 and in Turner syndrome,27 as a manifestation of bleeding from abnormal blood vessels. Cutaneous hemangiomas (blue rubber bleb nevus) may be associated with hemorrhage from intestinal hemangiomas.28
In hereditary hemorrhagic telangiectasia (Chap. 122), characteristic lesions commonly occur on fingertips, nasal septum, tongue, lips, margins (helices) of ears, oral and pharyngeal mucosa, palms and soles, and other epithelial and cutaneous surfaces throughout the body. Those lesions that occur in the gastrointestinal tract are particularly likely to bleed and to cause iron deficiency.
Meckel diverticulum is a very common abnormality representing a vestigial remnant of the omphalomesenteric duct. In children, bleeding from this structure accounts for a small proportion of cases of iron-deficiency anemia.29
Heavy menstrual bleeding30 is a very common cause of iron deficiency. The amount of blood lost with menstruation31 varies markedly from one woman to another and is often difficult to evaluate by questioning the patient. The average menstrual blood loss is approximately 40 mL per cycle. Blood loss exceeds 80 mL (equivalent to approximately 30 mg of iron) per cycle in only 10 percent of women. The volume of blood lost in the course of one menstrual cycle may be as high as 495 mL in apparently healthy, nonanemic women who do not regard their menstrual flow to be excessive. The amount of menstrual blood lost does not seem to vary markedly from one cycle to another for any given individual. Oral contraceptives reduce menstrual blood loss, but the use of an intrauterine coil for contraception increases menstrual blood loss, especially during the first year of use. Because the daily dietary intake is usually between 10 and 12 mg of iron and only a few milligrams of this can be absorbed, iron balance in many menstruating women is precarious.
Excessive bleeding may be caused by uterine fibroids and malignant neoplasms. Neoplasms, stones, or inflammatory disease of the kidney, ureter, or bladder may cause enough chronic blood loss to produce iron deficiency.
In the absence of hematuria, urinary iron losses as high as 1 mg/day have been reported in rare patients with nephrotic syndrome, some of whom had hypoferremia and hypochromic anemia.32 We found only one report of a patient in whom abnormally high urinary iron loss may have caused anemia without proteinuria or hematuria.33
Hemostatic defects, particularly those related to abnormal platelet function or number may lead to gastrointestinal bleeding, although unless the thrombocytopenia or platelet dysfunction is severe, gastrointestinal bleeding usually signifies an abnormality in the gastrointestinal tract. Gastrointestinal bleeding is common in von Willebrand disease (Chap. 126), but often because of coexistent peptic ulcer disease. Polycythemia vera is typically associated with iron deficiency as a result either of spontaneous gastrointestinal hemorrhage that commonly occurs in this disorder, or phlebotomy therapy, or both (Chap. 84).
When a patient with a disorder of hemostasis suffers from gastrointestinal bleeding, one must consider the possibility that the bleeding may not be caused by a hemostatic defect alone, but that an anatomic lesion of the gastrointestinal tract may also be present.
Iatrogenic anemia is particularly prevalent in intensive care units34 where repetitive blood sampling may result in removal of 40 to 70 mL of blood daily, and this iatrogenic phlebotomy can result in iron-deficiency anemia.
The use of extracorporeal dialysis for treatment of chronic renal disease may cause iron deficiency,35,36 often superimposed upon the anemia of chronic renal disease (Chap. 37). Patients treated with chronic hemodialysis experience multiple sources of blood loss with the dialysis equipment is a major cause, along with gastrointestinal bleeding, blood sampling and bleeding related to vascular access.
Each whole-blood donation removes approximately 200 mg of iron from the body. Lesser amounts of iron are removed in the course of donating platelets or leukocytes. Potential donors are screened in blood banks, so that those with frank anemia are not phlebotomized. Yet, by the time they are excluded from donation, some blood donors are iron depleted37,38,39 and may readily develop iron-deficiency anemia with relatively small additional blood loss.
Factitious anemia as a result of self-inflicted bleeding may present a formidable diagnostic and therapeutic problem. This rare condition has also been called, in literary allusion to a fictitious character, “Lasthénie de Ferjol syndrome” (in Barbey d’Aurevilly’s gloomy novel, Une Histoire Sans Nom), or part of Munchausen syndrome (based on the Rudolf Raspe book, The Surprising Adventures of Baron Münchausen).40,41 Most patients are women, and are often employed in a medical setting. There is often a history of numerous blood transfusions. The anemia is chronic and may be severe. The site of induced blood loss is obscure. Hence, patients are subjected to numerous radiographic and endoscopic examinations, usually to no avail. The patients are usually refractory to medical advice and therapy. The patients may be depressed and suicidal; some also suffer anorexia nervosa. Psychiatric care is needed, but often is unsuccessful. Rarely, the outcome of self-bleeding may be fatal.42
Ingestion of whole cow’s milk may induce protein-losing enteropathy and gastrointestinal bleeding in infants,43,44 probably on the basis of hypersensitivity or allergy. In four such cases observed endoscopically, erosive gastritis or gastroduodenitis was demonstrated as the probable source of bleeding. At least during the first year of life, children should not be given whole bovine milk, either raw or pasteurized. More protracted heating, as in preparation of infant formulas, eliminates this problem. Intrinsic lesions of the gastrointestinal tract, such as those listed above, may cause bleeding in infants, as well as in older children.
Persistent recurrent hemoptysis may lead to iron-deficiency anemia. It may be a result of congenital anomalies of the respiratory tract, endobronchial vascular anomalies, chronic infections, neoplasms, or valvular heart disease. Severe iron-deficiency anemia is a manifestation of idiopathic pulmonary hemosiderosis45 and of Goodpasture syndrome (progressive glomerulonephritis with intrapulmonary hemorrhage). In some of these disorders, hemoptysis may not be observed, but sufficient amounts of blood-laden sputum may be swallowed to result in positive tests for occult blood in the stools. Iron deficiency occurs in a large proportion of patients with cystic fibrosis,46,47 and occurs even in the absence of hemoptysis, suggesting that inflammatory inhibition of dietary iron absorption and iron loss in purulent sputum could contribute to the deficiency.
Although physiologic decrease in hemoglobin concentration is an expected consequence of hemodilution associated with pregnancy, true iron deficiency frequently results in more severe anemia. In pregnancy, the average iron loss resulting from diversion of iron to the fetus, blood loss at delivery (equivalent to an average of 150 to 200 mg of iron), and lactation is altogether approximately 900 mg; in terms of iron content, this is equivalent to the loss of more than 2 L of blood. Approximately 30 mg of iron may be expended monthly in lactation. Because most women begin pregnancy with low iron reserves, these additional demands frequently result in iron-deficiency anemia. Iron depletion has been reported in some 85 to 100 percent of pregnant women. Iron-deficient mothers are likely to have smaller babies. The incidence of anemia and iron deficiency is lower in women who take oral iron supplementation, daily or intermittently.48,49,50,51 In regions with endemic malaria, iron supplementation may increase the risk of malaria and some recommend that it be combined with malarial prophylaxis.52 Most experts agree that oral iron supplementation during pregnancy is desirable despite side effects. Increasing safety and convenience of parenteral iron therapy may lead to reevaluation of its role in the prevention and treatment of iron-deficiency anemia of pregnancy.53
In infants, iron deficiency is most often a result of the use of unsupplemented milk diets, which contain an inadequate amount of iron. During the first year of life, the full-term infant requires approximately 160 mg and the premature infant approximately 240 mg of iron to meet the needs of an expanding red cell mass. Approximately 50 mg of this need is fulfilled by the destruction of erythrocytes that occurs physiologically during the first week of life (Chaps. 7 and 33); the rest must come from the diet. Milk products are very poor sources of iron, and prolonged breast- or bottle-feeding of infants frequently leads to iron-deficiency anemia unless iron supplementation is implemented. This is especially true of premature infants. The European Society for Pediatric Gastroenterology, Hepatology, and Nutrition (ESPGHAN) Committee on Nutrition urges that all infant formulas be iron-fortified54; in North America, the use of iron-fortified formula is now generally accepted, but there is controversy about the appropriate level of fortification.55 In older children, an iron-poor diet may also contribute to the development of iron-deficiency anemia, particularly during rapid growth periods.
Infants and young women are usually in precarious iron balance, their iron intake being less than 80 percent of the recommended daily allowance (RDA).56 Fortification of bread and cereals with ferrous sulfate or metallic iron is commonplace. This practice was suspended in Sweden because of concern for the possibility of increasing iron storage in patients with the hemochromatosis genotype, resulting in increased incidence of iron-deficiency anemia.57
The scant iron supply of the American diet places young women and children at particular risk of negative iron balance. Because the adult male needs to absorb only approximately 1 mg iron daily from his diet to maintain normal iron balance, iron deficiency in older men is very rarely caused by insufficient dietary intake alone.
Gastric secretion of hydrochloric acid is often reduced in iron deficiency.58 Histamine-fast achlorhydria has been found in as many as 43 percent of patients with iron deficiency. Gastric function may improve after correction of the iron deficiency, so that iron deficiency may be both a cause and a result of impairment of gastric iron secretion. However, in persons older than the age of 30 years, the achlorhydria is usually irreversible. Furthermore, when atrophic gastritis coexists with iron deficiency, no improvement in gastric secretory function has followed iron therapy. Autoimmune gastritis, which is often associated with H. pylori infection,14,15 may play an important role in both iron-deficiency anemia and, in later life, in the development of pernicious anemia.
Intestinal malabsorption of iron is quite an uncommon cause of iron deficiency except after gastrointestinal surgery and in malabsorption syndromes. Ten to 34 percent of patients who have undergone subtotal gastric resection develop iron-deficiency anemia years later. Many such patients have impaired absorption of food iron, caused in part by more rapid gastrojejunal transit and in part by partially digested food bypassing some of the duodenum as a result of the location of the anastomosis. Fortunately, medicinal iron is well absorbed in post–partial gastrectomy patients. Moreover, gastrointestinal blood loss may also play an important role in anemia following gastric resection (see “Gastrointestinal Blood Loss” earlier). In malabsorption syndromes, absorption of iron may be so limited that iron-deficiency anemia develops over a period of years. Celiac disease, whether overt or occult, may be associated with iron-deficiency anemia.14,15,59,60
Iron-deficiency anemia may occur in paroxysmal nocturnal hemoglobinuria (Chap. 40) and in hemolysis resulting from mechanical erythrocyte trauma from intracardiac myxomas, valvular prostheses (particularly if malfunctioning), or patches (Chaps. 33 and 51). In these disorders, up to 10 mg/day of iron is lost in the urine as hemosiderin and ferritin in desquamated tubular cells and as hemoglobin dimers, an amount sufficient to cause systemic iron deficiency.61,62
Iron deficiency occurs frequently in athletes engaged in a variety of sports (Chaps. 33 and 51), especially female athletes.63 There may be mild anemia. Increased intravascular hemolysis, presumably with some renal loss of iron, may play a role, but gastrointestinal blood loss has also been demonstrated in persons engaged in strenuous athletic pursuits. Hemoglobinuria and hemosiderinuria are also seen in competitive and recreational runners, that is, march hemoglobinuria (Chaps. 33 and 51). Strenuous exercise also elicits a rise in serum interleukin (IL)-6 and hepcidin, and this could decrease dietary iron absorption.63
Women soldiers undergoing basic training experience iron depletion as determined by serum ferritin measurements, and this can be partially reversed by iron supplementation.64 The etiology may be similar to the iron deficiency seen in athletes.
Based on twin studies,65 genetic factors play a role in iron deficiency. Mutations in multiple genes, including HFE and transferrin, show weak associations with iron stores but only mutations of the membrane serine protease Tmprss666 have been identified in genome-wide association studies as genetic factors that cause or predispose to iron deficiency. The genetic syndrome of iron-refractory iron deficiency anemia is mediated by inappropriately increased hepcidin as a result of homozygous or compound heterozygous mutations in Tmprss6.67,68,69 Increased hepcidin diminishes iron absorption and causes inappropriate retention of available iron in splenic macrophages and Kupffer cells.
As iron deficiency develops, different compartments are depleted in iron in an overlapping sequence, as illustrated schematically in Fig. 43–1.
Figure 43–1.
Stages in the development of iron deficiency. Early iron deficiency (iron depletion) is usually not accompanied by any abnormalities in blood; at this stage, serum iron concentration is occasionally below normal values and storage iron is markedly depleted. As iron deficiency progresses, development of anemia precedes appearance of morphologic changes in blood, although some cells may be smaller and paler than normal; serum iron concentration is usually low at this time, but it may be normal. With advanced iron depletion, classic changes of hypochromic, microcytic, hypoferremic anemia become manifest. (Reproduced with permission from Lichtman’s Atlas of Hematology, www.accessmedicine.com.)
As the body becomes depleted of iron, changes occur in many tissues. Hemosiderin and ferritin virtually disappear from marrow and other storage sites. Hemoglobin synthesis in the marrow decreases, first as a result of fewer erythroblasts,70 but eventually also per erythroblast if iron deficiency becomes more severe, resulting in hemoglobin-deficient erythrocytes. The concentration of many other iron-containing proteins is affected, often in an organ-specific manner.71 Studies in laboratory animals on defined iron-deficient diets are most informative about this process, because human iron deficiency is often confounded by other forms of malnutrition. In such models of severe (pure) iron deficiency, skeletal muscle myoglobin is mildly depleted but cardiac myoglobin is not. Cytochromes and other mitochondrial ferroproteins are depleted but selectively so. Since these classical studies were performed, it has become apparent that the synthesis of many ferroproteins is regulated in an iron-dependent manner, mainly via the iron-responsive element (IRE)/iron-regulatory protein (IRP) system (Chap. 42). The changes in iron-containing proteins may thus be adaptive,72 to allow the survival of the organism until more iron becomes available.
Decrements in high-intensity exercise performance can be detected even during nonanemic iron deficiency,63 and worsen with increasing anemia.73 The limitation of high-level exercise by oxygen delivery, and, therefore, hemoglobin content of blood, is well known, and has given rise to surreptitious blood doping and erythropoietin abuse by some athletes. The impairment of performance during nonanemic iron deficiency consists of decreased spontaneous activity (seen in humans and in animal models) and decreased ventilatory threshold, that is, the point at which ventilation starts increasing more rapidly than oxygen consumption.74 Other deficits that have been reported include decreased endurance and increased muscle fatigue. The biochemical basis of the deficits associated with nonanemic iron deficiency is not well understood but is attributed to the depletion of iron-containing mitochondrial proteins that are involved in energy metabolism.63 The condition is reversible with iron supplementation.
Iron deficiency is associated with both developmental abnormalities in children and with restless leg syndrome in adults, but in neither case has iron deficiency been established as the primary cause.75,76 The substantia nigra is a particularly iron-rich region of the brain and contains dopaminergic neurons that are suspected of involvement in restless leg syndrome. In mouse models of iron deficiency, iron depletion of the substantia nigra is highly strain-dependent,77 suggesting that iron deficiency and as yet incompletely characterized genetic variations may cooperate in the pathogenesis of restless leg syndrome by allowing the depletion of iron from susceptible brain regions involved in dopaminergic signaling.78
In multiple publications, iron deficiency has been reported to impair various immune functions, but the effects appear minor and inconsistent.79 Perhaps surprisingly, the evidence for a narrowly protective and proinflammatory effect of iron deficiency appears stronger. Iron deficiency decreases the risk and severity of malaria,80,81 and iron supplementation may have the opposite effect, especially when not targeted to patients with iron deficiency.52,82 The mechanism of this effect is of great interest but not yet well understood.83 There are some indications that iron deficiency may have a proinflammatory effect. In a mouse model, iron deficiency potentiated the systemic effect of lipopolysaccharide in a hepcidin-dependent manner,84 and in a mouse model of asthma, iron deficiency promoted allergic inflammation.85
Iron-deficient children have been reported to suffer from growth retardation but it is difficult to isolate the effect of iron deficiency from other nutritional and environmental causes of stunting. Two comprehensive analyses of randomized controlled trials did not detect an effect on growth of iron supplementation alone.86,87 Decreased thermoregulation in response to cold exposure is seen in both humans and laboratory models.88 It has been attributed to the conflicting effects on blood flow of decreased oxygen content of blood and need to minimize heat loss, as well as the effect of iron deficiency on thyroid function.
Severe iron deficiency may lead to histologic changes in various organs. The rapidly proliferating cells of the upper part of the alimentary tract seem particularly susceptible to the effect of iron deficiency. There may be atrophy of the mucosa of the tongue and esophagus,89 stomach,90 and small intestine.91 The epithelium of the lateral margins of the tongue is reduced in thickness despite increase in the progenitor compartment. This thinning presumably reflects accelerated exfoliation of epithelial cells.92 Buccal mucosa has shown thinning and keratinization of epithelium and increased mitotic activity.93,94 However, light microscopic and electron microscopic examination of exfoliated oral mucosal cells showed no aberrations in morphology of nuclei or cytoplasm of the cells of patients with iron-deficiency anemia.95 In iron-deficiency anemia resulting from idiopathic pulmonary hemosiderosis, characteristic pathologic changes are found in the lungs, including intense deposition of iron in the littoral cells of the alveoli and interstitial fibrosis.45
Widening of diploic spaces of bones, particularly those of the skull and hands,96,97,98 may be a consequence of chronic iron deficiency beginning in infancy. In the skull, this is of the same character as in thalassemia, except that in β-thalassemia major there is maxillary hypertrophy, whereas in severe iron-deficiency anemia maxillary growth and pneumatization are normal.
The anemia in iron-deficient patients can be very severe, with blood hemoglobin levels as low as less than 4 g/dL being encountered in some patients. Severe iron-deficiency anemia is associated with all of the various symptoms of anemia, resulting from hypoxia and the body’s response to hypoxia, as described in Chap. 32. Thus, tachycardia with palpitations and pounding in the ears, headache, light-headedness, and even angina pectoris, may all occur in patients who are severely anemic.
The clinical features of iron deficiency encompass nonhematologic effects and symptoms caused by the anemia itself. A number of controlled studies show that various manifestations of iron deficiency can occur in individuals whose hemoglobin is within the accepted normal range.
Objective measurements of work performance and studies using O2 consumption as an index of work performance have given contradictory results, but a comprehensive review led to the conclusion that severe iron-deficiency anemia (hemoglobin <8 g/dL) and mild iron deficiency anemia (hemoglobin between 8 and 12 g/dL) led to decreased work performance, primarily as estimated by peak oxygen consumption (VO2max) measurements, but the evidence that nonanemic iron deficiency had such an effect was less convincing.99 However, in athletes with low ferritin levels but normal hemoglobin levels, iron-supplemented subjects showed an increased VO2max without a change in their red cell mass, and in other studies nonanemic subjects treated with iron showed improved performance and/or VO2max.63
It has been proposed that iron deficiency in infants and children is associated with poor attention span, poor response to sensory stimuli, and retarded behavioral and developmental achievement even in the absence of anemia. The causality of these associations is confounded by other coexisting nutritional deficits and socioeconomic deprivation, so reversibility by iron supplementation would be important in establishing causality. However, in systematic meta-analyses, iron supplementation had weak or no effects on these deficits.86,100,101,102
It has been speculated that there is a relationship between restless legs syndrome, Tourette syndrome, and attention deficit hyperactivity disorder and that iron deficiency contributes to their pathophysiology. Restless legs syndrome, a common nocturnal problem, especially in the elderly, is associated with iron deficiency and is reported to improve on iron therapy, but the beneficial effects are inconsistent and not well predicted by blood ferritin or transferrin saturation.75,103,104 In children there may be a relationship between iron deficiency and attention deficit hyperactivity disorder, but the association is inconsistent.105
Breath holding in children, headaches, and paresthesias have been attributed to iron deficiency but there are no controlled studies to support these impressions. Anecdotal reports of intracranial hypertension with papilledema are buttressed by apparent response to iron therapy.106,107,108,109 Stroke in children and in adults, possibly triggered by thrombocytosis, is associated with iron-deficiency anemia.110,111,112,113,114
Burning of the tongue has also been described anecdotally in many accounts of iron deficiency, and although this symptom has been observed to diminish with treatment, no controlled studies have been performed. The tongue symptoms could be a result of concurrent pyridoxine deficiency. Although iron deficiency has been proposed as a cause of atrophic rhinitis, the evidence for this is weak.
In the laryngopharynx, mucosal atrophy may lead to web formation in the postcricoid region, thereby giving rise to dysphagia (Paterson-Kelly also known as Plummer-Vinson syndrome).115 If these alterations are of long duration, they may lead to pharyngeal carcinoma. Although it has been generally thought that these changes are secondary to longstanding iron deficiency, this mechanism is not universally accepted. The frequency of the condition is considered to have decreased considerably, and it is remarkably rare in many parts of the world where iron deficiency is common.
The craving to eat unusual substances, for example, dirt, clay, ice, laundry starch, salt, cardboard, and hair, is a well-documented manifestation of iron deficiency and is usually cured promptly by iron therapy.116,117,118,119
Although the association of hair loss with iron deficiency is controversial,120 low ferritin levels were a risk factor for hair loss in a large multivariate analysis.121 Remarkably, hair loss sparing the face (“mask mouse”) is a sign of iron deficiency in mice.122
The physical findings in iron-deficiency anemia include pallor, glossitis (smooth, red tongue), stomatitis, and angular cheilitis. Koilonychia (spoon nails), once a common finding, is now encountered rarely. Retinal hemorrhages and exudates may be seen in severely anemic patients (e.g., hemoglobin concentration of <5 g/dL). Splenomegaly has occasionally been attributed to iron-deficiency anemia, but when it occurs, it is probably from other causes.
In severe, uncomplicated iron-deficiency anemia, the erythrocytes are hypochromic and microcytic; the plasma iron concentration is diminished; the iron-binding capacity is increased; the serum ferritin concentration is low; the serum transferrin receptor (TfR) and erythrocyte zinc protoporphyrin concentrations are increased; and the marrow is depleted of stainable iron. However, the classic combination of laboratory findings occurs consistently only when iron-deficiency anemia is far advanced, when there are no complicating factors such as infection or malignant neoplasms, and when there has not been previous therapy with transfusions or parenteral iron.
Anisocytosis is the earliest recognizable morphologic change of erythrocytes in iron-deficiency anemia (Fig. 43–2).123 The anisocytosis is typically accompanied by mild ovalocytosis. As the iron deficiency worsens, a mild normochromic, normocytic anemia often develops. With further progression, hemoglobin concentration, erythrocyte count, mean corpuscular volume (MCV), and mean erythrocyte hemoglobin content all decline together.124,125 As the indices change the erythrocytes appear microcytic and hypochromic on stained blood films. Target cells may sometimes be present. Elongated hypochromic elliptocytes may be seen, in which the long sides are nearly parallel. Such cells have been called “pencil cells,” although they more nearly resemble cigars in shape. The red cell indices are consistently abnormal in adults only when iron-deficiency anemia is moderate or severe (e.g., in males with hemoglobin concentrations <12 g/dL or in women with hemoglobin concentrations <10 g/dL) (Fig. 43–3). The distribution of erythrocyte volume (e.g., red cell distribution width [RDW]) is usually increased in established iron-deficiency anemia. The RDW is reported often as the coefficient of variation (in percent) of erythrocyte volume (see “Differential Diagnosis” below).
Figure 43–2.
Variability in morphologic diagnosis of iron-deficiency anemia from blood film. As in all deficiency states leading to anemia, the blood film morphology and blood cell changes are a function of the severity of the deficiency. A. Normal blood film. Normocytic-normochromic red cells with normal shape. B. Mild iron deficiency. Serum iron, ferritin, and transferring saturation were consistent with mild iron deficiency. Cannot discern if mean red cell size has decreased. There may be a few red cells that have larger central pallor, but that is arguable. A few cells have oval or elliptical shape. C. Severe iron deficiency. Serum iron, ferritin, and transferring saturation were consistent with severe iron deficiency. Note obvious increase in overtly hypochromic cells and higher frequency of microcytes. (Reproduced with permission from Lichtman’s Atlas of Hematology, www.accessmedicine.com.)
Figure 43–3.
Erythrocyte indices in iron-deficiency anemia of adults; data obtained with Coulter Counter, Model S. Normal ranges of indices observed in approximately 500 healthy adults using the same instrument are indicated by shading. The dashed line in the top panel indicates the more widely accepted lower normal limit of mean corpuscular hemoglobin concentrations (MCHCs) stated in this text. (Top) Correlation between venous blood hemoglobin concentration and MCHC. More than half of 62 patients with iron-deficiency anemia had MCHC values clearly in the normal range. (Bottom) Correlation between venous blood hemoglobin concentrations and mean corpuscular volume (MCV). Nearly 70 percent of cases exhibited distinct microcytosis. Thus when indices are determined by automated cell-counting methods, the MCV is much more sensitive than is the MCHC in detecting changes of iron deficiency. However, at least 30 percent of cases of iron-deficiency anemia will be misdiagnosed if physicians rely on the erythrocyte indices. (Data from Klee GG: Decision Rules for Accelerated Hematology Laboratory Investigation: Thesis, University of Minnesota.)
Leukopenia has been found in some patients with iron-deficiency anemia, but the overall distribution of leukocyte counts in iron-deficient patients seems to be approximately normal.
Both thrombocytopenia126 and, more commonly, thrombocytosis127 have been associated with iron deficiency. Platelet abnormalities correct with iron therapy. Thrombotic complications of iron deficiency have been reported but are very rare.128 The etiology of either abnormality is not known. Low-iron-diet-induced iron-deficiency anemia developed in a rat model within 2 weeks, and this was accompanied by sustained 50 percent increase in platelet count with increased platelet size but without significant changes in known megakaryocyte growth factors (thrombopoietin, IL-6 or IL-11). It has been suggested that high erythropoietin levels may stimulate thrombopoietin receptors because the two hematopoietic factors are structurally related, but this does not seem to be the case.129
Reticulocyte count is often mildly increased,130 a finding consistent with the increased erythroid activity of the marrow (see “Marrow” below).
Because most of the iron in the body is normally in erythrocytes, and iron is not excreted, decrease in erythrocyte mass generally results in increased storage iron. Iron-deficiency anemia is the exception, as iron stores are depleted before the red cell mass is compromised. Thus, evaluation of iron stores should be a sensitive and usually reliable means for the differentiation between iron-deficiency anemia and all other anemias. Decreased or absent hemosiderin in the marrow is characteristic of iron deficiency, and is readily evaluated after staining by the simple Prussian blue method. Stored iron in the macrophages of the marrow can be seen in marrow spicules in marrow sections, or in marrow aspirate films. Iron granules, normally found in the cytoplasm of approximately 30 percent of erythroblasts, become rare but may not be entirely absent.
Evaluation of the amount of iron in marrow macrophages has long been considered the “gold standard” for the diagnosis of iron deficiency. There are, however, technical barriers to the accurate histochemical determination of marrow iron. First, an invasive procedure, marrow aspiration, is required. Second, the differentiation of iron within macrophages from artifacts takes experience and skill. In one study only 74 of 108 cases had been accurately reported.131 Moreover, misleading results may be obtained in patients who have been transfused or who have been treated with parenteral iron.132 The marrow of such patients may contain normal, or even increased, quantities of stainable iron in the face of typical iron-responsive iron-deficiency anemia. In such patients, iron that is seen on marrow examination is not readily available for erythropoiesis. As serum markers of iron deficiency became widely available, the reasons for the primacy of marrow iron estimation have been questioned.133
The serum iron concentration is usually low in untreated iron-deficiency anemia, but may rarely be normal.125,134,135 Iron in blood plasma turns over every few hours and constitutes less than 0.1 percent of total body iron in adults, so iron concentrations are readily perturbed by transient changes in iron supply or demand. Physiologically, the serum iron concentration has a diurnal rhythm; it decreases in late afternoon and evening, reaching a nadir near 9 pm and increases to its maximum between 7 and 10 am. This effect is rarely of sufficient magnitude to influence diagnosis.136 Serum iron levels decrease at about the time of menstrual bleeding137,138 regardless of whether the bleeding is physiologic or induced by withdrawal of contraceptive hormonal preparations.
Importantly, the serum iron concentration is reduced in the presence of either acute or chronic inflammatory processes139 or malignancy140 and following acute myocardial infarction.141,142 The serum iron concentration under these circumstances may be decreased sufficiently to suggest iron deficiency. Conversely, during chemotherapy of malignancy, the serum iron concentration may be quite elevated, as cytotoxic effects of the drugs on erythroblasts inhibit erythropoiesis and related iron uptake by erythroblasts. This effect is observed from the third to the seventh day after inception of chemotherapy of a variety of tumors.143
Normal or high concentrations of serum iron are commonly observed even in patients with iron-deficiency anemia if such patients receive iron medication before blood is drawn for these measurements. Even multivitamin preparations, which commonly contain approximately 18 mg of elemental iron per tablet, can result in this effect. Oral iron medication should be withheld for 24 hours before blood samples are obtained. Parenteral injection of iron dextran may result in a very high serum iron concentration (e.g., 500 to 1000 mcg/dL), at least with some methods,144 for several weeks. The elevation of serum iron levels after infusion of sodium ferric gluconate or iron sucrose is of much shorter duration.145
The iron-binding capacity is a measure of the amount of transferrin in circulating blood. Normally, there is enough transferrin present in 100 mL serum to bind 4.4 to 8.0 μmol (250 to 450 mcg) of iron; because the normal serum iron concentration is approximately 1.8 μmol/dL (100 mcg/dL), transferrin may be found to be approximately one-third saturated with iron. The unsaturated or latent iron-binding capacity (UIBC) is easily measured with radioactive iron or by spectrophotometric techniques. The sum of the UIBC and the plasma iron represents total iron-binding capacity (TIBC). TIBC can also be measured directly. In iron-deficiency anemia, UIBC and TIBC are often increased and serum iron concentrations are decreased so that transferrin saturation of 15 percent or less is usually found. Because transferrin concentration and TIBC are decreased during inflammation, a normal value for transferrin saturation often accompanies a low serum iron concentration in the anemia of chronic inflammation.
Serum ferritin, secreted mainly by macrophages146 and hepatocytes, contains relatively little iron, yet serum ferritin concentration empirically correlates with total-body iron stores,147 for reasons that are still obscure. Serum ferritin concentrations of 10 mcg/L or less are characteristic of iron-deficiency anemia. In iron deficiency without anemia, serum ferritin concentration is typically in the range of 10 to 20 mcg/L. An increase in serum ferritin concentration occurs in inflammatory disorders, such as rheumatoid arthritis, in chronic renal disease, and in malignancies.148 When one of these conditions coexists with iron deficiency, as they often do, the serum ferritin concentration is commonly in the normal range; interpretation of results of this assay then becomes difficult. In patients with rheumatoid arthritis who are anemic, some suggest that concomitant iron deficiency may be suspected when the serum ferritin concentration is less than 60 mcg/L,149 but such empiric guidelines are unlikely to apply to the full spectrum of severity of inflammation. Increased serum ferritin concentrations are also characteristic of some malignancies, as well as of acute and chronic liver disease and chronic renal failure.150,151,152,153 In Gaucher disease, juvenile rheumatoid arthritis, and various macrophage activation syndromes, and in ferroportin disease characterized by massive iron loading of macrophages, the serum ferritin concentration is commonly in the range of thousands of mcg/L and may mask iron deficiency.154,155,156,157,158
Erythrocyte protoporphyrin, principally zinc protoporphyrin, is increased in disorders of heme synthesis, including iron deficiency, lead poisoning, and sideroblastic anemias, as well as other conditions.159,160,161 This assay analyzes the fluorescence of erythrocytes and uses small blood samples. It is quite sensitive in the diagnosis of iron deficiency and practical for large-scale screening programs designed to identify children with either iron deficiency or lead poisoning.58,159 It does not differentiate between iron deficiency and anemia that accompanies inflammatory or malignant processes.162
The role of TfR in transporting transferrin iron into cells is described in Chap. 42 section “Transport of Iron”. The circulating receptor is a truncated form of the cellular receptor, lacking the transmembrane and cytoplasmic domains of the cellular receptor. It circulates bound to transferrin. Sensitive immunologic methods can detect approximately 5 mg/L of receptor in serum. The levels of circulating TfR mirror the amount of cellular receptor, and therefore are proportional to the number of erythroblasts expressing the receptor. Because receptor synthesis is greatly increased when cells lack iron, the amount of the circulating receptor increases in iron deficiency.163,164 In anemia of inflammation, the synthesis of the TfR is suppressed by cytokines and this negates the opposing stimulatory effect of iron restriction, resulting in a lower serum TfR concentration than in pure iron deficiency.165 This test for iron deficiency has gradually come into clinical use, but the methodology has not yet been standardized, making laboratory-to-laboratory comparisons difficult. A method for performing reproducible assays for the soluble TfR has been standardized.166 Like the serum ferritin and serum iron, serum TfR assay results may be confounded by poorly understood variations in patients with malignancies; in patients in whom the serum TfR concentration is reduced; and in patients with asymptomatic malaria or thalassemia trait,167,168 in whom, in the absence of iron deficiency, it is increased. The ratio of serum TfR to serum ferritin seems to be a useful but not infallible reflection of body iron stores.169 Moreover, several studies show that the soluble transferrin index calculated as a ratio of the serum TfR/log ferritin (TfR-F Index) may be superior to other means for detection of iron deficiency.170,171,172
Some automated hematology instruments offer a method for diagnosis of iron deficiency using an assay of hemoglobin content within reticulocytes. This parameter is an indicator of iron restriction of hemoglobin synthesis during 3 to 4 days prior to the test.173,174 Percent hypochromic erythrocytes offers a longer term assessment of iron restriction during the preceding few months.173,175
Iron-deficiency anemia is characterized by many abnormal laboratory features. Because none of these are unique, a small deviation from normal will detect most cases of iron deficiency (high sensitivity), but also falsely identify non–iron-deficient subjects as being iron deficient (low specificity). On the other hand, a large deviation from normal will exclude most nondeficient patients (high specificity), but miss many iron-deficient subjects (low sensitivity). This tradeoff is shown graphically in so-called receiver operator characteristic curves. These curves are constructed by plotting the sensitivity against the false-positive rate (1 − specificity) at various values of the analyte. Figure 43–4
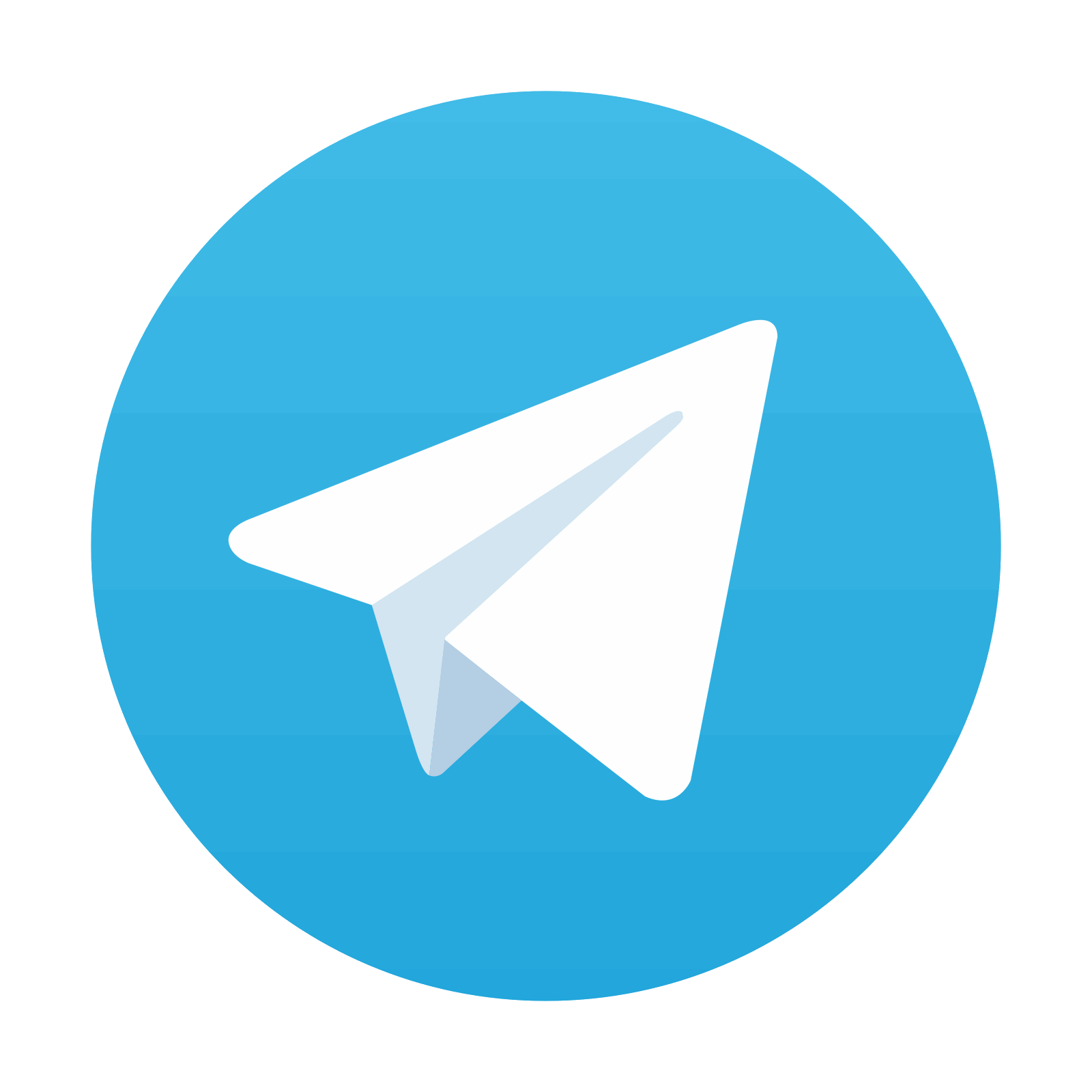
Stay updated, free articles. Join our Telegram channel

Full access? Get Clinical Tree
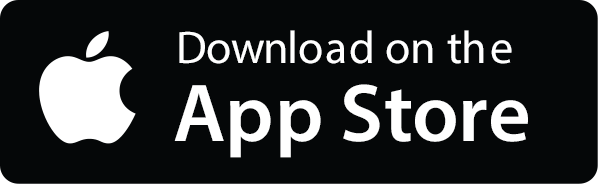
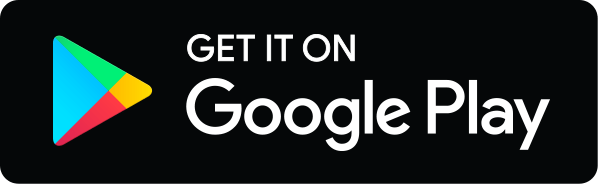
