INTRODUCTION
SUMMARY
Hemolytic anemia is a prominent part of the clinical presentation of patients infected with organisms such as the Plasmodium sp., Babesia, and Bartonella, which directly invade the erythrocyte. Malaria is the most common cause of hemolytic anemia on a worldwide basis, and much has been learned about how the parasite enters the erythrocyte and the mechanism of anemia. Falciparum malaria, in particular, can cause severe and sometimes fatal hemolysis (blackwater fever). Other organisms cause hemolytic anemia by producing a hemolysin (e.g., Clostridium perfringens), by stimulating an immune response (e.g., Mycoplasma pneumoniae), by enhancing macrophage recognition and hemophagocytosis, or by as yet unknown mechanisms. The many different infections that have been associated with hemolytic anemia are tabulated and references to the original studies provided.
Acronyms and Abbreviations:
CR1, complement receptor 1; EBA, erythrocyte-binding antigen; G6PD, glucose-6-phosphate dehydrogenase; ICAM, intercellular adhesion molecule; PfEMP, Plasmodium falciparum erythrocyte membrane protein; RSP-2, ring surface protein 2; VCAM, vascular cell adhesion molecule.
Shortening of erythrocyte life span occurs commonly in the course of inflammatory and infectious diseases. This effect may occur particularly in patients with glucose-6-phosphate dehydrogenase (G6PD) deficiency (Chap. 47), splenomegaly (Chap. 56), and in the microvascular fragmentation syndrome (Chaps. 51 and 132). In some infections, however, rapid destruction of erythrocytes represents a prominent part of the overall clinical picture (Table 53–1).1–49 This chapter deals only with the latter states.
Aspergillus1 |
Bacillus anthracis2 |
Babesia microti and Babesia divergens3 |
Bartonella bacilliformis4,5 |
Campylobacter jejuni6,7 |
Clostridium perfringens (Welchii)8,9 |
Coxsackievirus10 |
Cytomegalovirus11 |
Diplococcus pneumoniae12 |
Epstein-Barr virus13,14 |
Escherichia coli15,16,123 |
Fusobacterium necrophorum17 |
Haemophilus influenzae12,23 |
Hepatitis A18,19,20 |
Hepatitis B19,21 |
Hepatitis C22 |
Herpes simplex virus10 |
Human immunodeficiency virus24,25,26 (Chap. 81) |
Influenza A virus27,28 |
Leishmania donovani30 |
Leptospira interrogans serovar ballum and/or Leptospira kirschneri serovar butembo29 |
Mumps virus31 |
Mycobacterium tuberculosis12,32 |
Mycoplasma pneumoniae33 |
Neisseria intracellularis12 |
Parvovirus B1934 |
Plasmodium falciparum35 |
Plasmodium malariae35 |
Plasmodium vivax36 |
Rubella virus37,38 |
Rubeola virus10 |
Salmonella12,39 |
Shigella40,41,123 |
Streptococcus12,42,43,44,45 |
Toxoplasma12 |
Trypanosoma brucei46 |
Varicella virus10,47 |
Vibrio cholerae12 |
Yersinia enterocolitica48 |
Several distinct mechanisms may lead to hemolysis during infections.49 These include direct invasion of or injury to the erythrocytes by the infecting organism, as in malaria, babesiosis, and bartonellosis; elaboration of hemolytic toxins, as by Clostridium perfringens; and development of antibodies, either autoantibodies against red cell antigens or deposition of microbial antigens or immune complexes on erythrocytes, which result in hemolytic anemia.50
MALARIA
Known since antiquity, malaria is the world’s most common cause of hemolytic anemia.36 Human malaria is caused by one of five species of a protozoan, Plasmodium. In 2012, an estimated 207 million episodes of malaria occurred worldwide, resulting in approximately 627,000 deaths, mainly children in sub-Saharan Africa.51 Severe malaria anemia is most commonly seen in young children and pregnant women.52
Malaria transmission depends on geography, rainfall patterns, and the breeding sites of the Anopheles mosquito, the specific vector. Some regions have conditions that make malaria common throughout the year, so-called endemic areas, whereas in other places there are seasonal peaks, usually the rainy season when mosquito breeding is enhanced. Persons in Africa, Asia, the Middle East, and parts of Europe may be at risk. Travelers to such places are at high risk because of lack of immunity and because when they return home, the diagnosis might not be considered promptly. Malaria may also be transmitted by blood transfusion or organ donation from an infected donor.
Sporozoites enter the circulation while the female Anopheles mosquito takes a blood meal. They invade and multiply in hepatocytes. The latter cells rupture when engorged and release merozoites that invade the red cell. In the red cell, the merozoites also cycle through these stages: trophozoites (ring-forms), which then can convert to schizonts. Mature schizonts burst the red cells and release merozoites that invade other red cells. The bursting and release coincides with the abrupt rises in temperature and related signs and symptoms seen in malaria. A small fraction of merozoites in red cells convert to male and female gametocytes that are ingested when the mosquito bites. In the mosquito, they fuse and form an oocyst that divides asexually into numerous sporozoites. The sporozoites migrate to the mosquito’s salivary glands from where they reenter a victim’s blood upon the next bite, initiating a malarial infection. Plasmodium vivax and Plasmodium ovale can persist in the liver in a dormant stage (hypnozoites) and produce relapses months or years later.
After the host is bitten by an infected female Anopheles mosquito, the sporozoites invade the liver and possibly other internal organs in the asymptomatic tissue stage of malaria. Merozoites, emerging at first from the tissues and later from previously parasitized red cells, use specialized invasion proteins such as the erythrocyte binding-like (EBA) and reticulocyte homology (RH) protein families, which bind to receptors on the erythrocyte surface, including glycophorins A/B/C, CR1 (CD35), and basigin (CD147).53,54,55 A complex series of events eventuates in invasion of the interior of the red cell by the parasite.35,53 Having entered the erythrocyte, the parasite grows intracellularly, nourished by the cell’s contents, and modifies the host cell by exporting hundreds of proteins into the cytoplasm, some of which are inserted into the red cell membrane.56
Erythrocytes infected with Plasmodium falciparum develop surface knobs57 that contain receptors, especially the P. falciparum erythrocyte membrane protein-1 (PfEMP-1), for endothelial proteins. All parasites bind to CD36 antigen (platelet glycoprotein IV) and thrombospondin found on endothelial surfaces, whereas some bind to the intercellular adhesion molecule-1 (ICAM-1), and a few bind to the vascular cell adhesion molecule (VCAM)58,59,60,61,62 and mediate the adherence of parasitized cells to endothelium. Activated endothelium secretes strands of ultralarge von Willebrand factor, which bind platelets, allowing PfEMP-1 to interact with platelet CD36 and this provides an additional means of cytoadherence.63 Rosetting of parasitized cells with unparasitized cells also occurs through a mechanism mediated by complement receptor-1 (CR1) on uninfected erythrocytes.64 One of the membrane proteins of P. falciparum binds specifically to the spectrin on the inner surface of the red cell membrane.65 The anemia of falciparum malaria is characteristically normocytic-normochromic anemia with a paucity of reticulocytes (see “Pathogenesis of the Anemia” below). If microcytosis is present, the concomitant presence of α– or β-thalassemia or iron deficiency should be considered.66 A large number of genetic polymorphisms that interfere with invasion of erythrocytes by parasites and their proliferation have developed in areas where malaria has been a leading cause of death for many generations.64,67,68,69 These include G6PD deficiency, Southeast Asian ovalocytosis, CR1 deficiency, the thalassemias, sickle cell anemia (Chaps. 46 to 48), and other hemoglobinopathies.
There are five plasmodial species that cause human malaria: P. falciparum, P. vivax, Plasmodium malariae, P. ovale and Plasmodium knowlesi. The first two cause the most cases worldwide and are principally associated with hemolytic anemia. P. vivax invades only young red cells, whereas P. falciparum attacks both young and old cells. Thus, anemia tends to be more severe in the latter form of malaria and is the most deadly type.35
Destruction of parasitized red cells appears to occur largely in the spleen, and splenomegaly typically is present in chronic malarial infection. The “pitting” of parasites from infected erythrocytes may also occur in the spleen.70 The degree of parasitemia, in part, determines the destruction of infected erythrocytes. Low rates of red cell parasitemia may have little effect on the development of anemia, whereas high rates, for example, 10 percent, may have very significant effects.71 The degree to which anemia develops often seems to be disproportionate to the number of cells infected with the parasite. It is estimated that 10 times the number of uninfected red cells are removed for each infected red cell, dramatically magnifying the hemolytic rate. Osmotic fragility is increased in nonparasitized cells, as well as in cells containing plasmodia.72 The erythrocyte cation permeability is altered in monkeys with malaria.73 Hemin accumulation facilitates hemolytic cell loss via a process of programmed cell death, referred to as eryptosis. This suicidal death pathway is mediated by increased cell calcium, increased annexin-V binding, and ceramide formation.74 Oxidative damage to red cell lipids occurs75,76 and there is an abnormality in the phosphorylation of membranes of parasitized red cells.77 P. falciparum-infected red cells have a highly irregular surface produced by the intracellular growth of the plasmodium, but nonparasitized cells often have similar surface defects.78 Activation of hepatosplenic macrophages enhance red cell clearance supported by red cell surface changes in both parasitized and unparasitized cells that foster recognition and erythrophagocytosis by macrophages. Both marked loss of red cell deformability and deposition of immunoglobulin G and complement (C3d), sometimes resulting in a positive direct antiglobulin reaction, may enhance red cell removal by macrophages.79,80 Parasite products are part of the immune complexes on the red cell surface. The P. falciparum ring surface protein 2 (RSP-2) mediates adhesion of infected red cells to endothelial cells and is deposited on uninfected cells, undoubtedly providing a mechanism for removal of these cells by mediating complement-dependent phagocytosis.66 Splenomegaly further enhances red cell removal from the circulation.
P. falciparum also decreases the erythropoietin response, resulting in less erythropoiesis than expected for the degree of anemia, reticulocytopenia, and coincidentally striking dyserythropoiesis with stippling, cytoplasmic vacuolization, nuclear fragmentation and multinuclearity.66 The inhibition of the erythroid response (anemia of chronic disease) is secondary to release of the cytokines interferon-γ and tumor necrosis factor-α, and the interleukin-10–to–tumor-necrosis-factor ratio, which when low correlates with severe malarial anemia in children (Chap. 37).66
The fever associated with malaria, accompanied by rigors, headache, abdominal pain, nausea and vomiting, and extreme fatigue, is characteristically cyclic, varying in frequency according to the malaria type. Although classic periodicity is often absent, febrile paroxysms of P. vivax malaria tend to occur every 48 hours; those of P. malariae infection occur each 72 hours; and those of P. falciparum malaria, daily. In the latter cases, the periodicity is the result the synchronization of schizogony with schizont rupture occurring at regular intervals. It is schizont rupture that accounts for the fever and associated symptoms. Falciparum malaria is occasionally associated with particularly severe hemolysis, hemoglobinemia, hemoglobinuria, and the passage of dark, almost black, urine. This disorder, also called blackwater fever, is no longer common. At one time it was seen frequently among Europeans in Africa and in India, usually after quinine was given to treat malaria. The event seems to be related to the intermittent use of antimalarials.81
Diagnosis of malaria depends upon demonstration of the parasites on the blood film, or the presence of antigenic parasite proteins using rapid detection tests (RDTs).82 Alternative techniques involve polymerase chain reaction (PCR) to demonstrate the appropriate DNA sequences in the blood83,84 or the use of automated hematology analyzers to identify parasites as part of a routine complete blood count (CBC) investigation.85 The morphologic differentiation of P. falciparum from other forms of malaria, principally P. vivax, is clinically important as P. falciparum infection may constitute a clinical emergency. If more than 5 percent of the red cells infected contain parasites, the infection is almost certainly with P. falciparum. In an infection with this organism, rings are practically the only form of parasite evident on the blood film. The finding of two or more rings within the same red cells is regarded as pathognomonic of P. falciparum (Fig. 53–1A and B). In nonimmune patients, examination of the blood film for malarial parasites should be made for at least 3 days after onset of symptoms because parasitemia may not reach detectable levels for several days.
Figure 53–1.
A. Blood film from a patient with malaria caused by Plasmodium falciparum. Several red cells contain ring forms. Note red cell with double ring form in center of the field, characteristic of P. falciparum infection. Note the ring form with double dots at the left edge of figure, suggestive of P. falciparum infection. Note also high rate of parasitemia (~10 percent of red cells in this field) characteristic of P. falciparum infection. B. Blood film from a patient with malaria caused by Plasmodium vivax. Note mature schizont. C. Blood film from a patient with Babesia microti infection. The heavy parasitemia is characteristic of babesiosis (approximately two-thirds of red cells infected). D. Blood film from a patient with Clostridium perfringens septicemia. Few red cells evident as a result of intense erythrolysis. Neutrophil with two bacilli (C. perfringens). (Reproduced with permission from Lichtman’s Atlas of Hematology, www.accessmedicine.com.)
Early treatment is important. The spread of antimalarial therapy has resulted in major problems with drug resistance. Eradication of blood forms can be achieved with individual agents or combinations of antimalarials. Artemisinins are the most effective agents for P. falciparum. Numerous studies are in progress to determine the best single agent or combination of agents to be used in the treatment of malaria in different regions.86
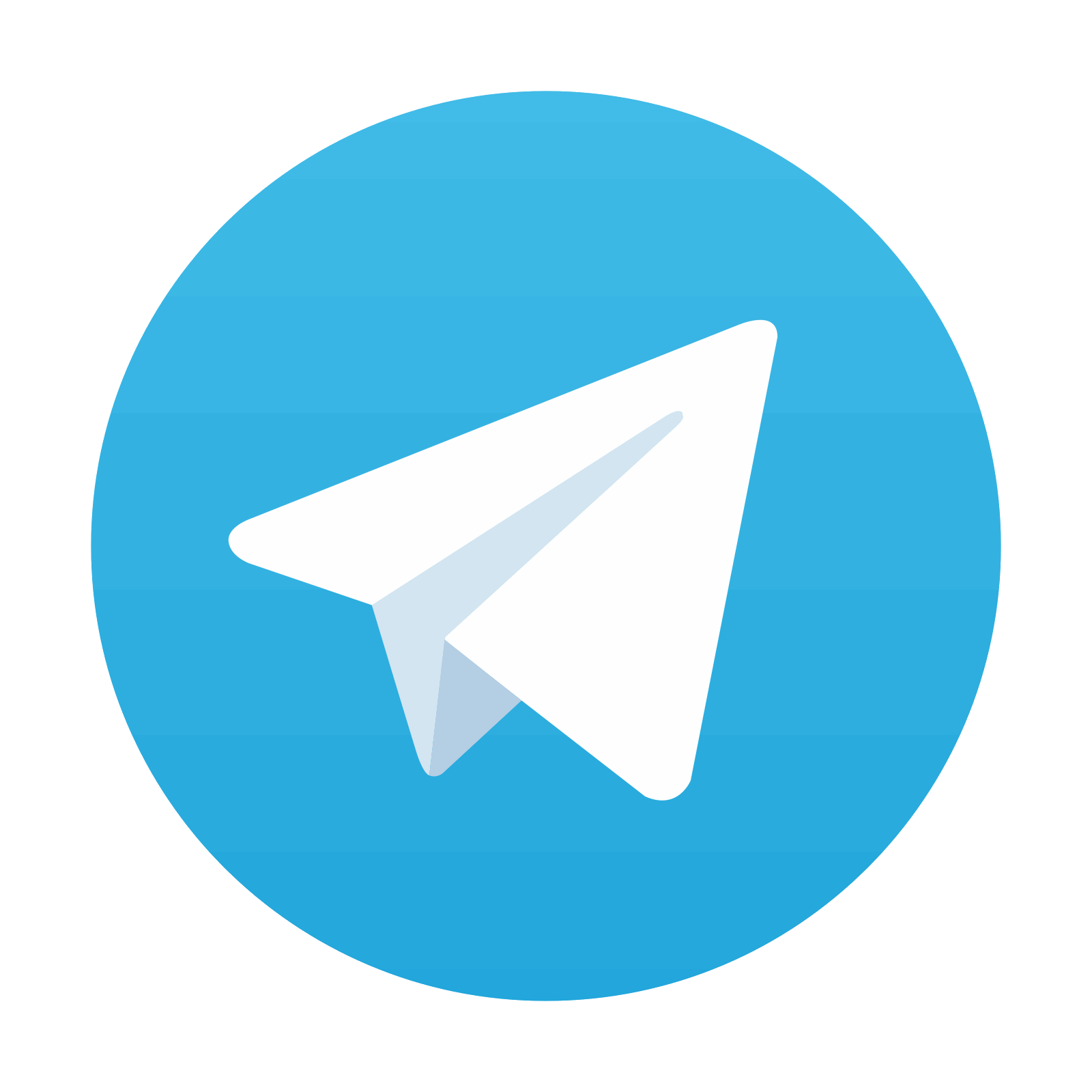
Stay updated, free articles. Join our Telegram channel

Full access? Get Clinical Tree
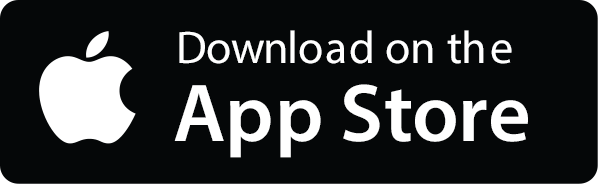
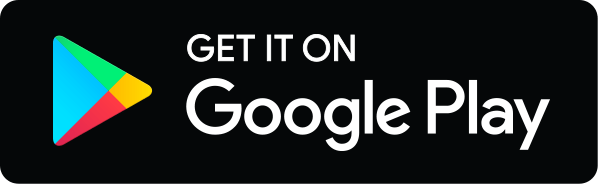
