20 Genetic Profiling in Colorectal Cancer
Introduction
The project of creating genetic profiles involves the molecular characterization of large groups of individuals and/or tissue samples and then discerning patterns in the information gathered. Analysis of the data has been enormously advanced by recruiting statistics, computing, and bioinformatics methods to the enterprise of pattern recognition and validation of the profiles created. In this chapter, we discuss the genetic profiling of colorectal cancer with a specific focus on high-throughput technologies, their potential and current applications, and the future of genetic profiling in our understanding and management of colorectal cancer. This chapter presupposes some familiarity on the reader’s part with the basic models of the development of colorectal cancer, but some of the classic methods of genetic analysis are discussed as they relate to the basic assumptions upon which newer methods of analysis rely.
Types of Genetic Profiling
Genetic Analysis
Although most colorectal cancer is understood as sporadic, up to 5% is thought to result from inherited genetic lesions (see Chapter 3), and another 10% to 15% of colorectal cancers may have a familial predisposition.1 The identification of such familial syndromes of colorectal cancer as Lynch syndrome, Gardner syndrome, Peutz-Jeghers syndrome, and juvenile polyposis syndrome has contributed to our understanding of disease. Familial adenomatous polyposis (FAP) and hereditary nonpolyposis colorectal carcinoma (HNPCC) were identified initially from pedigree analysis, as are many heritable diseases2,3 (see Chapter 3 for further details). Conventional techniques such as linkage analysis were ultimately combined with molecular techniques to identify specific genes involved in the transmission of these diseases, but a thorough understanding of family history remains the mainstay of screening patients for familial cancer syndromes. Thus, the Amsterdam criteria remain an important means of identifying HNPCC patients and their families (Table 20-1), who have an underlying genetic defect in the mismatch repair genes and also display microsatellite instability. The Amsterdam criteria, as revised, include non-colorectal tumors as well, reflecting a broader understanding of HNPCC, whereas the Bethesda criteria identify patients with HNPCC who should undergo further molecular characterization to look for microsatellite instability.4,5
Table 20-1 Amsterdam and Bethesda Criteria for Hereditary Nonpolyposis Colorectal Cancer
Amsterdam I | |
Amsterdam II | Expanded inclusion criteria to tumors of endometrium, stomach, ovary, ureter/renal pelvis, brain, small bowel, hepatobiliary, and skin (sebaceous) |
Bethesda |
CRC, colorectal cancer, FAP, familial adenomatous polyposis; HNPCC, hereditary nonpolyposis colon cancer.
Cytogenetic Analysis
Cytogenetics refers to “gross” chromosomal analysis, in which lesions such as large deletions are detected and initial genetic mapping is carried out. Techniques in chromosomal analysis include G-band analysis, fluorescence in situ hybridization (FISH), and comparative genomic hybridization (CGH) among others. Indeed, the initial involvement of the p53 and DCC genes was discovered on the basis of chromosomal studies on the 17p and 18q loci, respectively. These investigations subsequently led to the recognition of microsatellite instability as a marker of chromosomal aberrations that incur loss of tumor suppressor gene activity.6–8 Table 20-2 lists some chromosomal alterations associated with the development and progression of colorectal cancer that were identified in early studies of the progression of colorectal tumorigenesis. The initial adenoma-carcinoma progression model of colorectal cancer has now evolved further into models that recognize differences in the sequence and character of genetic lesions apparent in different subsets of colorectal cancer. Most important, cytogenetic analysis has clearly identified two pathways in which genetic instability occurs: (1) the microsatellite instability (MIN) pathway and (2) the chromosomal instability (CIN) pathway. Each pathway has a specific and different complexion of genetic lesions with chromosomal instability pathway tumors carrying multiple gross chromosomal lesions versus an accumulation of more subtle mutations in microsatellite instability pathway cancers.9
Table 20-2 Classic Chromosome Alterations Associated with Colorectal Cancer
Chromosome | Alteration | Role in Carcinogenesis |
---|---|---|
5q21 | APC gene mutation/loss | Early event in adenoma-carcinoma sequence; induces degradation of β-catenin |
12p | KRAS mutation | Middle event in adenoma-carcinoma sequence; growth promotion through MAP kinases |
17p | p53 gene deletion | Late event in adenoma-carcinoma sequence; G1 cell cycle arrest, induction of apoptosis |
18q | DCC gene deletion | Late event in adenoma-carcinoma sequence; transmembrane protein that interacts with netrin |
31q | β-catenin mutation/loss | Possible alternate germline mutation in familial adenomatous polyposis, target of APC activity, growth promoter when active form accumulates |
Classical Molecular Analysis
Concurrent with genetic and cytogenetic analysis, numerous investigations into the molecular biology of colorectal cancer have enriched our understanding of the disease process, but initially at the individual gene level in what can now be described as the “classical” molecular biology era. Newtonian in approach, the project of identifying genes that play a role in disease revolved mainly around the identification of mutations in individual genes that then had to (1) be associated with a disease state with some degree of specificity, (2) satisfy the rules of causality (e.g., Koch’s postulates), and (3) be reliably attributed with some pathogenetic feature in terms of diagnosis, prognosis, or response to treatment, and so on. The result in the field of colorectal cancer is the now classic description of the stepwise progression to cancer of Vogelstein and colleagues10 (Fig. 20-1). Such studies were critical to the development of the technical capacity to study mutations and gene expression in a “quantum” fashion. The establishment of cDNA libraries, the cataloging of expressed sequence tags (ESTs) and the use of serial analysis of gene expression (SAGE), subtractive hybridization, and polymerase chain reaction (PCR) techniques remain ways in which molecular biology allows the investigation of a multitude of genes. Aside from the genes listed in Table 20-2, other genes discovered to have pathogenetic roles in colorectal cancer include members of the matrix metalloprotease family of proteins, p16, SMAD4, c-src, phosphatidylinositol-3-kinase, and epidermal growth factor receptor family members, to name but a few.11–17 Individually, some of these genes have been identified as prognostic in terms of survival, response to therapy, presence of metastatic disease, and other clinical characteristics in small retrospective studies. Identification of robust biomarkers for determining cancer prognosis and recurrence remains one of the core goals of genetic profiling.
Microarray Analysis
Microarray analysis of gene expression has developed into a powerful tool for the characterization of many pathophysiologic processes. The basic idea is that RNA isolated from tissue is hybridized to probes for specific genes that are fixed in a grid in small microscopic spots. Figure 20-2 provides a schematic that shows a typical arrangement of a microarray. Depending on the design of the experiment, the signal intensity of the hybridization is normalized to internal controls and other tissues to yield a result for each gene that tells the investigator whether a particular gene has an increased, decreased, or normal expression. The result for the sample, then, is an answer for each gene included in the microarray as to whether the gene is up- or downregulated; the composite data represent a gene expression profile for the sample. When a large number of samples are thus tested, depending on the experimental design, the use of microarrays makes possible the establishment of gene expression profiles for any given disease state, the comparison of subsets to determine molecular predictors of clinical behavior, and so on (Fig. 20-3). As the technology has advanced, the sophistication of microarray analysis has likewise provided increasingly powerful ways of discriminating the molecular characteristics of disease states, including the identification of methylation status of genes (an epigenetic modification of expression) and alternative splicing.18–20
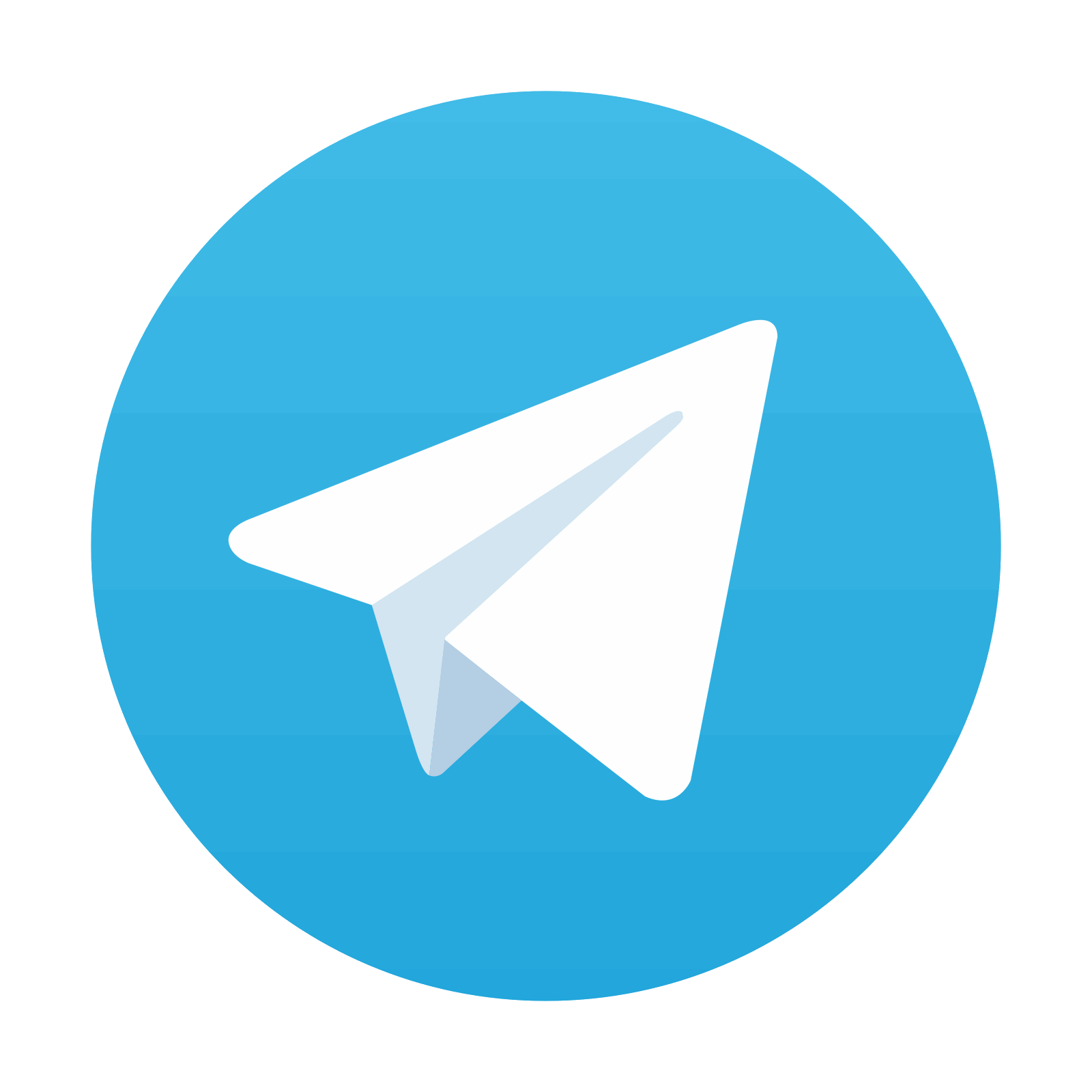
Stay updated, free articles. Join our Telegram channel

Full access? Get Clinical Tree
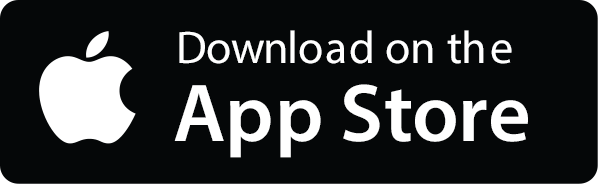
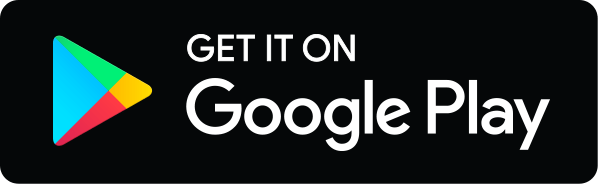