Abstract
Although breast cancer tumors tend to have less tumor immune infiltration than immunogenic tumors such as melanoma and colon cancer, the prognostic importance of tumor immune infiltrates in breast cancer has confirmed the essential role of the immune response to the breast tumor. Increased tumor immune infiltrate, particularly antitumor type 1 (Th1) immune infiltrate, has been shown to predict improved disease-free and overall survival, particularly in triple-negative breast cancer, and to influence the response to chemotherapy. Furthermore, autoantibodies against tumor antigens are present in patients even before breast cancer development but not in women who will not develop breast cancer, and these autoantibodies may provide both early diagnosis and response to therapy biomarkers. Finally, the generation of clinical responses to emerging therapies including vaccines and immune checkpoint inhibitor monoclonal antibodies has clearly demonstrated that breast cancer is immunologically active. In the future, therapies that harness the immune system will be used in all stages of breast cancer treatment including the possibility of stimulating immunity for breast cancer prevention. This chapter provides an overview of current immunologic approaches to develop predictive and prognostic assays in breast cancer and novel, clinically effective immune therapy; it also provides an overview of considerations for new directions in breast immunooncology for the future.
Keywords
breast cancer tumor immune environment, tumor-infiltrating lymphocytes, breast cancer vaccines, checkpoint inhibitors, adoptive T-cell therapy, breast cancer immunoprevention.
The emergence of the prognostic importance of tumor immune infiltrates in breast cancer, the essential role of the immune system in contributing to the response to chemotherapy, and the generation of clinical responses with emerging therapies including vaccines and immune checkpoint inhibitor monoclonal antibodies have clearly demonstrated that breast cancer is immunologically active. In the future, therapies that harness the immune system will be used in all stages of breast cancer treatment, including the possibility of stimulating immunity for breast cancer prevention. This chapter provides an overview of the current immunologic approaches to develop predictive and prognostic assays in breast cancer, novel and clinically effective immune therapy, and will overview considerations of new directions in breast immuno-oncology for the future.
Immunity and Breast Cancer
The human immune system is generally categorized as providing either an innate immune response or an adaptive immune response. Innate immune cells are the body’s first responders to a foreign insult, and the innate immune response does not require recognition of proteins or antigens. The innate response is short-lived; no immunologic memory is generated. Adaptive immunity does require antigen recognition and is a slower response because antigens have to be processed and presented to T cells by innate immune cells. Immunologic memory can be elicited in an adaptive response so that protective immunity can be generated in anticipation of a future threat by the same organism.
Some cells of the innate immune system demonstrate antitumor activity. Natural killer T cells (NK T cells) respond to stress signals, including loss of major histocompatibility complex (MHC) class I expression and can both trigger direct cytotoxicity of tumor cells and release cytokines that promote adaptive immunity. Increased tumor-infiltrating NK T cells and the presence of NK T-cell–associated gene expression patterns in tumors have been shown to predict improved outcome for breast cancer patients. Other cells of the innate immune system suppress potentially functional immunity. Macrophages are antigen-presenting cells and are needed for displaying immunogenic proteins to the cells of the adaptive immune system (lymphocytes). In response to environmental signals, macrophages also release cytokines that influence the phenotype of the evolving adaptive immune response. M1 macrophages secrete type I lymphocyte-activating cytokines (including interferon gamma [IFN-γ], interleukin [IL]-2, and IL-12) that promote the generation of a CD8 + T-cell (cytotoxic T lymphocytes, CTL) response. M2 macrophages secrete type II cytokines including transforming growth factor-beta (TGF-β), IL-4, and IL-13 that inhibit proliferation of CD8 + T cells and facilitate antibody formation. Intratumoral macrophages in breast cancer are predominantly M2 and have been associated with poor prognosis. In a study of 216 breast cancer samples, increased proliferating macrophages (CD68 + , a macrophage marker, and PCNA + , an anti–proliferating cell nuclear antigen, a proliferation marker) in breast tumors were associated with an increased risk of death (hazard ratio [HR] 1.75, 95% confidence interval [CI] 1.0–3.0; p = .048). An investigation in almost 200 patients with locally advanced breast cancer demonstrated patients whose tumors did not express a macrophage-associated gene signature enjoyed a greater clinical benefit after chemotherapy and improved survival compared with patients whose tumors displayed such a signature ( p = .0009).
Another innate immune cell type that is associated with tumor progression and advanced stages of breast cancer is the myeloid-derived suppressor cell (MDSC). MDSCs are a population of immature myeloid cells that trigger immune suppression by producing reactive oxygen species and secrete immune-suppressive cytokines. The presence of MDSCs has been shown to predict an unfavorable prognosis in breast cancer, with an increase in circulating peripheral blood MDSCs associated with progression of disease. The highest levels of circulating MDSCs are found in metastatic breast cancer. MDSCs may be particularly important in enhancing the development of bone metastasis. MDSCs have been proposed to be osteoclast progenitors contributing both to bone resorption and modification of the bone immune environment in a manner that promotes increased breast cancer metastases. The cells of the innate immune system play a critical role in helping to define the immune microenvironment for a particular tumor and can significantly influence the adaptive immune response that develops in breast cancer.
Adaptive immunity requires interaction with a specific antigen ( Fig. 71.1 ). Many antigens are available for immune recognition. These include tumor-associated antigens that are not very different from self (see Fig. 71.1B ) and antigens that may be mutated or phosphorylated to appear more dangerous to the immune system (see Fig. 71.1C ). Interaction with antigen displayed in the MHC is the first signal to the immune system of the strength of the immune response needed upon antigen recognition. Adaptive immunity is defined by the presence of lymphocytes, either T or B cells, and includes both CD8 + cytotoxic T cells that are the effector cells that directly destroy tumor cells, CD4 + helper T cells that regulate CD8 + T-cell and B-cell function, and B cells that present antigen and produce antibodies. Type 1 (Th1) CD4 + T helper cells secrete cytokines that support the function of CTL. Type 2 (Th2) CD4 + helper T cells secrete cytokines that support B-cell proliferation and antibody production. Regulatory CD4 + helper T cells (Tregs) are a third type of T cell that modifies the tumor immune environment. Tregs secrete cytokines that dampen an inflammatory response and play a role in preventing autoimmunity. Tregs are defined by Forkhead box P3 (FOXP3) expression and are operative in cancer as many immunogenic proteins are nonmutated self-antigens that are aberrantly expressed. Antigen-specific Tregs have been defined that function to prevent immunity to self as a mechanism of limiting potential autoimmunity. Recent studies have suggested that Th1 immunity is needed for cancer eradication. In examining 20 tumor types, including breast cancer, increased Th1/CD8 + tumor immune infiltrates predicted improved clinical outcomes whereas increased Th2/Treg tumor immune infiltrates predicted inferior outcomes. In breast cancer, Th1 tumor-infiltrating T cells, as defined by the expression of Tbet, have been shown to impart a favorable prognosis. In a study of more than 600 tumors derived from breast cancer patients, a lack of Tbet positive cells in the tumor was associated with a reduced disease-free survival compared with Tbet high tumors in multivariate analysis (relative risk [RR] 5.62, 95% CI 1.48–50.19, p = .0027). However, the majority of breast tumors display a Th2 immune environment including Th2 and Treg cells that inhibit CD8 + T-cell function and dampen the antitumor immune response.
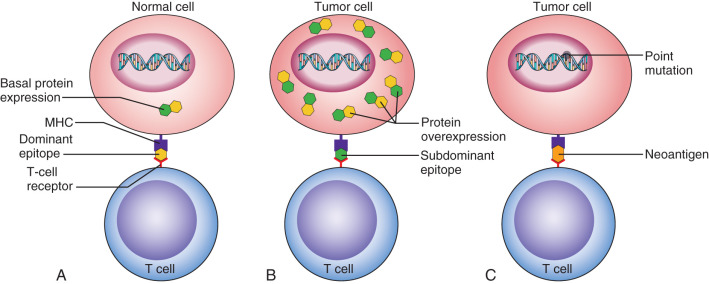
B cells present in the tumor environment indicate an evolving adaptive humoral immune response. B cells develop into plasma cells that release antibodies against an antigen. Autoantibodies against oncogenic proteins can be detected in patients before cancer diagnosis and are indicative of immune recognition of the tumor. Tumor antigen–specific antibodies have been isolated from the peripheral blood of patients and been shown to have potential antitumor activity. Endogenous HER2-specific antibodies can bind the extracellular domain of HER2, inhibit cell signaling, and limit the growth of breast cancer in culture. In a transgenic mouse model of mammary cancer, stimulating antibody immunity against multiple intracellular oncoproteins resulted in complete disease regression. Furthermore, several studies have also shown increased tumor-infiltrating B cells to predict improved outcome in breast cancer. In one gene expression study, the B-cell metagene was associated with improved metastasis-free survival in three independent patient populations totaling 558 patients (first validation cohort n = 286, HR 0.78, 95% CI 0.6–0.8, p = .034; second validation cohort n = 302, HR 0.83, 95% CI 0.7–0.9, p = .021). A second study of 37 breast cancers found a B-cell metagene to be associated with improved distant relapse–free survival in high proliferating hormone receptor positive breast cancer (HR 4.29, 95% CI 2.0–9.0, p = .001) and triple-negative breast cancer (HR 3.34, 95% CI 1.6–7.0, p = .001). In ovarian cancer, improved outcome with tumor-infiltrating B cells have not been associated with increased autoantibodies, but rather their role in presenting tumor antigens to the tumor-infiltrating T cells. Furthermore, tumor-infiltrating B cells may help facilitate Th1 CD4 + T-cell memory. However, the presence of tumor-infiltrating B cells has also been linked with chronic inflammation and immunosuppression promoting a Th2 immune environment and tumor progression. B cells have been found to be elevated in 70% of solid tumors, with some studies associating increased tumor-infiltrating B cells with poor prognosis in several cancer types including breast cancer. Furthermore, the presence of certain autoantibodies has been associated with poor prognosis in breast cancer; for example, in 61 patients with breast cancer, high titers of p53 autoantibodies was associated with a lower 5-year survival than patients with lower titers. Defining the elements of a successful immune response in breast cancer has led to a greater understanding of how to harness immune system cells for diagnostic and prognostic purposes.
Diagnostic Role of Tumor-Associated Autoantibodies
The immune system is capable of responding to abnormal cells that develop early in the malignant transformation of breast cancer. Overexpressed or aberrantly expressed proteins present in breast cancer elicit an immune response and are antigenic. Immune recognition can be assayed by the detection of autoantibodies directed against tumor-associated proteins in the serum of women who have breast cancer compared with non–tumor-bearing controls. Investigations of antibody immunity against single antigens have demonstrated that the presence of such antibodies can be a reflection of oncogenic protein expression in breast cancer. The HER-2/neu (HER2) protein is overexpressed in about 25% of breast cancers and is one of the most commonly studied breast cancer antigens. In an evaluation of 107 recently diagnosed breast cancer patients and 200 women who did not have breast cancer, HER2 autoantibodies could be detected in the serum of 11% of the breast cancer patients but none of control samples. Furthermore, the presence of HER2 autoantibodies correlated with HER2 overexpression as 20% of HER2-positive patients had detectable autoantibodies compared with 5% of HER2-negative breast cancer patients. The likelihood of developing humoral immunity to HER2 has been shown to increase with increasing HER2 protein overexpression in the tumor. An assessment of HER2 antibodies in 104 women with breast cancer demonstrated that 82% of patients with 3+ HER2 expression in their tumors had detectable HER2 autoantibodies compared with 18% of patients with 2+ tumor HER2 expression, and 0% of patients with 1+ tumor HER2 expression as measured by immunohistochemistry (IHC) ( p < .001). Furthermore, there was an increase in the magnitude of the HER2 antibody response with increasing levels of overexpression of the HER2 protein ( p = .02). The p53 protein is found in abundance in the tumors of approximately 50% of breast cancer patients. Studies have shown that autoantibodies to the p53 protein can be detected in up to half of all women with breast cancer. In one analysis of 82 patients with stage I–II breast cancer, 48% of patients had detectable autoantibodies to p53. Similarly, an investigation of 24 nonmetastatic breast cancer patients demonstrated 47% had evidence of p53 autoantibodies at initial diagnosis. The decline in p53 antibody titer paralleled treatment in some patients, whereas an increase in titers preceded relapse in others. A recent evaluation of more than 100 breast cancer patient sera identified autoantibodies to Breast Cancer Susceptibility Gene (BRCA)1 in 19% of serum samples and 37% of samples contained detectable antibody titers to BRCA2 reflecting disease pathogenesis.
There are many tumor-associated proteins that elicit autoantibodies in breast cancer patients, and these few examples demonstrate that breast cancer patients mount antibody responses to the oncogenic proteins that are present their disease. Investigations of antibody immunity to single antigens, however, demonstrate that only a minority of breast cancer patients develops autoantibodies to a particular antigen expressed in their tumor. Common examples are shown in Table 71.1 . Furthermore, each breast cancer subtype is likely to have significant variability in the types of tumor-associated antigens expressed, thus the use of single antigens as diagnostic biomarkers may not be the optimal method of exploiting the humoral immune response for breast cancer detection. Investigators have therefore begun to assess autoantibody panels for breast cancer diagnostics.
Autoantibody | % Patients With Antibody Response | %Tumors Overexpressing Antigen |
---|---|---|
MUC1 | 24 | 38 |
SURVIVIN | 24 | 65 |
P53 | 48 | 43 |
SOX2 | 18 | 33 |
HER2 | 11 | 30 |
Unlike circulating tumor cells or tumor DNA that are typically detected in the presence of bulky tumor, serum autoantibodies can be detected in the presence of small or even preinvasive lesions. Breast cancer–associated autoantibodies have been identified in women with preinvasive breast cancer and have also been detected retrospectively in the serum of women before a breast cancer diagnosis. These observations and the understanding that humoral immunity is a reflection of the malignant antigenic repertoire has led to the exploration of the use of panels of autoantibodies to diagnose breast cancer. The evaluation of panels of tumor-associated autoantibodies has been shown to improve diagnostic sensitivity and specificity over the assessment of individual antigens alone. In a study of 184 advanced-stage breast cancer patients and 134 control individuals, p53 specific antibody immunity could not discriminate between the two populations (area under the curve [AUC] 0.48, p = .538). A combination of the detection of p53 and HER2 specific antibodies increased both sensitivity and specificity to distinguish breast cancer patient sera from controls (AUC 0.61, p = .006). If four antigens (p53, HER2, topoisomerase 2 [TOPO2A], and insulin-like growth factor-binding protein 2 [IGFBP2]) were assessed, breast cancer patient sera could be more readily identified with an AUC 0.63 ( p = .001).
Several studies have now shown the potential for autoantibody panels to detect breast cancer. In one investigation evaluating 137 patients and testing for six antibodies (p53, mucin 1 [MUC1], c-myc, New York Esophageal Cancer-1 [NY-ESO-1], BRCA2, and HER2), 64% of patients with early invasive breast cancer and 45% of patients with ductal carcinoma in situ (DCIS) were positive for at least one of the antigens providing a sensitivity of 85%, despite positivity to single antigens ranging from 3% to 34%. A study of 60 patients with primary breast cancer, 82 patients with DCIS and 93 controls demonstrated the detection of five autoantibodies (peptidylprolyl isomerase A [PPIA], peroxiredoxin 2 [PRDX2], FK506 binding protein 4 [FKBP52], heat shock protein family D [HSP60], and MUC1) could discriminate invasive breast cancer (AUC 0.73, 95% CI 0.60–0.79) and DCIS (AUC 0.80, 95% CI 0.71–0.85) from control sera. A second autoantibody panel could discriminate DCIS sera from samples derived from patients with invasive breast cancer. This study used protein microarray analysis to identify overexpressed immunogenic proteins in DCIS versus invasive breast cancer serum samples. Five autoantibody targets (recombination signal binding protein for immunoglobulin kappa J region [RPBJκ], high mobility group nucleosome binding domain 1 [HMGN1], proline/serine rich coiled coil 1 [PSRC1], cold inducible RNA binding protein [CIRBP], and ethylmalonyl-CoA decarboxylase 1 [ECHDC1]) could distinguish DCIS from invasive breast cancer with AUC 0.794 (95% CI 0.674–0.877). Among patients with DCIS, higher antibody levels against these antigens could differentiate higher grade (grade 3) from low and intermediate grade (grade 1 and 2) DCIS (AUC 0.749, 95% CI 0.581–0.866) as well as identify patients who had inferior relapse-free survival ( p = .011).
Retrospective studies provide evidence that autoantibodies against tumor-associated proteins can be detected well before the diagnosis of breast cancer. Using serum collected from a longitudinal analysis of the Women’s Health Initiative, panels of autoantibodies have been able to differentiate serum samples derived from women who eventually developed breast cancer from samples collected from women who did not develop breast cancer. In an evaluation of a panel of three autoantibodies (HER2, p53, and cyclin B1), samples from women who would develop breast cancer could be distinguished from control serum samples drawn more than 150 days before a cancer diagnosis with AUC 0.60 (95% CI 0.46–0.73). Serum proteomic evaluation of similar serum samples identified numerous autoantibodies associated with the glycolysis and spliceosome pathways that provided AUCs of 0.68 (95% CI 0.59–0.78) and 0.73 (95% CI 0.63–0.82), respectively, to predict those women who would eventually develop breast cancer. These data suggest humoral immunity is operative early in the course of breast cancer and that autoantibody panels may be useful to diagnose breast cancer either as a single modality or in conjunction with other forms of screening such as mammography. Large-scale validation trials are needed to fully assess the utility of the approach.
Prognostic Role of Tumor-Infiltrating Lymphocytes
The cellular immune infiltrate found in the stroma surrounding a breast cancer as well as infiltrating the tumor parenchyma indicates the immune system has recognized the cancer and has mounted an immune response against that tumor. The presence and levels of tumor-infiltrating lymphocytes (TILs) have been shown to predict both prognosis and response to therapy across many tumor types, including breast cancer. Initial studies of the importance of TILs to influence response to chemotherapy in breast cancers have focused on the evaluation of patients undergoing neoadjuvant chemotherapy. In the neoadjuvant setting, the development of a pathologic complete response (pCR) after treatment is used as a surrogate end point for improved survival. An evaluation of TILs in 1058 patients undergoing neoadjuvant chemotherapy for locally advanced breast cancer demonstrated that some patients’ tumors contained greater than 60% lymphocytic infiltrate and were termed “lymphocyte predominant.” Those patients with lymphocyte-predominant breast cancer (LPBC) were more likely to obtain a pCR by multivariate analysis both in a test (42% pCR with LPBC and 3% pCR without LPBC, HR 1.38, 95% CI 1.08–1.78, p = .012) and validation set (40% pCR with LPBC and 7% pCR without LPBC HR 1.21, 95% CI 1.08–1.35, p = .001). Tumors of patients with the triple-negative subtype of breast cancer had the highest percentage of LPBC. This observation has been confirmed in a second trial of 580 patients with triple-negative and HER2-positive disease in which increased pCR was associated with LPBC (HR 1.2, 95% CI 1.16–1.36, p < .001).
The prognostic role of TILs predicting improved overall and disease-free survival in breast cancer has also been evaluated in several large adjuvant chemotherapy trials. In these studies, the beneficial role TILs in predicting improved overall survival was seen only in the triple-negative breast cancer subtype. In one study of node positive patients, enrolled in the Breast International Group (BIG) 02-98 adjuvant study, for every 10% increase in TILs in the tumors of patients with triple-negative breast cancer, there was a 17% decrease in risk of recurrence and 27% decrease risk of death. Triple-negative breast cancer patients whose tumors demonstrated LPBC enjoyed a 5-year disease-free survival of 92% (compared with 62% if there was <10% TILs, p = .018) and a 5-year overall survival of 92% (compared with 71% if there was <10% TILs, p = .036). The improvement in overall and disease-free survival associated with LPBC was not observed for hormone receptor–positive, HER2-negative, or HER2-positive breast cancers. Very similar survival benefits in triple-negative breast cancer have been validated in subsequent large clinical cohorts receiving adjuvant chemotherapy. In one study of 481 triple-negative breast cancer patients who received adjuvant chemotherapy, each 10% increase in stromal TILs was associated with a 14% reduction in risk of recurrence (HR 0.84, 95% CI 0.74–0.95, p = .005) and 19% increase in overall survival (HR 0.79, 95% CI 0.62–0.92, p = .003). These retrospective analyses demonstrate that, for triple-negative breast cancer, the benefit of TILs is not only in tumors with the highest immune infiltrate but that there is an additive effect on prognosis with each increase of 10% of TILs in the tumor. The location of TILs in breast cancer is not as critical as has been reported in other tumor types. Both intraepithelial and stromal TILs have been shown to be prognostic. Because stromal TILs appear to be found in more abundance than intratumoral TILs, many studies only report the number of lymphocytes that are present at the stromal barrier.
The phenotype of the immune response generated is also important in prognosis. Although increased Th1 TILs have consistently predicted improved prognosis in breast cancer, the benefit has been primarily observed in the triple-negative breast cancer subtype. One large study of 1334 breast cancers demonstrated that an increased level of CD8 + tumor T-cell infiltrate predicted improved overall survival across all breast cancers (HR 0.55, 95% CI 0.39–0.78, p < .001). When the subtypes were evaluated separately, however, breast cancer–specific and disease-free survival benefit was only observed for triple-negative tumors. In one small study of 102 HER2-positive breast tumors, presence of the Th1 transcription factor Tbox transcription factor 21 (Tbet) in TILs predicted a survival benefit when increased after trastuzumab therapy, but this marker has not been consistently evaluated in larger data sets. The presence of FOXP3 + Treg TILs is associated with an unfavorable prognosis.
An evaluation of 237 breast cancers revealed increased Treg tumor infiltrates, defined by FOXP3 expression on the lymphocytes, was associated with inferior progression-free survival (HR 2.15, 95% CI 1.10–4.17, p = .03) and overall survival (HR 2.4, 95% CI 1.07–5.38, p = .03) in hormone receptor–positive tumors. This result has been confirmed in a second study of 1270 breast cancers, which showed that FOXP3 + TIL infiltrate greater than the median predicted worse progression-free (HR 1.90, 95% CI 1.12–3.22, p = .018) and overall (HR 1.53, 95% CI 1.04–2.25, p = .029) survival. Data suggest that subtype-specific differences can affect tumor immune recognition and may affect the composition of TILs. The triple-negative subtype is most likely to contain the greatest amount of lymphocytic immune infiltrate of any of the subtypes. Hormone receptor–positive tumors tend to have the fewest tumors with LPBC and the greatest number of patients with no lymphocytic infiltrate. However, patients with HER2-positive tumors have similar levels of TILs to triple-negative breast cancer and yet have not experienced the same survival benefit seen with increasing levels of TILs in triple-negative breast cancers. Future studies of TILs should evaluate breast cancer subtypes separately when examining the effect of specific tumor-infiltrating immune cell populations on prognosis.
More work is needed to determine whether an evaluation of TILs should be added to the standard staging system for breast cancer. An international consortium has developed recommendations for the enumeration of breast cancer TILs, which include the locations of TILs to be examined, how to quantitate TILs, the number of tissue sections to be evaluated, and the instruction that TILs be assessed as a continuous parameter. In the future, the evaluation of the existing tumor immune infiltrate at diagnosis may provide important prognostic information or even act as a guide as to whether a patient will benefit from the addition of immune therapy to standard treatment regimens.
Immunologic Effects of Standard Breast Cancer Therapies
Breast cancer treatments with immunologically mediated mechanisms of action are currently the standard of care for HER2-positive breast cancer. Monoclonal antibodies induce antibody-dependent cell-mediated cytotoxicity (ADCC) that results in the activation of NK T cells, macrophages, and dendritic cells. Activation of cells of the innate immune system leads to the secretion of Th1 cytokines, enhanced antigen processing, and presentation of endogenous tumor antigens to T cells eliciting an adaptive immune response. These immune effects are evident in studies of patients receiving trastuzumab. In an analysis of 38 HER2-positive patients, the use of trastuzumab resulted in increased NK T cells both infiltrating the tumor ( p = .0532) and in the surrounding tissue ( p = .0097) compared with the patients not receiving the monoclonal antibody. Furthermore, in a similar evaluation of 26 metastatic breast cancer patients, increased NK T-cell activity was associated with increased progression-free survival ( p = .024). Patients treated with trastuzumab also demonstrate increased TILs compared with those not receiving monoclonal antibody therapy. Patients treated with trastuzumab (n = 38) increased both intratumoral CD4 + T-cell infiltrate ( p = .02) and stromal CD8 + T-cell infiltrate ( p = .05) compared with patients not treated with trastuzumab (n = 23). Furthermore, CD8 + T-cell infiltration predicted pCR in the trastuzumab-treated arm ( p = .05). There is increasing evidence that trastuzumab therapy results in the development of HER2-specific adaptive immunity. In 40 patients with stage I–III HER2-positive disease receiving adjuvant chemotherapy plus trastuzumab, patients with pCR (n = 16) had increased levels of circulating IFN-γ secreting HER2 specific CD4 + T cells compared with those without pCR (98% vs. 33%, p = .0002). In addition, the HER2-specific Th1 response was independently associated with pCR by multivariate analysis (odds ratio [OR] 8.82, 95% CI 1.50–51.83, p = .016). These data indicate the immune response elicited by trastuzumab potentially improves clinical outcome. Early studies suggest that a quantitative assessment of HER2 specific Th1 immunity may be a marker of the therapeutic efficacy of the drug.
Therapeutic chemotherapy regimens have been selected for the cytotoxic effect of specific drug combinations against rapidly dividing cancer cells. Other cell types with high turnover rates are also adversely affected by chemotherapy, such as cells in the gastrointestinal tract and hematopoietic cells. The ability of chemotherapy to significantly reduce numbers of immune cells resulting in immune suppression in some cases has led to the wide-held belief that cytotoxic chemotherapy generally depresses immunity. There is emerging evidence that certain forms of chemotherapy may actually increase immune recognition and aid in the development of effective antitumor immunity through specific immunologic mechanisms ( Table 71.2 ). For example, the immune system is variably triggered by different forms of cell death. Normal apoptotic cell turnover induces immune tolerance whereas infection and inflammation triggers cellular stress receptors that induce immune activation. These stress signals are recognized through toll-like receptors (TLR) and other pattern recognition receptors in dendritic cells causing dendritic cell activation, maturation, antigen processing, and T-cell activation. Some chemotherapeutic agents have been shown to trigger immune recognition of the tumor by induction of specific proteins released during cell death. Doxorubicin, for example, induces the secretion of a protein called high-mobility-group box 1 (HMGB1) by dying cancer cells. HMGB1 binds to TLR-4 on dendritic cells resulting in the secretion of IFN-γ, antigen presentation, and activation of T cells. The resulting adaptive immune response may be a major mechanism of effective clinical responses after doxorubicin treatment. A TLR-4 genetic polymorphism, Asp299Gly, has been shown to decrease the binding of HMGB1 thereby decreasing IFN-γ secretion by 50% ( p < .05) in in vitro assays. In an evaluation of 280 breast cancer patients treated with adjuvant anthracycline, 40% of the patients carrying the TLR-4 Asp299Gly polymorphism developed metastatic disease in 5 years compared with 27% of patients that did not bear the polymorphism (RR 1.53, 95% CI 1.1–3.59, p = .03). Similarly, preapoptotic release of calreticulin induces immunogenic cell death not only for anthracyclines but for oxaliplatin and cisplatin as well.
Chemotherapy | Immunologic Effects |
---|---|
Doxorubicin | Increases antitumor immune recognition through TLR4-like stress signals |
Taxanes | Increases antitumor CD4 + tumor infiltrate by increasing expression of Th1 cytokines and decreases intratumoral Th2 regulatory T cells |
Cyclophosphamide | Decreases peripheral circulating Th2 regulatory T cells without affecting circulating Th1 immune cells at low doses |
Gemcitabine | Increases MHC class 1 expression on tumors |
Cisplatin/carboplatin | Increases MHC class 1 expression and decreases MDSC and Th2 regulatory T cells in the tumor |
Trastuzumab/pertuzumab | Increases ADCC NK T-cell recruitment to tumors by recognition of FC portions of the targeted antibody therapy |
Specific chemotherapy use is associated with an increased influx of immune system cells into the tumor. Anthracyclines are cornerstones of breast cancer neoadjuvant and adjuvant chemotherapy and, in preclinical mouse models, have been shown to increase CD8 + tumor T-cell infiltrates. When evaluating gene expression panels from breast cancer patients, 1062 patients who did not receive chemotherapy and 114 patients who received anthracycline monotherapy, the CD8 (HR 0.72, 95% CI 0.59–0.82, p = .005) and IFN-γ (HR 0.56, 95% CI 0.56–0.89, p = .016) tumor expression predicted improved pCR only in the patients who had been treated with an anthracycline. This study suggests that some of the clinical efficiency of anthracycline-based chemotherapy may be due to the enhanced immune infiltration. Similar results have been observed when examining CD8 + tumor T-cell infiltrate by immunohistochemistry in 368 triple-negative breast cancer patients were improved from 31% to 74% (95% CI 2.49–16.08, p < .0001) when patients were treated with anthracyclines and demonstrated CD8 + TILs. However, pCR was not dependent on tumor-immune infiltrate in non–anthracycline-containing regimens.
Some standard chemotherapies have the potential to induce more effective immunity by limiting the outgrowth of cells that suppress the adaptive immune response. This inhibitory effect may occur at much lower doses than what is used for cytoreduction. Very low doses of paclitaxel have recently been shown to decrease the accumulation of MDSC that limit T-cell activation in a preclinical melanoma model. The use of low-dose paclitaxel reduced the chronic inflammation seen with growing malignancy and enhanced CD8 + T-cell effector functions. Similarly, low-dose cyclophosphamide can deplete regulatory T cells without affecting levels of effector T cells. In a study of 12 metastatic breast cancer patients, low-dose oral cyclophosphamide (50 mg daily) resulted in a short-term 40% reduction in peripheral Tregs ( p = .002). Depletion of Tregs was associated with an increase in circulating tumor-specific T cells ( p = .03) that remained elevated throughout treatment and were associated with both disease stabilization ( p = .03) and overall survival ( p = .027). The current evidence suggests that common chemotherapies may affect the tumor immune environment in different ways and that many of the cornerstone drugs used in breast cancer treatment are associated with enhancing immunity which may be linked to improved clinical outcomes.
Breast Cancer Vaccines
The goal of a breast cancer vaccine is to educate the patient’s immune system to recognize the cancer and elicit an antitumor immune response capable of destroying any current tumor and providing durable immune surveillance to prevent disease recurrence. Most of the early studies of breast cancer vaccines focused on glycosylated antigens that were ubiquitously expressed on most breast cancers. Antigens such as MUC1 or sialyl-TN were constructed as vaccines using a variety of adjuvants and studied for immunogenicity and clinical efficacy in a number of settings in breast cancer but the results of phase III studies were not encouraging. A double-blind randomized phase III trial of sialyl-TN with keyhole limpet hemocyanin (KLH) as an adjuvant versus KLH alone enrolled 1028 women with metastatic breast cancer. There was no difference in time to progression or overall survival between vaccinated and nonvaccinated patients. A pilot phase III study of a mannan-MUC1 vaccine construct demonstrated that although the vaccine could generate antibody immunity in majority of patients vaccinated, few individuals developed T-cell immunity potentially needed for tumor eradication. These two studies underscore the pitfalls of early vaccines: the vaccines were poorly immunogenic and the glycosylated antigens were more likely to induce antibodies of the immunoglobulin (Ig)M subtype that are short-lived and incapable of further activating immunity. Newer approaches to vaccinating with carbohydrate-based vaccines are focusing on methods to increase the conversion of IgM to IgG antibodies, which are more potent in triggering innate immune cells, leading to cross-priming for tumor-specific T cells.
Most current phase II and III vaccine trials are targeting nonmutated self-proteins that are immunogenic and tumor associated. Many of these proteins are markedly overexpressed in cancer compared with tissues that express basal levels of these proteins. Studies in autoimmune disease have demonstrated that overexpression of self-proteins results in a unique MHC-peptide repertoire display associated with unmasking of subdominant epitopes that have not been previously available for immune recognition. Thus, overexpression of cancer-associated proteins can allow the T-cell response to be selective to the cancer tissues because of the higher expression of subdominant epitopes. One of the most commonly studied vaccine targets in breast cancer is the HER2 protein. The degree of HER2 overexpression can trigger both increasing antibody and T-cell responses. Several HER2 vaccines have been shown to be safe, immunogenic, and have evidence of clinical response in early trials. In a study of 22 HER2-positive metastatic breast cancer patients receiving a peptide-based HER2 vaccine targeting class II MHC binding epitopes with granulocyte macrophage colony stimulation factor as an adjuvant, 74% of the patients developed new or augmented HER2-specific Th1 immunity. Both intramolecular and intermolecular epitope spreading developed, with 71% of evaluated patients developing augmented Th1 immunity to oncogenic proteins other than HER2. In this trial, the median progression-free survival was 33% at 3 years with overall survival of 86% at 4 years. Increased HER2 specific T-cell responses trended to improved overall survival ( p = .08) suggesting that development of immunity may be a factor for the improved outcomes. HER2-specific vaccines have been explored in the adjuvant setting as well in patients with early-stage breast cancer. In a pooled analysis of phase I/II studies that evaluated immunization with a short peptide vaccine targeting a HER2 epitope that has been shown to induce cytotoxic T cells in human leukocyte antigen (HLA) A2/A2 positive patients (E75), a 24-month landmark analysis demonstrated that disease-free survival was 94.3% in the vaccinated group and 86.8% in the control group ( p = .08). In a subset analysis, the beneficial outcome was associated with receiving the optimal dose of the vaccine, having a grade 1 or 2 tumor, and the presence of positive lymph nodes. Furthermore, those patients with the lowest levels of HER2 expression on their tumors as measured by IHC (1–2+) appeared to have a more favorable outcome than those who demonstrated 3+ overexpression. Whether this observation was due to the more aggressive nature of tumors that highly express HER2 was not explored.
The E75 vaccine, now called NeuVax, is in the final stages of a phase III study to prevent disease recurrence. HER2 vaccination has also been evaluated for the treatment of DCIS. Patients with DCIS were vaccinated with a HER2 peptide-based vaccine. The majority of patients vaccinated developed significant levels of HER2-specific CD4 + and CD8 + T cells. Seven of 11 evaluable patients also showed decreased HER2 expression in the surgical tumor specimen, often with measurable decreases in HER2 expression in residual DCIS. These early data, using similar vaccines against a single antigen, suggest a potential role for vaccination in all stage of HER2-positive breast cancer to improve clinical outcomes.
Other self-antigens have also been used in breast cancer vaccination. Mammaglobin-A is overexpressed in 80% of hormone receptor–positive breast cancer, and a trial was performed using a DNA vaccine that stimulated antigen-specific CD8 + T cells in 57% of patients (8 of 14) with metastatic breast cancer. Vaccinated patients had an improved progression-free survival at 6 months of 53% compared with 33% ( p = .011) in patients who were unable to be vaccinated based on HLA phenotype but otherwise met trial enrollment criteria. Studies have also been performed using vaccines to enhance chemotherapy. The PANVAC vaccine is a poxvirus vaccine that expresses carcinoembryonic antigen (CEA) and MUC1 as epitopes, along with costimulatory molecules B7.1, intracellular adhesion molecule 1 (ICAM1), and lymphocyte associated antigen-3 (LFA-3). A phase II study of 48 patients with metastatic breast cancer evaluated docetaxel with and without PANVAC. There was a trend toward improved progression-free survival of 7.9 months compared with 3.9 months (HR 0.65, 95% CI 0.03–1.14, p = .09) in patients who received both vaccine and docetaxel. These studies demonstrate newer methods of vaccination are effective in eliciting cellular immunity and that vaccines may be potentiated when combined with chemotherapy. Finally, there is much work being done to define patient-specific mutations that may encode neo-antigens that may have never been exposed to the immune system. Mutated proteins that occur in cancer may truly be cancer specific because mutations would not be present in normal tissues. Although mutations may be identified in a patient’s tumor, whether those mutations can be processed and presented in the MHC for T-cell recognition is unknown. The development of mutation specific vaccines for the treatment of breast cancer is an area of intense investigation.
Data from all breast cancer vaccine clinical trials to date suggest vaccines are safe and well tolerated with primarily grade 1 and 2 toxicities and no evidence clinically significant autoimmune toxicities. HER2 targeting vaccine studies show the majority of toxicities were grade 1–2 injection site reactions. Furthermore, none of the HER2 vaccines tested have had evidence of the cardiac toxicity seen with other HER2 directed therapies such as trastuzumab. The Mammaglobin-A vaccine similarly demonstrated a favorable toxicity profile with no grade 3 toxicities and mainly infusion site grade 1 toxicities. Low-risk toxicity profiles have been consistent across published breast cancer vaccine studies and will be particularly beneficial when combining vaccine therapies with other typically less well-tolerated therapies. Vaccination as monotherapy tends to be less effective in patients with a large tumor burden as advanced breast cancer display an immune suppressive tumor environment that can serve as an antigen sink, drawing the vaccine-activated immune cells to the tumor but then inactivating them and preventing effective function. Therefore, vaccine therapy may show the most benefit in the adjuvant setting where there is no evidence of cancer and therefore the immune response mounted to the vaccine can acutely target minimal residual disease. For patients with more advanced disease, vaccine therapy may be more effective if used in combination with other therapies such as chemotherapy, adoptive T-cell therapy, or checkpoint inhibitor monoclonal antibody therapy.
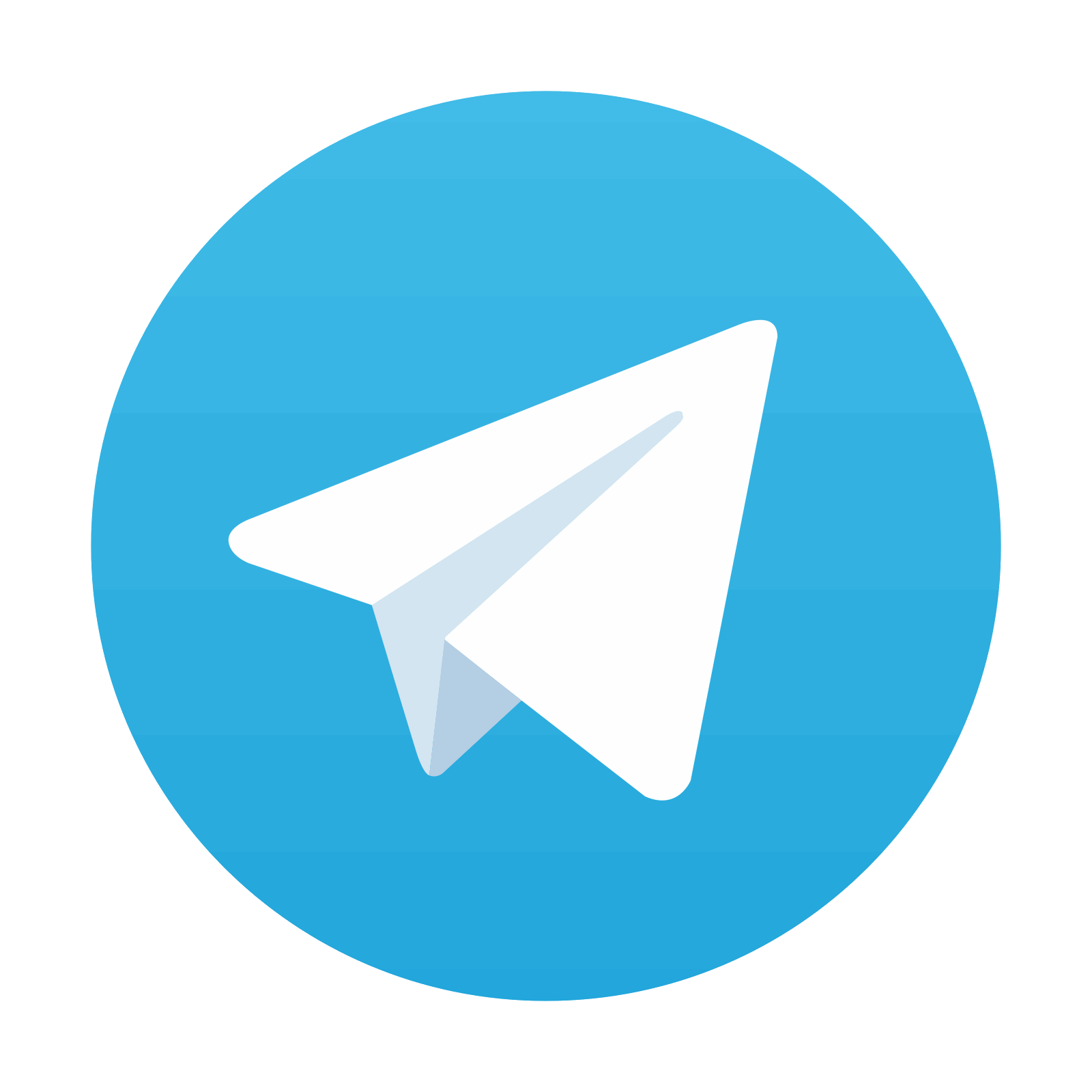
Stay updated, free articles. Join our Telegram channel

Full access? Get Clinical Tree
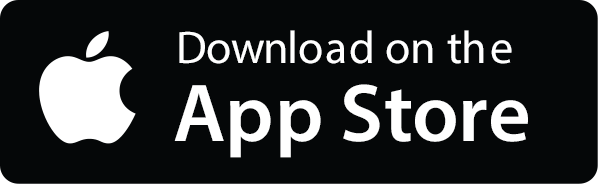
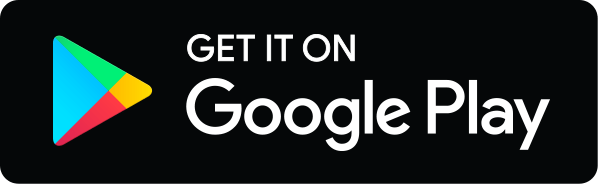