IATROGENIC INFECTIONS OF THE CENTRAL NERVOUS SYSTEM
KELLY J. BALDWIN AND JOSEPH R. ZUNT
Iatrogenic infection of the central nervous system (CNS) can occur as a complication of surgery to the cranium, brain, or, less frequently, dural puncture. There is significant morbidity and mortality associated with postneurosurgical-related CNS infections. Early recognition and treatment is crucial to reduce adverse outcomes and long-term complications. This chapter describes the epidemiology, differential diagnosis, clinical presentation, workup, and treatment of iatrogenic CNS infection. Special considerations regarding neurosurgical devices and surgery-related prion disease are also reviewed.
INFECTION ASSOCIATED WITH NEUROSURGICAL DEVICES
Etiology
Coagulase-negative staphylococci (CoNS), Staphylococcus aureus, gram-negative bacilli, and Propionibacterium acnes are the most common organisms causing infection associated with placement of a neurosurgical device (1,2). Tables 35.1 and 35.2 summarize the risks for CNS infection and etiologic agents associated with ventriculoperitoneal (VP) shunt and external ventricular drains (EVD) (1–33). Diagnosis of device-related infection can be complicated by the ability of some organisms to produce biofilms, often referred to as “slime,” resulting in false-negative cultures. S. aureus and certain CoNS, such as Staphylococcus epidermidis, are able to form biofilm. First recognized in 1972, biofilms are complex communities of bacteria that enter a dormant mode to survive. They induce enhanced adherence of bacteria to shunt material, thus decreasing susceptibility to both diagnosis and antibiotic therapy (34–36). The presence of foreign material, such as a shunt catheter, can also diminish the host immune system’s ability to adhere to and phagocytose bacteria (37).
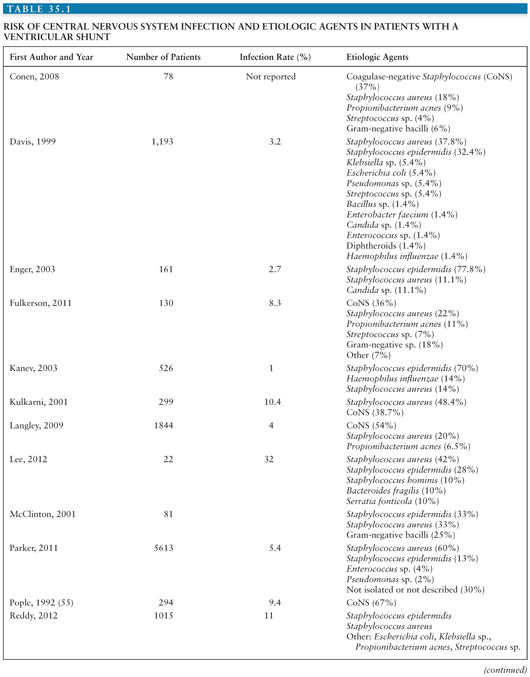
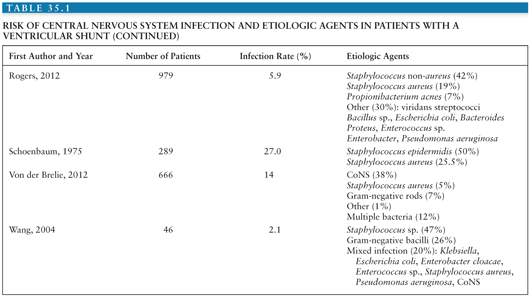
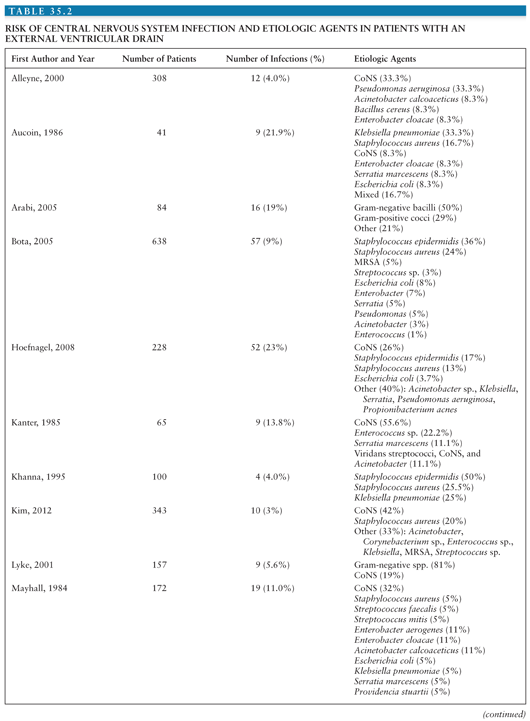
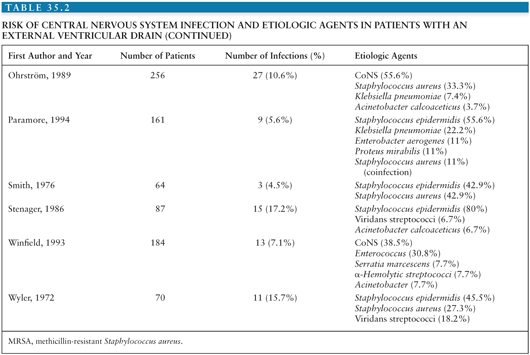
S. aureus is the organism most commonly isolated in shunt infection of children (38). Although gram-positive organisms are the most common cause of early postsurgical infection, gram-negative organisms can produce delayed infection (18). Neisseria gonorrhoeae has been reported in a fetus with a ventriculoamniotic shunt who developed ventriculitis (39).
Fungal meningitis, particularly due to Candida spp., is a rare complication of device-related infections; however, it is associated with significant morbidity and mortality. Candidal infection should be suspected in patients with neurologic deterioration in the setting of device placement, especially if there is an antecedent history of bacterial infection (40). In one study, fungal infection accounted for 17% of shunt infections in premature babies undergoing VP shunt placement for treatment of hydrocephalus; fungi isolated included Candida albicans, Candida parapsilosis, Candida tropicalis, and Torulopsis glabrata (41).
The four most common mechanisms for acquisition of shunt infection include (a) contamination at the time of surgery, (b) direct extension of skin infection at the surgical site, (c) transmission of infection from the distal catheter tip, and (d) hematogenous spread of infection from other sites (42).
Differential Diagnosis
Potential complication of VP shunt placement include mechanical malfunction, occlusion of the distal outflow catheter in the abdomen, intracranial migration, displacement or malfunction of the proximal inflow catheter or valve, and shunt infection. Over 50% of shunts malfunction within 10 to 12 years of placement, and the ratio of shunt revisions to primary shunt placement approaches 3:1 (43–45). As many symptoms associated with shunt infection, such as headache, drowsiness, and vomiting, are similar to those caused by shunt malfunction, blockage, or breakage (46), it is often difficult to distinguish between infection and malfunction, particularly when cerebrospinal fluid (CSF) cultures are negative and CSF parameters are normal. More than half (62%) of shunt infections manifest within the first month of placement, with most patients presenting with fever (78%), nuchal rigidity (45%), or local sign of infection, characterized by erythema, local pain, swelling, or purulent discharge (49%) (2). In addition, over 80% of patients have a CSF leukocytosis with elevated lactate.
Clinical Symptoms and Signs
Symptoms of shunt infection or malfunction typically result from increased intracranial pressure and can include headache, nausea, vomiting, and altered mental status. Symptoms of shunt infection usually develop within the first month, and nearly all occur within 6 months of device placement (47). Regardless of shunt type, over 80% of patients with shunt infection will develop fever. Drowsiness may be the best clinical predictor of shunt malfunction, followed by headache and vomiting (44). Due to containment of most infections within the ventricles, only one third of patients with VP shunt infection develop signs of meningeal irritation, whereas the majority of patients with lumboperitoneal shunt infection develop meningeal irritation, due to direct extension of infection within the CSF space to the meninges (48). Atypical symptoms may include seizure, cranial nerve palsy, hemiparesis, visual deficit, and rigidity (44,49–51). Erythema, pain, swelling, or wound drainage may be present over the valve, cranial incision site, or distal outflow catheter (2). Accumulation of fluid along the shunt tract or at the operative site, although highly correlated with CNS infection, occurs in less than 10% of patients with VP shunt infection (3). Infection at the distal catheter site typically produces an inflammation of surrounding tissues, followed by decreased absorption of CSF, then formation of CSF-filled cysts or loculations (52). Patients with an infected distal catheter in the peritoneal space may develop symptoms of peritonitis, appendicitis, or perforated viscus (53,54).
In children, VP shunt infection is usually preceded by fever, irritability, or shunt malfunction (56). Among children who undergo initial shunt insertion after 2 months of age, absence of irritability, nausea, vomiting, and headache are strong predictors for the absence of shunt failure or infection (57).
Laboratory and Imaging Studies
Neuroimaging should be obtained in all patients in whom shunt infection is suspected. Neuroimaging should be followed by CSF examination to evaluate for suspected shunt infection. CSF can be obtained from the shunt reservoir or via lumbar puncture. Bacterial and fungal culture of CSF, in addition to blood culture, should be obtained from all patients with suspected shunt infection; in the setting of suspected shunt infection, CSF culture is positive in up to 66% of patients (2). Culture of CSF obtained from the shunt is more likely to identify the causative organism than culture of CSF obtained by lumbar or ventricular tap (14,58,59). Administration of antibiotics prior to obtaining CSF culture reduces the likelihood of obtaining a positive culture; one study noted a decline in positive CSF cultures from 97% to 53% (59).
A positive CSF Gram stain and culture is considered the gold standard for diagnosis of shunt infection. CSF analysis in shunt infections typically reveals a leukocyte count of greater than 5 × 106 cells/mm3; however, cell count can be normal in up to 20% of patients with shunt infection (2). Most experts agree that more than 100 white blood cells (WBC)/mm3 is strongly suggestive of infection (60–62). The presence of leukocytosis with a predominance of polymorphonuclear leukocytes (PMN) is typical for shunt infection and should elicit initiation of antimicrobial treatment. In children, eosinophilia (defined as ≥8% eosinophils of total CSF WBC), in the absence of peripheral eosinophilia or parasitic infection, was associated with increased risk of shunt infection and higher number of shunt revisions (63). Other studies have reported eosinophilia without infection (64). A recent study correlated the initial CSF WBC count with organism type. Gram-negative organisms were associated with a higher WBC count, with early peak and higher percentage of PMNs. Propionibacterium acnes produced a lower percentage of PMNs, lower WBC count, and higher percentage of eosinophils. As Propionibacterium spp. grow slowly, CSF culture should be held for at least 14 days before a negative report is considered final. Shunt infection typically follows a pattern of initial PMN predominance followed by a later peak of lymphocytes, monocytes, and eosinophils. With successful treatment, an elevated cell count should normalize (1). CSF lactate levels are higher than 1.9 mmol/L in over 80% of patients with infection (2). Neither shunt infection nor shunt malfunction is significantly associated with increased CSF protein concentration (65).
Neuroimaging
In general, a multimodality approach is used during the radiologic evaluation of suspected shunt infection or malfunction: plain radiography, computed tomography (CT), magnetic resonance imaging (MRI), and CSF flow study each providing valuable information (66). Neuroimaging is most useful when postoperative images are available for comparison. Either CT or MRI can determine if hydrocephalus, enhancement, or fluid loculation is present. An increase in ventricular size is observed in up to 66% to 84% of patients with shunt infection or shunt malfunction. Figure 35.1 demonstrates acute hydrocephalus. An unenhanced CT can provide information regarding ventricular size, shunt location, and the type and integrity of shunt components. When traditional neuroimaging is nondiagnostic, shunt injection or radionuclide scans (shuntograms) can provide an assessment of shunt function. The combination of CT and shuntogram, compared to CT alone, increases the sensitivity for diagnosing shunt malfunction (67). MRI using single-shot fast spin echo (SSFSE) is as sensitive as CT for diagnosing shunt malfunction and does not expose children to radiation. MRI requires reevaluation of the valve setting following the MRI as the magnetic field can affect the valve setting. MRI may also reveal evidence of ventriculitis not seen with CT imaging, such as irregular ventricular enhancement (68). Figure 35.2 demonstrates acute ventriculitis and meningitis in a postneurosurgical patient. At the distal outflow end of the shunt, formation of an abdominal pseudocyst has been reported (69). Distal shunt malfunction can also produce cerebral edema (70). In addition, focal displacement of the dorsal midbrain, visualized by MRI, has been described with shunt infection due to P. acnes (71). Ultrasound has been used as a tool to diagnose neonatal ventriculitis associated with VP shunt; typical findings include increased echogenicity of ventricular fluid in a fine homogeneous pattern or with strandlike material and coarse particles (72).
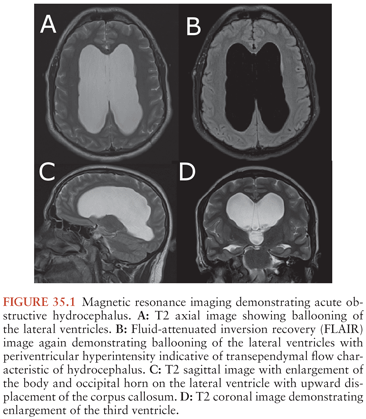
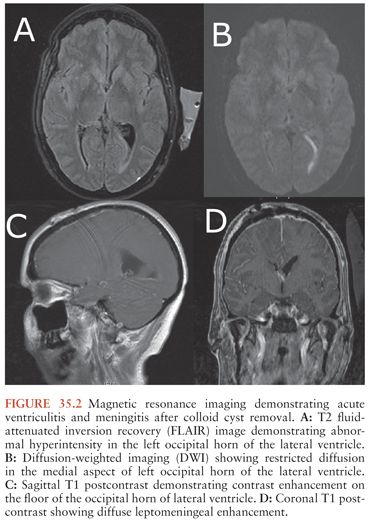
Treatment and Prevention
When shunt infection is suspected, antibiotics should be started after initial CSF and blood cultures are obtained and then adjusted according to antibiotic sensitivity of any identified pathogen(s); in most patients, the etiologic pathogen will be S. epidermidis or S. aureus. A combination of vancomycin with a third-generation cephalosporin will cover the majority of pathogens causing shunt infection. Doses and recommended administration schedules can be found in Table 35.3 (73,74). Side effects of vancomycin include ototoxicity and nephrotoxicity, which can be limited through careful monitoring of serum vancomycin levels. There have been reports of increasing rates of β-lactam antibiotic-resistant CoNS (15).
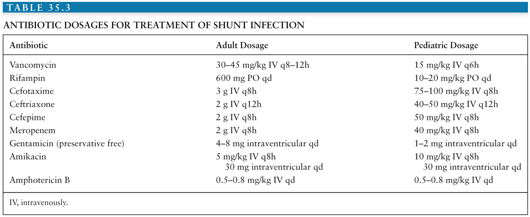
Some experts recommend intrathecal administration of vancomycin in patients with gram-positive organisms or of gentamicin when CSF culture reveals infection with Pseudomonas spp. or enteric coliforms (73,15). Intrathecal amikacin has successfully eradicated shunt infection due to Mycobacterium fortuitum (75). Successful treatment of fungal shunt infection usually requires shunt removal and administration of systemic amphotericin C; intrathecal administration should be considered for patients who do not respond to systemic therapy (41).
Systemic antibiotics, with or without concomitant administration through the infected device, are not as effective as administration of antibiotics plus removal of the infected device. Most experts recommend externalizing the infected shunt or replacing the infected shunt with an EVD, administering antibiotics until CSF is sterilized, and then removing the drain and placing a new shunt (76). Recurrent shunt infection following treatment and replacement of an infected shunt occurs in 17% to 52% of patients (77,78).
In general, the literature supports early removal of infected shunt hardware in combination with antibiotics as the treatment of choice for all but the exceptional patient with a shunt-associated infection.
Prevention
Prevention of shunt infection starts with good sterile technique and a neurosurgeon with extensive experience. A recent meta-analysis of prophylactic systemic antibiotics identified 21 eligible trials and supported the use of periprocedural prophylactic antibiotics for placement of VP and ventriculoatrial shunts. The analysis revealed a lower percentage of shunt infections in patients receiving antibiotics prior to and up to 24 hours after surgery (79). These results were not confirmed by two randomized placebo-controlled trials of EVD placement and systemic prophylactic antibiotics (80).
Two new catheter types that have reduced CNS infection include antibiotic and silver-impregnated devices. In vitro studies suggest catheters impregnated with clindamycin and rifampin reduce catheter colonization without inducing antibiotic resistance (81). In a rodent model, rifampin-impregnated catheters prevented colonization by S. epidermidis and S. aureus, whereas nonimpregnated catheters developed colonization with either organism (82). Antibiotic-impregnated shunts have also shown promising results in reducing infection rates. In a recent systematic review of adults and children, antibiotic-impregnated shunts were significantly associated with a lower incidence of shunt infection (3.3% vs. 7.2%) (10). A recent prospective randomized controlled trial of EVD catheters impregnated with clindamycin and rifampin compared to standard catheters did not reveal reduction in EVD infections, but infections were uncommon in both patient groups (11). A prior prospective randomized study reported that positive CSF cultures were seven times less common in patients receiving antibiotic-impregnated EVDs (83). The discrepancy between these two studies may be due to the very low baseline infection rate of the most recent trial (2.8% in the standard cohort) resulting in a failure to demonstrate a benefit of the antibiotic-impregnated EVD. In the Zabramski et al. (83) study, 80% of patients underwent EVD placement in the critical care unit, resulting in higher initial infection rates in the standard catheter group. Similarly, a single institution study found infection rates decreased from 15% to 5% with the use of antibiotic-impregnated catheters (84). Development of antimicrobial resistance has thus far not been increasingly reported in patients receiving these coated catheters. Several studies evaluating the use of silver-impregnated devices, including the SILVER (Silver-Impregnated Line Versus EVD Randomized trial), demonstrated a significant reduction in CNS infections (21% in patients with standard EVD vs. 12.3% in patients with silver-impregnated EVD) and provided class I evidence that silver-impregnated catheters reduced CNS infection. Silver catheters may thus be an alternative to antibiotic-impregnated catheters, as silver is both safe and effective for preventing CNS infection (85).
Specific Device Considerations
Ventriculoperitoneal Shunt
Ventricular shunts, first introduced in 1952, are inserted to relieve increased intracranial pressure associated with hydrocephalus; shunts may also be used to deliver medication or intermittently monitor and reduce intracranial pressure (86). Ventricular shunts consist of a proximal ventricular catheter, an optional access reservoir, a valve to regulate flow, and a distal outflow catheter draining into the peritoneum, atrium, or pleural space. The most commonly used shunt is the VP shunt. The diagnosis of shunt infection can be based on the laboratory and clinical criteria presented in Tables 35.4 and 35.5 (27,87).
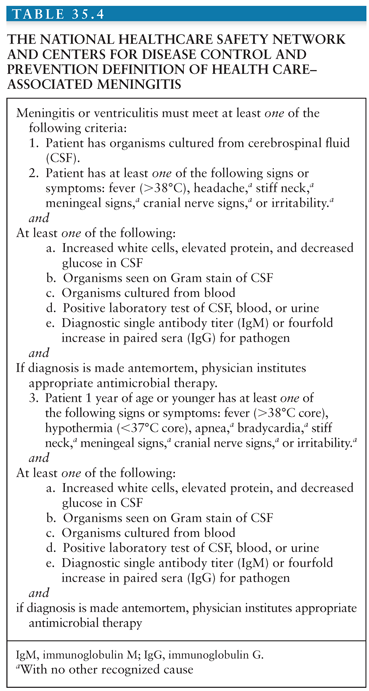
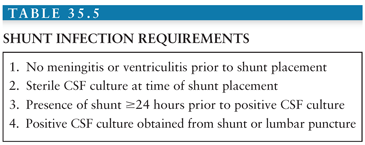
The incidence of shunt infection reported in the literature varies widely from 1% to 39% (88). Children may be at higher risk for developing shunt infection due to longer hospitalization, relative immunodeficiency, and more prominent skin colonization of adherent bacteria (11,34). Factors associated with increased risk of shunt infection include postoperative CSF leak, prematurity, nonsterile technique, use of a three-piece shunt system, implantation by a less-experienced neurosurgeon, placement of a shunt revision, and previous shunt infection (6,45,89).
External Ventricular Drain
EVD catheters have been used since the 1960s for the management of acutely elevated ICP. EVDs are often an essential component in the initial management of subarachnoid hemorrhage, intraventricular hemorrhage, head trauma, and obstructive hydrocephalus. Ventriculitis and bacterial meningitis following EVD placement occur with an average incidence of 9% (90). Risk factors for EVD infection include prior history of shunt infection, craniotomy, systemic infection, CNS trauma with cranial fracture, intraventricular hemorrhage, high frequency of device access for sampling CSF, catheter leakage, and prolonged duration of implantation (22,27,91,92). A recent study reported no difference in the incidence of ventriculitis based on age, gender, or mean EVD duration; there was a significant decrease in the incidence of ventriculitis from 17% to 11% with decreased sampling from daily to once every 3 days (93). Another recent study reported a significant decrease in the development of bacterial ventriculitis associated with shorter duration of EVD placement (20 days vs. 12 days), suggesting that earlier removal is preferential (25).
Lumbar Drain
Lumbar drains are most often placed to treat communicating hydrocephalus following subarachnoid hemorrhage or CSF leak or as a diagnostic procedure for normal pressure hydrocephalus. Hydrocephalus complicates the clinical recovery of 20% to 31% of patients after subarachnoid hemorrhage, 40% of whom will improve within 1 day without intervention (94,95). The incidence of meningitis following lumbar drain placement varies from 0% to 25.6% and is highest in patients with subarachnoid or intraventricular hemorrhage (96–98). Meningitis following lumbar drain placement typically occurs within 24 hours of drain placement. Although CSF WBC count of 11 cells/mm3 or more was not a risk for development of meningitis, the majority of patients who developed meningitis typically had an increasing CSF WBC count in the days preceding diagnosis (96).
Intracranial Pressure Monitors
An intracranial pressure (ICP) monitor provides a quantitative measure of ICP and cerebral perfusion pressure when physical examination alone may not reliably predict neurologic deterioration, such as following open or closed head injury or neurosurgery. An ICP monitor may be placed within the subdural space, parenchyma, or ventricle. Complications associated with ICP monitor placement include hemorrhage and infection. CNS infection occurs in 2.9% to 10.3% of patients with ICP monitors. Risk factors for infection include placement in patients with open head injury or hemorrhage, intraventricular monitor placement, presence of CSF leak, concurrent infection outside the CNS, and presence of monitoring device for longer than 5 days (99–101). The use of prophylactic antibiotics for ICP monitor placement or use is controversial. Although initial studies suggested prophylactic antibiotics did not reduce the incidence of CNS infection, other studies have demonstrated a benefit (18,99). A prospective review of prophylactic nafcillin reported that 8% of patients with ventriculostomy systems and 0% of patients with ICP monitors developed CNS infection. However, the majority of infections that occurred were due to gram-positive organisms, many of which were nafcillin resistant. Patients with infection were more likely to be younger and had longer duration of ventriculostomy placement. No specific neurologic diagnosis, presence of intraventricular hemorrhage, or Glasgow Coma Scale (GCS) at time of admission was predictive of development of CNS infection (102). Unlike increased rates of CNS infection complicating shunts placed by less-experienced surgeons, CNS infection rates following insertion of subdural or parenchymal ICP monitor are similar, regardless of whether a neurosurgeon, general surgeon, or midlevel practitioner performs the procedure (103,104).
Deep Brain Stimulators
Deep brain stimulation is an effective treatment for a variety of movement disorders, including Parkinson disease, dystonia, and essential tremor. The determination of infectious complication rates are difficult due to low patient numbers, limited reporting, and lack of standardized protocols. The prevalence of infectious complications varies from 0% to 15% (105–108
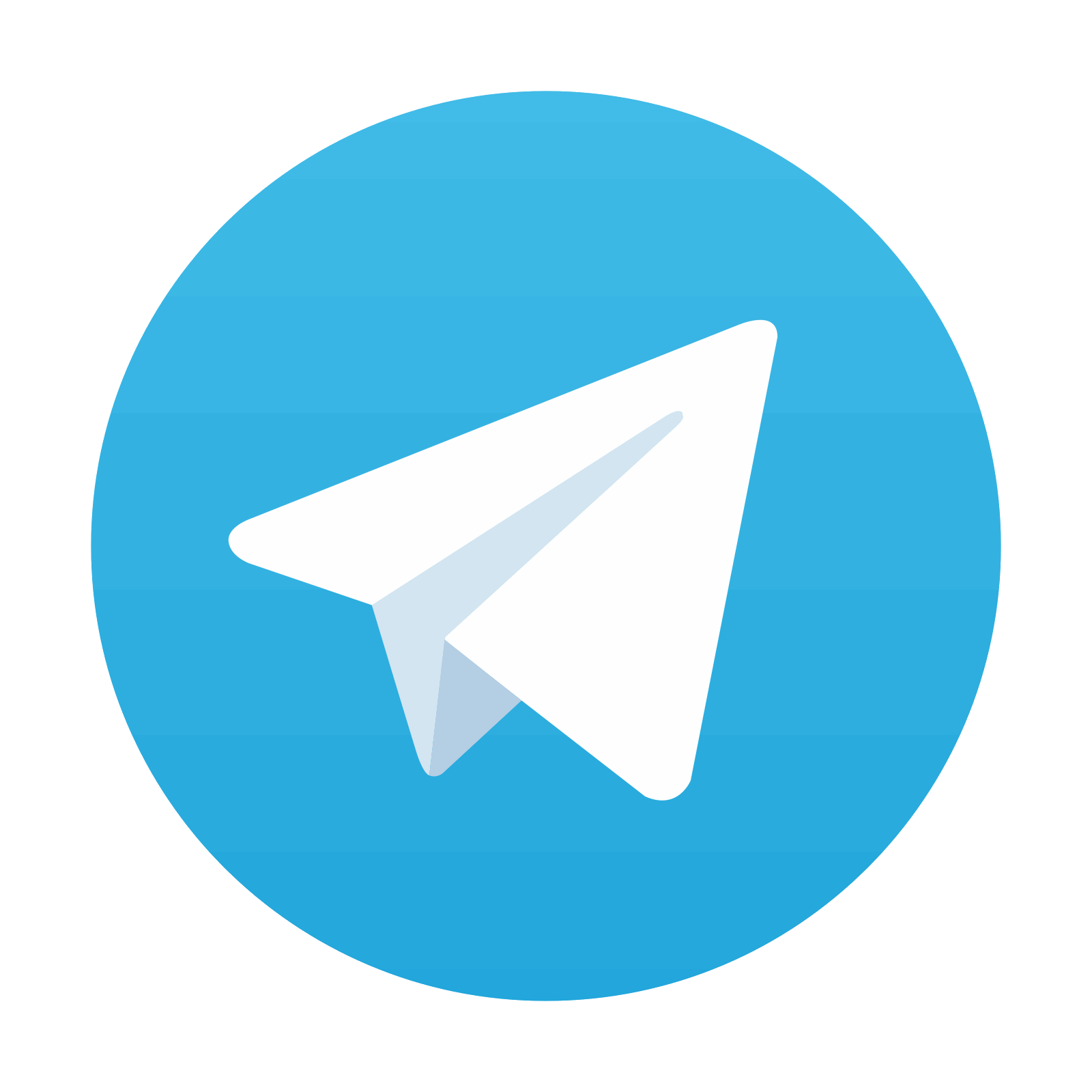
Stay updated, free articles. Join our Telegram channel

Full access? Get Clinical Tree
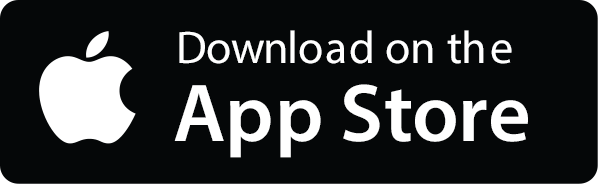
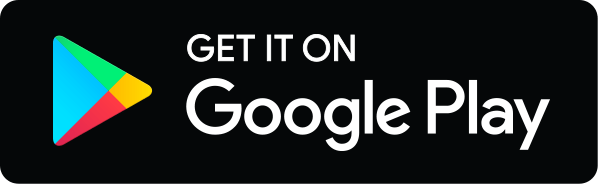