Hypersensitivity: Types II and III
Introduction
Hypersensitivity reactions characterized as type II and type III reactions are mediated by antibodies belonging to the IgG, IgM, and, in some cases, IgA or IgE isotypes. The distinction between these two forms of hypersensitivity lies in the type and location of antigen involved and the way in which antigen is brought together with antibody. Type II hypersensitivity reactions are the result of the binding of antibody directly to an antigen on the surface of a cell. Type III reactions are the result of deposition of antigen–antibody immune complexes. The target antigens involved in type II and type III hypersensitivity reactions are often self-antigens.
Type II Hypersensitivity
Three different antibody-mediated mechanisms are involved in type II hypersensitivity reactions. The targeted cell is either damaged or destroyed through a variety of mechanisms associated with (a) complement-mediated reactions; (b) antibody-dependent cell-mediated cytotoxicity; or, (c) antibody-mediated cellular dysfunction. As illustrated in the examples of clinically important type II hypersensitivity reactions discussed below, many of these reactions are manifestations of antibody-mediated autoimmunity. Mechanisms associated with the generation of autoantibodies were discussed in Chapter 13. The antibodies involved in these hypersensitivity reactions are either directed against normal self-antigens (e.g., cross-reactive antibodies elicited following an infection) or modified self-antigens (e.g., drug-induced autoantibodies elicited following the binding of drugs to certain cell membranes).
Complement-Mediated Reactions
In complement-mediated hypersensitivity reactions, antibodies react with cell membrane self-antigens and this is followed by complement fixation. This activates the complement cascade, as discussed in Chapter 14, and leads to lysis of the cell. Alternatively, binding of antibody to the cell surface and subsequent activation of complement to yield C3b effectively opsonize the target cell (Figure 16.1A). Opsonization culminates in the phagocytosis and destruction of the cell by macrophages and neutrophils expressing surface Fc receptors or receptors that bind C3b. Blood cells are most commonly affected by this mechanism. Interestingly, IgG Fc receptor knockout mice fail to mount type II (and type III) hypersensitivity reactions, a finding that underscores the pivotal role played by IgG Fc receptors in initiating these reaction cascades.
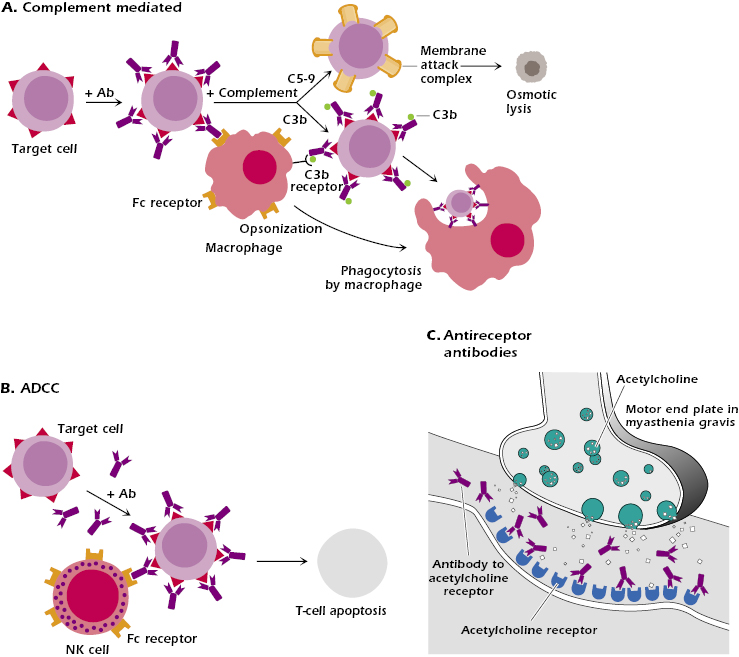
Antibody-Dependent Cell-Mediated Cytotoxicity
Antibody-dependent cell-mediated cytotoxicity (ADCC) utilizes Fc receptors expressed on many cell types (e.g., natural killer [NK] cells, macrophages, neutrophils, eosinophils) as a means of bringing these cells into contact with antibody-coated target cells (Figure 16.1B). Lysis of these target cells requires contact but does not involve phagocytosis or complement fixation. Instead, ADCC-mediated lysis of target cells is analogous to that of cytotoxic T cells and involves the release of cytoplasmic granules (modified lysosomes) containing perforin and granzymes. Once released from the lytic granules, perforins insert into the target cell membrane and polymerize to form pores. In contrast, granzymes, which consist of at lease three serine proteases, enter the cytoplasm of the target cell and activate events leading to apoptosis.
ADCC reactions are typically triggered by IgG binding to IgG-specific Fc receptors (FcγIII, also known as CD16) on the effector cell. However, IgE antibodies can also be involved in ADCC. In this situation, the low-affinity IgE Fc receptor (FcεRII) expressed on certain cells, including eosinophils (see Chapter 15), binds to the Fc portion of IgE antibodies bound to target antigens (e.g., parasites) (see Figure 15.2).
Antibody-Mediated Cellular Dysfunction
In some type II hypersensitivity reactions, antibodies bind to cell-surface receptors that are critical for the functional integrity of the cell. When autoantibodies bind to such receptors they impair or dysregulate cell function without causing cell injury or inflammation.
In the following section, we provide several examples of clinically important antibody-mediated cytotoxic hypersensitivity reactions.
Examples of Type II Hypersensitivity Reactions
Transfusion Reactions
Transfusion of ABO-incompatible blood results in complement-mediated cytotoxic reactions. As an example, individuals with type O blood have in their circulation IgM anti-A and anti-B antibodies (isohemagglutinins), which react with the A and B blood-group substances, respectively (see Chapter 5). If such a person were to be transfused with red blood cells (RBCs) expressing the type A blood group antigen, the consequences could be disastrous. Since there is a considerable amount of IgM anti-A antibody in this person’s circulation, all the transfused type A red blood cells will bind to some antibody. Because of the efficiency of IgM antibody in activating complement (a single IgM molecule is sufficient to activate many complement molecules; see Chapter 14), and because of the absence of repair mechanisms, RBCs will be lysed intravascularly by the destructive action of complement on their membranes. Not only will this nullify the desirable effects of the transfusion, but the individual will also face the risk of kidney damage from blockage by large quantities of RBC membrane, plus the possible toxic effects from the release of the heme complex.
Drug-Induced Reactions
In some people, certain drugs act as haptens and combine with cells or with other circulating blood constituents and induce antibody formation. When antibody combines with cells coated with the drug, cytotoxic damage results. The type of pathologic injury depends on the type of cell that binds the drug. For example, some drugs can bind to platelets causing them to become immunogenic. The antibody responses that are generated cause lysis of the platelets and resulting thrombocytopenia (low blood platelet count). This disorder, in turn, can give rise to purpura (hemorrhage into the skin, mucous membranes, and internal organs), which is the main problem in drug-induced thrombocytopenia purpura. Withdrawal of the offending drug leads to a cessation of symptoms. Other drugs, such as chloramphenicol (an antibiotic), may bind to white blood cells; phenacetin (an analgesic) and chlorpromazine (a tranquilizer) may bind to red blood cells. The consequences of an immune response to these drugs can lead to an agranulocytosis (decrease in granulocytes) in the case of white blood cells, and a hemolytic anemia in the case of red blood cells. Damage to the target cell in these examples may be mediated by either of the two mechanisms described above: by cytolysis via the complement pathway or by destruction of cells by phagocytosis mediated by receptors for Fc or C3b.
It should be pointed out that whereas the preceding discussion emphasizes type II reactions induced by drugs, hypersensitivity to drugs may also induce IgE-mediated immediate type I hypersensitivity reactions (Chapter 15), type IV delayed-type hypersensitivity reactions (discussed in Chapter 17), and the immune complex-mediated reactions (type III) discussed below. As noted earlier, some reactions are induced by a drug acting as a hapten conjugated to a particular self-antigen.
Rhesus Incompatibility Reactions
A somewhat similar mechanism is exemplified by the rhesus (Rh) incompatibility reaction seen in infants born of mothers with Rh-incompatible blood groups. Rh antigens are so named because rabbit antisera raised against rhesus monkey RBCs (RhRBCs) agglutinate the erythrocytes from approximately 85% of humans tested. RBCs from such individuals are therefore said to be Rh+, whereas cells from the remaining 15% of the population are Rh−. Rh− mothers can become sensitized to Rh antigens during their first pregnancy with a child whose RBCs are Rh+. This occurs as a result of the release of some of the baby’s RBCs into the mother’s circulation perinatally and particularly during birth. If the mother is thereby sufficiently immunized to produce anti-Rh antibody of the IgG isotype, subsequent Rh+ fetuses will be at risk, since, as we saw in Chapter 5, IgG antibody is capable of crossing the placenta. Thus, in second or subsequent pregnancies, when the anti-Rh IgG antibodies have crossed the placenta, they bind to the Rh antigen on the RBCs of the fetus. Because the density of Rh antigen on the surface of RBCs is low, these antibodies usually fail to agglutinate or lyse the cells directly. However, the antibody-coated cells are readily destroyed by the opsonic effect of the Fc portions of the IgG, which interact with the receptors for Fc on the phagocytic cells of the reticuloendothelial system. The result is progressive destruction of the fetal or newborn RBCs, with the pathologic consequences that come from decreased transport of oxygen and from increased bilirubin from the products of the breakdown of hemoglobin, a condition known as erythroblastosis fetalis (hemolytic disease of the newborn). Prevention of this Rh incompatibility reaction can be achieved with the administration of anti-Rh antibodies (passive immunization) to the mother at 26–28 weeks’ gestation and again within 72 hours of parturition to effectively block the sensitization phase. This also causes a rapid clearance of Rh+ cells from the mother’s circulation. One widely used preparation of anti-Rh antibodies involves the use of antibodies (Rhogam) against the D antigen, now known to be the strongest immunogen and the most important of all the Rh antigens.
Reactions Involving Cell Membrane Receptors
An example of antibody-mediated cellular dysfunction due to reactivity with a cell receptor is seen in the autoimmune disease myasthenia gravis. Antagonistic autoantibodies reactive with acetylcholine receptors in the motor end plates of skeletal muscles impair neuromuscular transmission, causing muscle weakness (Figure 16.1C). Conversely, in Graves’ disease, the autoantibodies serve as agonists, causing stimulation of the target cells. These antibodies are directed against thyroid-stimulating hormone receptor on thyroid epithelial cells and stimulate the cells, resulting in hyperthyroidism. These disorders are discussed in more detail in Chapter 13, which deals with the subject of autoimmunity.
Reactions Involving Other Cell Membrane Determinants
As a consequence of certain infectious diseases, or for other, still unknown reasons, some individuals produce autoantibodies reactive against their own blood cells. When RBCs are the target, binding of anti-RBC autoantibody shortens their life span or destroys them immediately. Destruction can be complement-mediated resulting in RBC hemolysis or mediated by phagocytosis following the binding of phagocytes to the Fc regions of autoantibodies or C3b bound to these cells. This may lead to progressive anemia if the production of new RBCs cannot keep pace with destruction. Occasionally, the antibody only binds effectively at lower temperatures (so-called cold agglutinins), in which case regions of lower body temperature, particularly in the figures and toes, leads to effective antibody binding and destruction of the RBCs.
Another example of cell destruction by autoantibodies is immune thrombocytopenia purpura (ITP), previously known as idiopathic thrombocytopenia purpura. In this condition, antibodies directed to platelets result in platelet destruction by complement or phagocytic cells with Fc or C3b receptors. Decrease in platelet numbers may lead to bleeding (purpura). Similarly, autoantibodies directed against granulocytes can induce agranulocytosis predisposing individuals to various infections. Finally, antibodies may form against other tissue components such as a type of basement membrane collagen particularly prevalent in the lung and kidneys, causing Goodpasture’s syndrome (see Chapter 13), and desmosomes between keratinocytes in the skin, resulting in pemphigus vulgaris.
Type III Hypersensitivity
Under normal conditions, circulating immune complexes
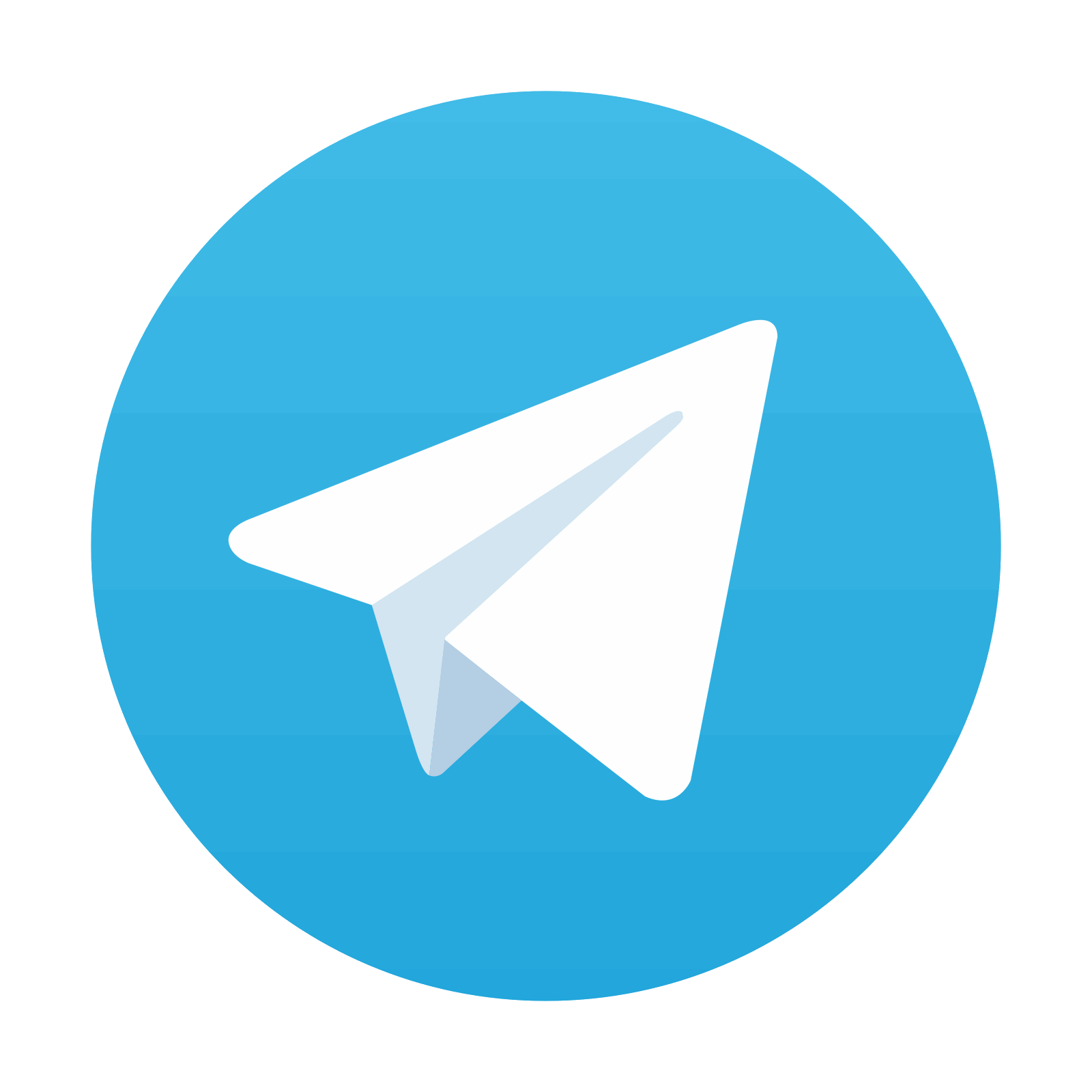
Stay updated, free articles. Join our Telegram channel

Full access? Get Clinical Tree
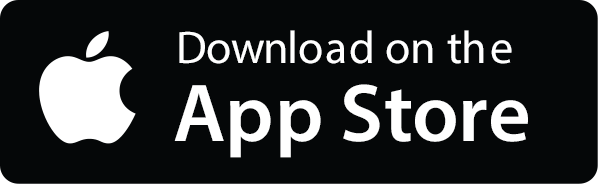
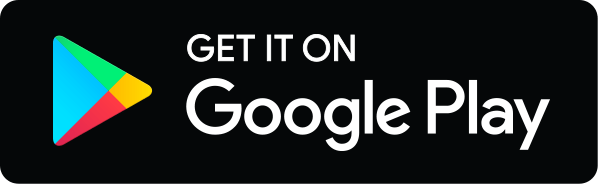