Transplantation
Introduction
The immune system has evolved as a way of discriminating between self and nonself. Once foreignness has been established, the immune system proceeds toward its ultimate goal of destroying the foreign material, be it a microorganism or its product, a substance present in the environment, or a tumor cell. Chapter 20 will describe immune mechanisms associated with the latter.
The same self/nonself discriminating power of the immune system is undesirable in therapeutic settings such as the transplantation of cells, tissues, or organs from one individual to another. Prior to the advent of effective immunosuppressive therapies, organ transplants uniformly culminated in the phenomenon of graft rejection, with one notable exception, namely, blood transfusions. Blood transfusions represent the earliest and most successful cell-based “transplants,” and they continue to be the most common of all transplants. The reason for this success is due to the fact that red blood cells (RBCs) do not express major histocompatibility complex (MHC) antigens, and they express only a limited number of different types of the major RBC antigens, most importantly, the ABO and Rh blood group antigens. Therefore, it is relatively easy to match the RBCs of the donor and recipient. Matching prevents rapid antibody-mediated destruction of donor RBC. By contrast, the MHC antigens expressed on other cells, tissues, and organs are highly polymorphic in the population, so matching donor and recipient is extremely difficult.
Our current understanding of cellular and molecular mechanisms associated with graft rejection and, as noted above, effective immunosuppressive therapies, have made transplantation of various cells, tissues, and organs for therapeutic purposes very commonplace (Table 19.1). For example, over 10,000 kidneys are transplanted annually worldwide with a high degree of success. Transplantations of heart, lungs, corneas, liver, and bone marrow that were considered spectacular and were widely publicized as recently as 25 years ago, have now become commonplace. Although rejection episodes have been significantly reduced due to the use of immunosuppressive therapies, they have not been eliminated. Thus, transplantation immunology continues to be a major area of research.
Table 19.1. Transplantation of Specific Organs and Tissues
Organ/Tissue | Clinical Uses | Comments |
---|---|---|
Skin | Burns, chronic wounds, diabetic ulcers, venous ulcers | Commonly autologous grafts; increasing use of artificial skin consisting of stromal elements and cultured cells of allogeneic or xenogeneic origin |
Kidney | End-stage renal failure | Graft survival now exceeds 85% at 1 year even with organs from unrelated donors |
Liver | Hepatoma and biliary atresia | Successful in about two thirds of recipients at 1 year |
Heart | Cardiac failure | Survival rates in excess of 80% at 1 year |
Lung | Advanced pulmonary or cardiopulmonary diseases | Sometimes performed together with heart transplantation |
Bone marrow | Incurable leukemias and lymphomas, congenital immunodeficiency diseases | Risk of graft-versus-host disease is a unique feature of bone marrow transplantation; increasingly, transplantation of hematopoietic stem cells being used |
Cornea | Blindness | HLA matching is not advantageous because this is a “privileged” site that normally lacks lymphatic drainage |
Pancreas | Diabetes mellitus | Pancreas and kidney transplantation are sometimes performed together; success rates are approaching that seen with kidney transplants |
Relationship between Donor and Recipient
Before we discuss the immunologic mechanisms associated with graft rejection, it is important to understand the various gradations in relationships of transplantation from donor to recipient. These are shown in Figure 19.1 and are described below.
- An autograft is a graft or transplant from one area to another on the same individual such as would occur in the transplantation of normal skin from one area of an individual to a burned area of the same individual. The graft is recognized as autochthonous or autologous (self), and no immune response is induced against it. Barring technical difficulties in the transplantation process, the graft will survive or take in its new location.
- An isograft or syngraft is a graft or transplant of cells, tissue, or an organ from one individual to another individual who is syngeneic (genetically identical) to the donor. An example of an isograft is the transplantation of a kidney from one identical (homozygotic) twin to the other. As in the case of an autograft, the recipient who is genetically identical with regard to the donor MHC and all other loci, recognizes the donor’s tissue as “self” and does not mount an immune response against it. The two individuals (i.e., donor and recipient) are described as histocompatible.
- An allograft is a graft, or transplant, from one individual to an MHC-disparate individual of the same species. Because of the high degree of MHC polymorphism within a given outbred species, this allogeneic transplant will result in rejection of the grafted foreign tissue. The donor and recipient, in this case, are nonhistocompatible or histoincompatible.
- A xenograft is a graft between a donor and a recipient from different species. The transplant is recognized as foreign, and the immune response mounted against it will destroy or reject the graft. Donor and recipient are again histoincompatible.
- An isograft or syngraft is a graft or transplant of cells, tissue, or an organ from one individual to another individual who is syngeneic (genetically identical) to the donor. An example of an isograft is the transplantation of a kidney from one identical (homozygotic) twin to the other. As in the case of an autograft, the recipient who is genetically identical with regard to the donor MHC and all other loci, recognizes the donor’s tissue as “self” and does not mount an immune response against it. The two individuals (i.e., donor and recipient) are described as histocompatible.
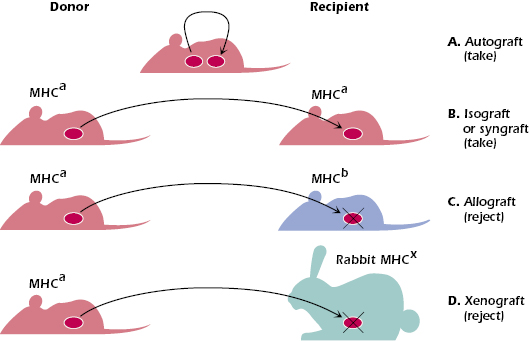
Immune Mechanisms are Responsible for Allograft Rejection
The most direct evidence that the immune response is involved in graft rejection is provided by experiments in which skin is transplanted from one individual to a genetically different individual of the same species, namely, between allogeneic donor and recipient. Skin from a mouse with black hair transplanted onto the back of an MHC-disparate white-haired mouse appears normal for 1 or 2 weeks. However, after approximately 2 weeks, the skin allograft begins to be rejected, and is completely sloughed off within a few days. This process is called first-set rejection. If, after this rejection, the recipient is transplanted with another piece of skin from the same initial donor, the graft is rejected within 6–8 days. This accelerated rejection is termed a second-set rejection. By contrast, grafting skin from a different MHC-disparate mouse strain will result in rejection at a rate similar to that of the initial graft, with so-called first-set kinetics. Thus, second-set rejection is an expression of specific immunologic memory for antigens expressed by the graft. The participation of CD4+ and CD8+ T cells in the rejection response can be shown by transferring these cells from an individual sensitized to an allograft into a normal syngeneic recipient. If the second recipient is transplanted with the same allograft that was used on the original T-cell donor, a second-set rejection ensues. This establishes that T cells primed in the initial grafting mediate the accelerated rejection in the second host. However, antibodies can also contribute to the destruction of grafted tissue in second-set rejection.
Many other lines of evidence establish the immunologic nature of graft rejection as follows: (1) histologic examination of the site of the rejection reveals lymphocytic and monocytic cellular infiltration reminiscent of the delayed-type hypersensitivity reaction (see Chapter 17); both CD4+ and CD8+ cells are present at the site (and, as we shall see later, both play a crucial role in graft rejection); (2) animals that lack T lymphocytes (such as athymic mice, or humans with DiGeorge syndrome; see Chapter 18) do not reject allografts; and (3) the process of rejection slows down considerably or does not occur at all in individuals treated with immunosuppressive drugs. Direct evidence for the immunologic basis of graft rejection comes from the observation that in the absence of immunosuppressive therapy, animals transplanted with foreign tissues or cells generate alloreactive T cells and alloantigen-specific antibodies that ultimately destroy the graft.
Categories of Allograft Rejection
Clinically, allograft rejections fall into three major categories: (1) hyperacute rejection, (2) acute rejection, and (3) chronic rejection. The following are descriptions of the rejection reactions as might be observed, for example, after transplantation of a kidney; they also apply for rejection of other tissues.
Hyperacute Rejection
Hyperacute rejection occurs within a few minutes to a few hours of transplantation. It is a result of destruction of the transplant by so-called preformed antibodies to incompatible MHC antigens and, in some cases, to carbohydrates expressed on transplanted tissues (e.g., on endothelial cells). Such antibodies have been produced in the recipient prior to transplant. In some cases, these preformed antibodies are generated as a result of previous transplantations, blood transfusions, or pregnancies. These cytotoxic antibodies activate the complement system, followed by platelet activation and deposition, causing swelling and interstitial hemorrhage in the transplanted tissue, thereby decreasing the flow of blood through the tissue. Thrombosis with endothelial injury and fibrinoid necrosis is often seen in cases of hyperacute rejection. The recipient may have fever, leukocytosis, and will produce little or no urine. The urine may contain various cellular elements, such as erythrocytes. Cell-mediated immunity is not involved at all in hyperacute rejection.
Acute Rejection
Acute rejection is seen in a recipient who has not previously been sensitized to the transplant. There are two types of acute rejection for solid organs. One type is antibody-mediated or humoral acute rejection, and the other is acute cellular rejection (sometimes abbreviated as acute rejection). Humoral acute rejection usually occurs within the first 3 months following transplantation. In the case of kidney transplants, this manifests as a sudden decline in kidney function. Donor-specific antibody (DSA) is detected and C4d is deposited on the endothelium of the kidney due to activation of complement. Enlargement and tenderness of the grafted kidney, a rise in serum creatinine level, a fall in urine output, decreased renal blood flow, and presence of blood cells and proteins in the urine are characteristic. Acute cellular rejection usually occurs 1–6 weeks to several years following transplantation when doses of immunosuppressive drugs are lowered. Histologically, cell-mediated immunity, manifested by intense infiltration of lymphocytes and macrophages, is taking place at the rejection site. The distinction between humoral acute rejection and acute cellular rejection is important because treatments may differ. If the acute rejection is antibody mediated, patients are treated with B-cell-directed drugs such as Rituxan and/or plasmaphoresis. Acute rejection may be reduced by immunosuppressive therapy, for example, with cyclosporine and other drugs, as we shall see later in this chapter. Treatment with corticosteroids is used for both types of acute rejection.
Chronic Rejection
Chronic rejection caused by both antibody and cell-mediated immunity occurs in allograft transplantation months or years after the transplanted tissue has assumed its normal function. Subclinical (insidious) microvascular inflammation, associated with antibody-mediated rejection, which can be with or without complement activation, predicts progression to chronic rejection, transplant dysfunction, and failure. It is noteworthy that humoral responses beyond major histocompatibility antigens continue to receive the attention of the transplantation community and these may contribute to such microvascular inflammatory responses. Clinical relevance of antibodies targeting angiotensin type 1 receptor is broadly confirmed in renal and cardiac transplantation, where, in addition, antibodies against endothelin type A receptor have been found. Finally, antibodies directed against K-α 1 tubulin, and collagen-V have been implicated as non-HLA (human leukocyte antigen) targets of antibodies formed following allografts, and more recently anti-perlecan antibodies have been identified as accelerators of obliterative vascular lesions. Clearly, a better understanding of intersections of HLA-related and non-HLA-related mechanisms, and identification of common effector mechanisms would represent an important step towards targeted therapies.
In cases of kidney transplantation, chronic rejection is characterized by slow, progressive renal failure. Histologically, the chronic reaction is accompanied by proliferative inflammatory lesions of the small arteries, thickening of the glomerular basement membrane, and interstitial fibrosis. Because the damage caused by immune injury has already taken place, immunosuppressive therapy at this point is useless, and little can be done to save the graft.
While the preceding example is for kidney transplantation, it is important to point out that the rate, extent, and underlying mechanisms of rejection may vary, depending on the transplanted tissue and site of the transplanted graft. The recipient’s circulation, lymphatic drainage, expression of MHC antigens on the graft, and several other factors determine the rejection rate. For example, bone marrow and skin grafts are very sensitive to rejection as compared to heart, kidney, and liver grafts.
Role of MHC Molecules in Allograft Rejection
Antigens that evoke an immune response associated with graft rejection are referred to as transplantation antigens, or histocompatibility antigens. Indeed, the major histocompatibility complex was so named because of its central role in graft rejection. Why do these molecules serve as the major antigenic targets for the T cells that are ultimately responsible for graft rejection? There are at least two reasons for this: As discussed in Chapter 9, gene products of the MHC are cell-surface proteins. All nucleated cells express class I MHC molecules whereas class II molecules are normally expressed only on a subset of hematopoietic cells and by thymic stromal cells. Other cell types may also be induced to express class II MHC following their exposure to the proinflammatory cytokine, IFN-γ . In an organ transplantation scenario, when a donor and recipient are MHC disparate (allogeneic), the immune response will be directed predominantly against foreign MHC class I antigens expressed on the cells in the grafted tissue. Furthermore, foreign MHC molecules activate an enormous number of T-cell clones in the recipient. It is estimated that up to 5% of all T-cell clones in the body may be activated in response to alloantigen activation, orders of magnitude higher than the response to other antigens. The combination of nonself MHC molecules plus bound peptides cross-reacts with T-cell receptors (TCRs) expressed on many different T-cell clones. Other mechanisms, discussed below, also contribute to how alloantigens on the transplant are presented to the recipient’s T cells.
Mechanisms of Alloantigen Recognition by T Cells
As we discussed in Chapters 9 and 10, the specificity of T cells is normally restricted by self-MHC, the allelic specificity seen in the thymus during T-cell differentiation. Thus, the exposure of an individual to nonself-MHC molecules expressed on the graft represents an artificial but clinically relevant situation.
There are two mechanisms of alloantigen recognition by T cells: direct and indirect. When T cells are exposed to foreign cells expressing nonself MHC (class I or class II), many clones are “tricked” into activation because their TCRs bind to (ligate) the foreign MHC–peptide complex being presented. This direct mechanism is presumably due to the recognition of foreign MHC bound to donor-derived peptides. It is important to recall that physiologically, MHC molecules can, and do, normally bind self-peptides. Self-proteins are routinely digested within cytosolic organelles called proteosomes, and peptides are delivered to the endoplasmic reticulum where they can bind to MHC class I molecules. Such MHC–self-peptide complexes are believed to stabilize the structure of the MHC molecules and are of no consequence when expressed on the surface of cells in a normal individual because there is tolerance to self-peptides. When foreign peptides are bound to donor MHC molecules expressed by grafted cells, the donor MHC–peptide complex has functional cross-reactivity with the self-MHC–peptide combination and thus activates peptide-specific T cells. Hence, when donor-derived peptides are presented to T cells by antigen presenting cells (APCs) that are “passengers” in the transplanted tissue (donor dendritic cells, in particular), they are recognized as foreign. These passenger APCs become activated due to the “danger signals” they encounter—unavoidable injurious consequences of organ transplantation. The donor APCs therefore become activated as evidenced by their increased expression of MHC class II molecules, increased expression of T-cell co-stimulatory molecules, and use of chemokine receptors to facilitate their trafficking to host secondary organs. In short, these activated APCs mature to become potent stimulators of host T cells.
The indirect mechanism of T-cell alloantigen recognition involves the APCs of the recipient. Host APCs process alloantigenic proteins and present the resulting peptides on self-MHC molecules to T cells expressing TCRs that bind to these foreign epitopes. This is essentially the same process by which epitopes derived from any other foreign protein activate peptide-specific T cells. It is now known that a major source of the donor-derived peptides presented in this direct fashion are the minor H antigens encoded by genes outside the MHC. Responses to minor H antigens are generally mediated by CD8+ T cells because they are presented by class I MHC molecules. As we will discuss later in this chapter, minor H antigens appear to be important in bone marrow transplantation and have been implicated in graft-versus-host (GVH) disease in cases of HLA-matched bone marrow transplantations.
In short, direct activation of T cells by alloantigens is due to recognition of donor-derived MHC antigens expressed by donor cells serving as APCs. Indirect activation of T cells occurs via recognition of donor-derived cellular peptides (mostly minor H antigens) bound to MHC antigens expressed by host APCs (Figure 19.2). The relative contribution of these two mechanisms to graft rejection is not known. The direct mechanism of alloantigen recognition is believed to be of importance in acute rejection of grafts as discussed earlier in this chapter. The destruction of donor cells, in this case, is therefore directly mediated by T cells. By contrast, indirect alloantigen recognition by T cells also involves activation of host macrophages that cause tissue damage and fibrosis. Moreover, such activation leads to the development of cytotoxic alloantibody responses, which may also play a role in the destruction of the graft.
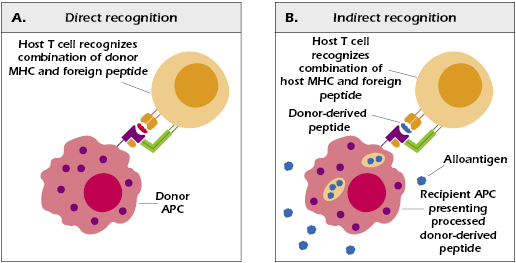
Role of T Cell Lineages and Cytokines in Allograft Rejection
Alloactivation of T cells generates both allospecific CD4+ and CD8+ cells. The cytokines produced are synthesized mainly by activated CD4+ T cell clones. The most important cytokines generated are during these responses are IL-2, IFN-α, IFN-β, and IFN-γ, TNF-α, and TNF-β. IL-2 is important for T-cell proliferation, and for differentiation of cytotoxic T lymphocytes (CTLs) and TH1 cells participating in the delayed-type hypersensitivity reactions associated with allograft rejection. IFN-γ is important for the activation of macrophages, which migrate to the graft area causing tissue damage, and TNF-β is cytotoxic to the cells present in graft. IFN-α, IFN-β, TNF-β, and TNF-α increase the expression of class I molecules, while IFN-γ increases the expression of class II molecules on cells (host and allograft cells), thus increasing the effectiveness of antigen recognition and enhancing graft rejection.
It is now clear that in addition to the roles played by the TH1 cells, other CD4+ T cell lineages also participate in graft rejection (Figure 19.3). As we have discussed in preceding chapters, depending on the microenvironment in peripheral lymphoid organs, helper CD4+ T cells differentiate into different types of effector cells that mediate different types of responses. In the presence of IL-12, TH1 cells dominate and mediate IFN-γ-dependent macrophage activation and delayed-type hypersensitivity; in the presence of IL-4, TH2 cells mediate IL-5-dependent eosinophilic rejection; in the presence of TGF-β, IL-6, and IL-23, TH
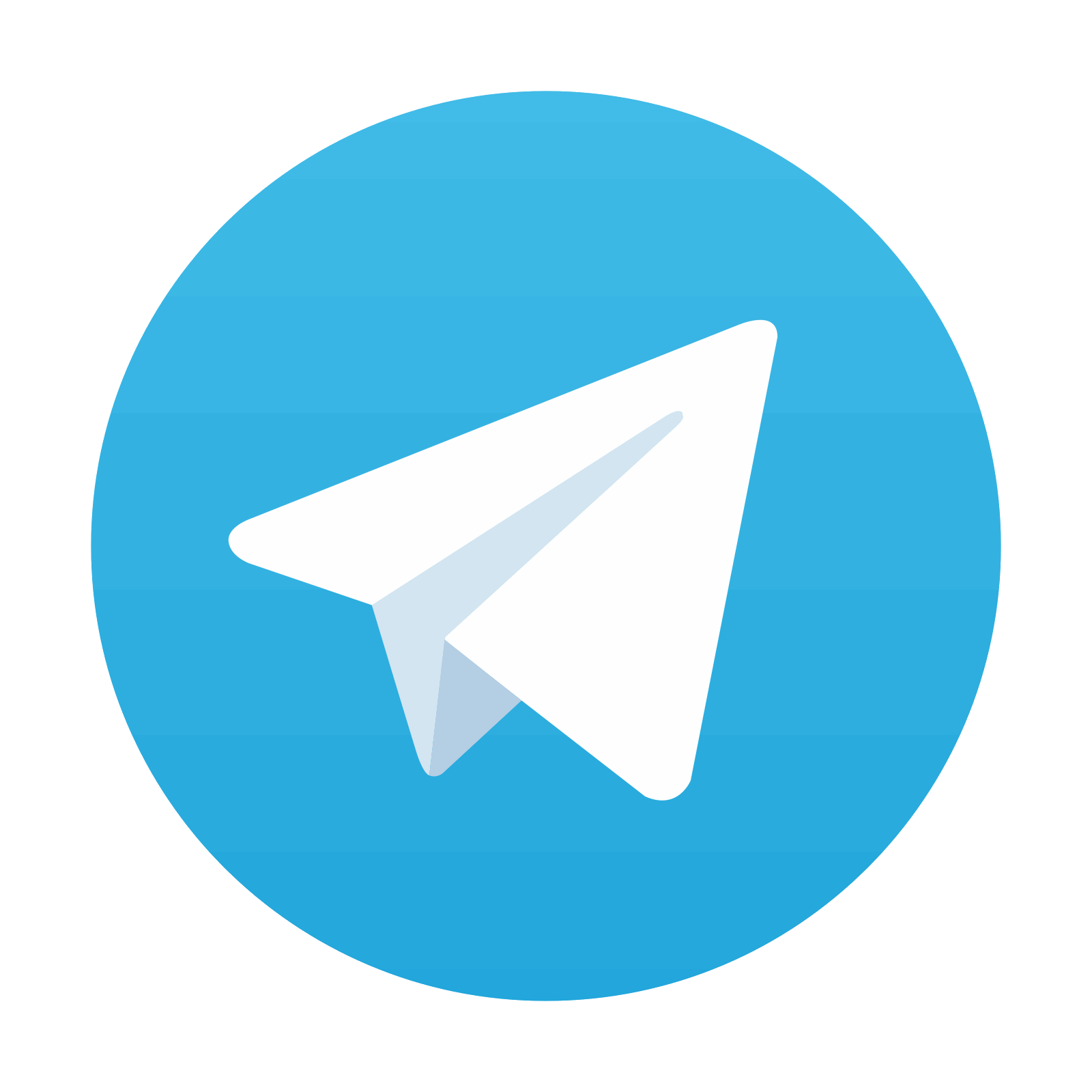
Stay updated, free articles. Join our Telegram channel

Full access? Get Clinical Tree
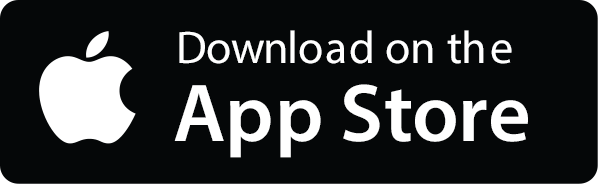
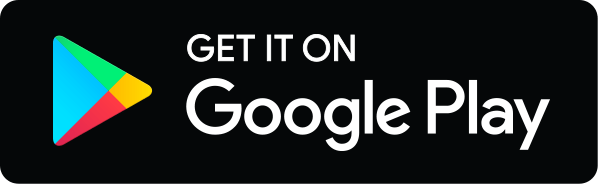