Immunogens and Antigens
Introduction
Immune responses arise as a result of exposure to foreign stimuli. The compound that evokes the response is referred to either as antigen or as immunogen. The distinction between these terms is functional. An antigen is any agent capable of binding specifically to components of the immune system, such as the B cell receptor (BCR) on B lymphocytes and soluble antibodies. By contrast, an immunogen is any agent capable of inducing an immune response and is therefore immunogenic. The distinction between the terms is necessary because there are many compounds that are incapable of inducing an immune response, yet they are capable of binding with components of the immune system that have been induced specifically against them. Thus all immunogens are antigens, but not all antigens are immunogens. This difference becomes obvious in the case of low molecular weight compounds, a group of substances that includes many antibiotics and drugs. By themselves, these compounds are incapable of inducing an immune response but when they are coupled with much larger entities, such as proteins, the resultant conjugate induces an immune response that is directed against various parts of the conjugate, including the low molecular weight compound. When manipulated in this manner, the low molecular weight compound is referred to as a hapten (from the Greek hapten, which means “to grasp”); the high molecular weight compound to which the hapten is conjugated is referred to as a carrier. Thus a hapten is a compound that, by itself, is incapable of inducing an immune response but against which an immune response can be induced by immunization with the hapten conjugated to a carrier.
Immune responses have been demonstrated against all the known biochemical families of compounds, including carbohydrates, lipids, proteins, and nucleic acids. Similarly, immune responses to drugs, antibiotics, food additives, cosmetics, and small synthetic peptides can also be induced, but only when these are coupled to a carrier. In this chapter, we discuss the major attributes of compounds that render them antigenic and immunogenic.
Requirements for Immunogenicity
A substance must possess the following characteristics to be immunogenic: (1) foreignness; (2) high molecular weight; (3) chemical complexity; and, in most cases, (4) degradability and interaction with host major histocompatibility complex (MHC) molecules.
Foreignness
Animals normally do not respond immunologically to self. Thus, for example, if a rabbit is injected with its own serum albumin, it will not mount an immune response; it recognizes the albumin as self. By contrast, if rabbit serum albumin is injected into a guinea pig, the guinea pig recognizes the rabbit serum albumin as foreign and mounts an immune response against it. To prove that the rabbit, which did not respond to its own albumin, is immunologically competent, it can be injected with guinea pig albumin. The competent rabbit will mount an immune response to guinea pig serum albumin because it recognizes the substance as foreign. Thus, the first requirement for a compound to be immunogenic is foreignness. The more foreign the substance, the more immunogenic it is. In general, compounds that are part of self are not immunogenic to that individual. However, there are exceptional cases in which an individual mounts an immune response against his or her own tissues. This condition is termed autoimmunity (see Chapter 3).
High Molecular Weight
The second feature that determines whether a compound is immunogenic is its molecular weight. In general, small compounds that have a molecular weight of less than 1,000 Da (e.g., penicillin, progesterone, aspirin) are not immunogenic; those of molecular weights between 1,000 and 6,000 Da (e.g., insulin, adrenocorticotropic hormone [ACTH]) may or may not be immunogenic; and those of molecular weights greater than 6,000 Da (e.g., albumin, tetanus toxin) are generally immunogenic. In short, relatively small substances have decreased immunogenicity whereas large substances have increased immunogenicity.
Chemical Complexity
The third characteristic necessary for a compound to be immunogenic is a certain degree of physicochemical complexity. Thus, for example, simple molecules such as homopolymers of amino acids (e.g., a polymer of lysine with a molecular weight of 30,000 Da) are seldom good immunogens. Similarly, a homopolymer of poly-γ-D-glutamic acid (the capsular material of Bacillus anthracis) with a molecular weight of 50,000 Da is not immunogenic. The absence of immunogenicity is because these compounds, although of high molecular weight, are not sufficiently chemically complex. However, if the complexity is increased by attaching various moieties (such as dinitrophenol or other low molecular weight compounds), which, by themselves, are not immunogenic, to the epsilon amino group of polylysine, the entire macromolecule becomes immunogenic. The resulting immune response is directed not only against the coupled low molecular weight compounds but also against the high molecular weight homopolymer. In general, an increase in the chemical complexity of a compound is accompanied by an increase in its immunogenicity. Thus copolymers of several amino acids, such as polyglutamic, alanine, and lysine (poly-GAT), tend to be highly immunogenic.
Because many immunogens are proteins, it is important to understand the structural features of these molecules. Each of the four levels of protein structure contributes to the molecule’s immunogenicity. The acquired immune response recognizes many structural features and chemical properties of compounds. For example, antibodies can recognize various structural features of a protein, such as its primary structure (the amino acid sequence), secondary structures (the structure of the backbone of the polypeptide chain, such as an α-helix or β-pleated sheet), and tertiary structures (formed by the three-dimensional configuration of the protein, which is conferred by the folding of the polypeptide chain and held by disulfide bridges, hydrogen bonds, hydrophobic interactions, etc.) (Figure 4.1). They can also recognize quaternary structures (formed by the juxtaposition of separate parts, if the molecule is composed of more than one protein subunit) (Figure 4.2).
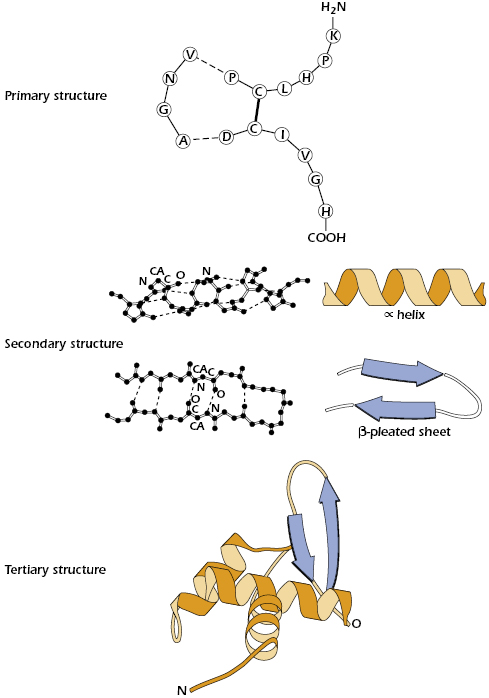
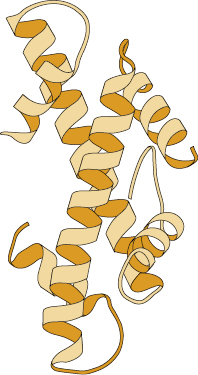
Degradability
In contrast to B cells, in order for antigens to activate T cells to stimulate immune responses, interactions with MHC molecules expressed on antigen-presenting cells (APCs) must occur (see Chapter 10). APCs must first degrade the antigen through a process known as antigen processing (enzymatic degradation of antigen) before they can express antigenic epitopes on their surface. Epitopes are also known as antigenic determinants. They are the part of an antigen that is recognized by the immune system and are the smallest unit of antigen that is capable of binding with antibodies and T-cell receptors. Once degraded and noncovalently bound to MHC, these epitopes stimulate the activation and clonal expansion of antigen-specific effector T cells. A protein antigen’s susceptibility to enzymatic degradation largely depends on two properties: (1) it has to be sufficiently stable so that it can reach the site of interaction with B cells or T cells necessary for the immune response, and (2) the substance must be susceptible to partial enzymatic degradation that takes place during antigen processing by APCs. Peptides composed of D-amino acids, which are resistant to enzymatic degradation, are not immunogenic, whereas their L-isomers are susceptible to enzymes and are immunogenic. By contrast, carbohydrates are not processed or presented and are thus unable to activate T cells, although they can directly activate B cells.
In general, a substance must have all four of these characteristics to be immunogenic; it must be foreign to the individual, have a relatively high molecular weight, possess a certain degree of chemical complexity, and be degradable.
Haptens
As noted earlier, substances called haptens fail to induce immune responses in their native form because of their low molecular weight and their chemical simplicity. These compounds are not immunogenic unless they are conjugated to high molecular weight, physiochemically complex carriers. Thus an immune response can be evoked to thousands of chemical compounds—those of high molecular weight and those of low molecular weight, provided the latter are conjugated to high molecular weight complex carriers.
Further Requirements for Immunogenicity
Several other factors play roles in determining whether a substance is immunogenic. The genetic makeup (genotype) of the immunized individual plays an important role in determining whether a given substance will stimulate an immune response. Genetic control of immune responsiveness is largely controlled by genes mapping within the MHC. Another factor that plays a crucial role in the immunogenicity of substances relates to the B- and T-cell repertoires of an individual. Acquired immune responses are triggered following the binding of antigenic epitopes to antigen-specific receptors on B and T lymphocytes. If an individual lacks a particular clone of lymphocytes consisting of cells that bear the identical antigen-specific receptor needed to respond to the stimulus, an immune response to that antigenic epitope will not take place. Finally, practical issues such as the dosage and route of administration of antigens play a role in determining whether the substance is immunogenic.
Insufficient doses of antigen may not stimulate an immune response either because the amount administered fails to activate enough lymphocytes or because such a dose renders the responding cells unresponsive. The latter phenomenon induces a state of tolerance to that antigen (see Chapter 13). Besides the need to administer a threshold amount of antigen to induce an immune response, the number of doses administered also affects the outcome of the immune response generated. As discussed below, repeated administration of antigen is required to stimulate a strong immune response.
Finally, the route of administration can affect the outcome of the immunization strategy, because this determines which organs and cell populations will be involved in the response. Antigens administered via the most common route—namely, subcutaneously, generally elicit the strongest immune responses. This is due to their uptake, processing, and presentation to effector cells by Langerhans cells present in the skin, which are among the most potent APCs. Responses to subcutaneously administered antigens take place in the lymph nodes draining the injection site. Intravenously administered antigens are carried first to the spleen, where they can either induce immune unresponsiveness or tolerance, or, if presented by APCs, generate an immune response. Orally administered antigens (gastrointestinal route) elicit local antibody responses within the intestinal lamina propria but often produce a systemic state of tolerance (antigen unresponsiveness) (see Chapter 13 for a detailed discussion about tolerance). Finally, administration of antigens via the respiratory tract (intranasal route) often elicits allergic responses (see Chapter 15).
Since immune responses depend on multiple cellular interactions, the type and extent of the immune response is affected by the cells populating the organ to which the antigen is ultimately delivered. The stringent requirements given above constitute a portion of the delicate control mechanisms, expanded and elaborated in subsequent chapters, which, on one hand, trigger the adaptive immune response and, on the other hand, protect the individual from responding to substances in cases where such responses are detrimental.
Primary and Secondary Responses
The first exposure of an individual to an immunogen is referred to as the primary immunization, which generates a primary response. As we shall see in subsequent chapters, many events take place during this primary immunization: cells process antigen, triggering antigen-specific lymphocytes to proliferate and differentiate; T-lymphocyte subsets interact with other subsets and induce the latter to differentiate into T lymphocytes with specialized function; T lymphocytes also interact with B lymphocytes, inducing them to synthesize and secrete antibodies.
A second exposure to the same immunogen results in a secondary response. This may occur after the response to the first immune event has leveled off or has totally subsided (within weeks or even years). The secondary response differs from the primary response in many respects. Most notably and biologically relevant is the much quicker onset and the much higher magnitude of the response. In a sense, this secondary (and subsequent) exposure behaves as if the body remembered that it had been previously exposed to that same immunogen. In fact, secondary and subsequent responses exploit the expanded number of antigen-specific lymphocytes generated in response to the primary immune response. Thus the increased arsenal of responding lymphocytes accounts, in part, for the magnitude of the response observed. The secondary response is also called the memory or anamnestic response, and the B and T lymphocytes that participate in the memory response are termed memory cells.
Antigenicity and Antigen-Binding Site
An immune response induced by an antigen generates antibodies or lymphocytes that react specifically with the antigen. The antigen-binding site of an antibody or a receptor on a lymphocyte has a unique structure that allows a complementary fit to some structural aspect of the specific antigen. The portion of the immunoglobulin that specifically binds to the antigenic determinant or epitope is concentrated in several hypervariable regions of the molecule, which form the complementarity-determining region (CDR). Additional structural features of the immunoglobulin molecule are described in Chapter 5.
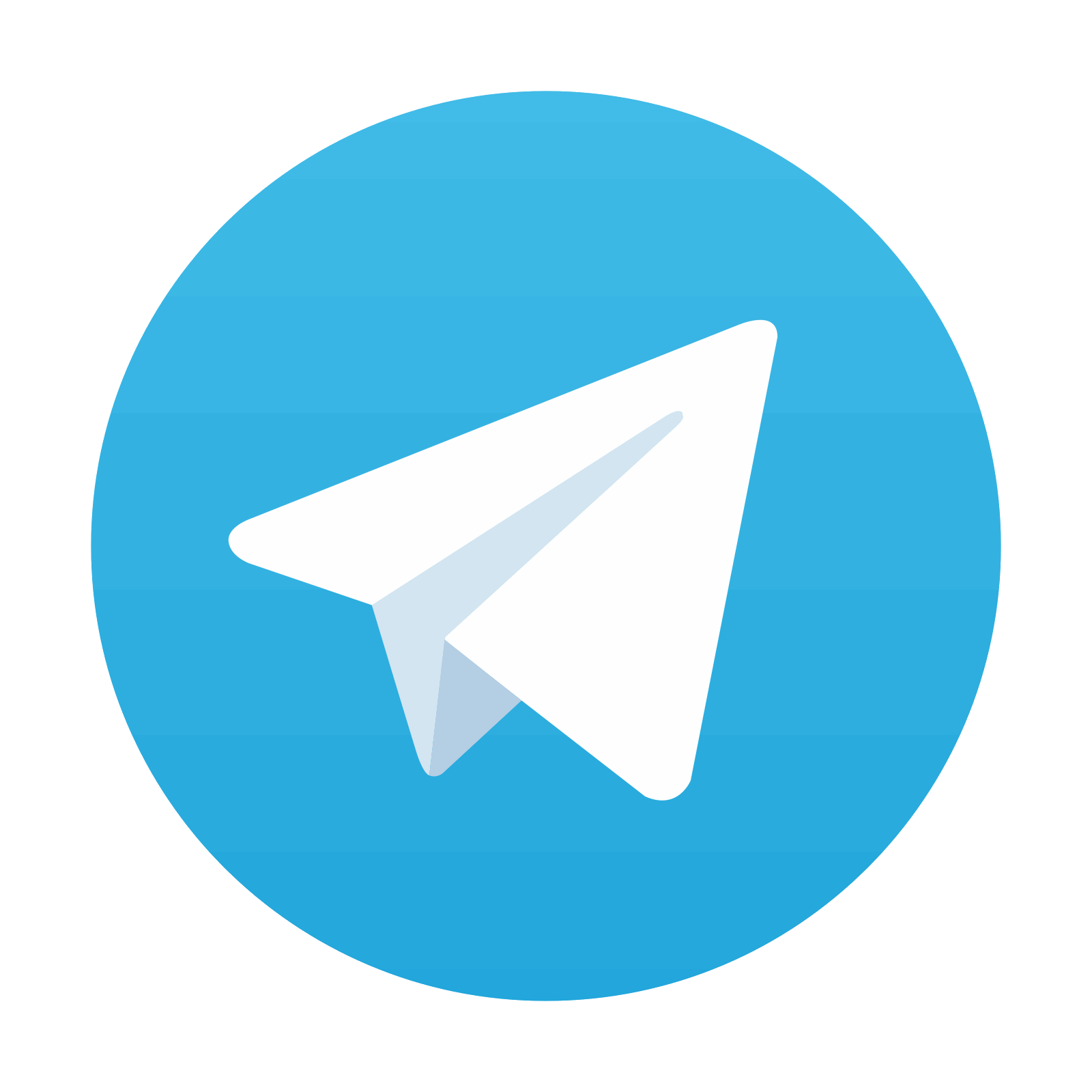
Stay updated, free articles. Join our Telegram channel

Full access? Get Clinical Tree
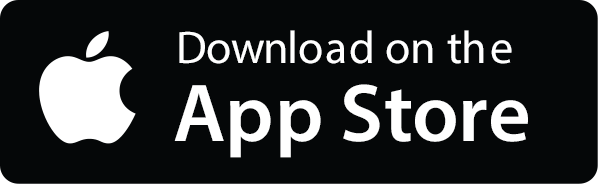
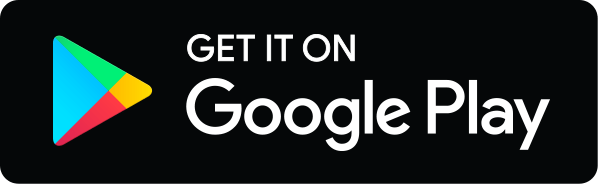