Fig. 14.1
Main environmental and lifestyle factors affecting testicular development and/or function in adulthood
Seasonal Effects on Testicular Function
Though humans are considered to be non-seasonal mammals, we are undoubtedly sensitive to photoperiod [12], as exemplified by ‘seasonal affective disorder’ and by seasonal trends in the frequency of births and in the incidence of twins. Such effects are most obvious in Northern Europe where photoperiodic changes are most extreme [13]. Furthermore, both longitudinal and cross-sectional studies have demonstrated that sperm counts in men are consistently ~30% lower in summer than in spring and/or winter [14–16], though not all studies have reported such effects, and they may be less apparent or absent in tropical countries [17]. An alternative explanation is that exposure to the higher summer temperature is responsible for lowering sperm production (see below), though temperature changes do not account for all of the seasonal trends in births, especially in Northern Europe [12]. If the reported ‘seasonal’ changes in sperm counts/semen quality are an echo from our seasonally breeding ancestors, then this may be rather variable in its effect and, in most men, is unlikely to exert a major effect on their fertility. It is likely that any effects of season in humans/individuals will be driven by seasonal changes in the duration of melatonin secretion, as in seasonally breeding animals (below).
Studies in seasonally breeding animals have established the key pathways via which daylight length is able to regulate the reproductive axis, and melatonin secreted by the pineal gland plays a pivotal role [1, 2]. It is therefore curious that young men with hypogonadotropic hypogonadism (HH) show abnormal blood levels of melatonin, being increased in males with idiopathic HH and decreased in males with Kallmann syndrome, when compared with controls [18, 19]. However, as melatonin levels may be normalized by testosterone replacement therapy in HH men, it implies that the abnormal melatonin level may be a consequence rather than a cause of the HH [18], although this reasoning may not extend to men with hypergonadotropic hypogonadism [19]. More generally, the evidence for seasonal fluctuations in testosterone levels in men is equivocal [20] and is likely to be highly confounded by the influence of other factors such as obesity, activity levels and age.
Occupational Effects on Testicular Function: General Aspects
Numerous published studies have retrospectively surveyed the occupations of men attending infertility clinics and/or compared occupations of fertile and infertile groups. There is some consensus in showing, for example, that farmers/agricultural workers or lorry drivers, painters or welders may be over-represented among infertile men, but overall the findings of such studies are inconsistent and have failed to identify common occupational causes of male infertility [21–24]. Occupation is only one of a range of factors that may cause male infertility, and therefore searching for such factors among patients at the infertility clinic may not be the most sensitive approach. Unfortunately, alternative approaches such as the direct investigation of particular working groups also have various problems [25]. Low participation rates are common and may be biased toward those who have experienced, or suspect, a fertility problem [26]. This makes interpretation of any findings difficult. Another common problem is the low numbers of workers who may eventually compose the ‘exposed’ or control groups, as many published studies involve 30–50 men or less per group [23, 25, 27]. As sperm counts and other semen parameters show great variation between subjects, detection of a workplace/occupational effect against such a background requires considerable numbers of subjects; otherwise, the study will lack sufficient power to detect anything other than a major change in semen parameters [25]. For example, ~80 control and ‘exposed’ men would be required to detect a 20% fall in sperm count. Moreover, because within-subject variation in semen parameters can also be substantial, a rigorous study should involve two or more semen samples per subject, adding considerably to study complexity and cost.
Finally, there are likely to be many confounding factors in any occupational study. These may include age, ejaculatory frequency/abstinence, smoking and alcohol consumption, recreational drug use, time spent seated (see below), recent infection or febrile period, past history of cryptorchidism and sexually transmitted disease [28]. Attempts can be made to control for some of these factors, but with the generally small numbers of subjects involved, this is inevitably less than satisfactory. Against this background, it is therefore unsurprising that relatively few occupations or workplace exposures have been shown consistently to impact significantly on male reproductive health (usually on sperm counts/fertility). However, there is reasonable evidence to suggest that exposure to some solvents [29], older glycol ethers [23, 30] and inorganic lead [31, 32] can impact one or more aspects of semen quality in exposed workers and in some instances affect fertility or miscarriage rates (though effects on the latter two parameters are small). Nevertheless, other studies that showed no significant effect of similar exposures can also be found in the literature [e.g., 33, 34]. This would support the view that such workplace exposures have relatively minor effects on semen quality and male fertility, though in some individuals, such as those with a low sperm count for other reasons, such effects might significantly impact fertility.
Information on occupational impacts on testosterone levels in men is even more equivocal, in part for reasons of study group sizes as just outlined for semen quality aspects. Moreover, any adverse effect on testosterone levels should automatically trigger a compensatory increase in LH secretion that will return testosterone levels toward normal, unless the factor(s) in question exerts its adverse effect via altering secretion of LH. While any such compensatory change should theoretically be detectable via LH measurement and calculation of the LH/testosterone ratio, the inter-individual variation in LH and its pulsatile secretion pattern as well as the circadian rhythm in testosterone levels can make differences difficult to detect unless changes are extreme, which would be unusual. Reasons such as these probably explain why the literature on this topic is small with highly equivocal, and usually very minor, findings. These will not be reviewed in any detail here, but an example is a Chinese study of 154 electrical power-plant workers, half of whom were exposed to electromagnetic fields, which was associated with a 3% average drop (p = 0.015) in total testosterone levels [35]; such a change is likely to be incidental. Where occupation has been associated with altered testosterone levels, it has been mainly in connection with pesticide/fungicide exposure, which is outlined below. A more convincing study involved Italian policemen exposed to traffic fumes [36], compared with police office workers. Those exposed to traffic fumes exhibited ~20% reduction in free testosterone levels, a difference that was even more marked (~29%) when those in the age range 30–40 years were compared [36]. Unfortunately, LH levels and the LH/testosterone ratio were not reported in that particular study, although a similar earlier study by the same authors (N = 166 traffic-exposed vs. 166 non-exposed) reported a mean 50% increase in LH levels (from 1.8 to 2.7 mIU/ml; p < 0.001), although no testosterone levels were reported in that study! [37] Thus, it appears that high exposure to traffic pollutants may induce compensated Leydig cell failure (i.e., decreased or normal testosterone in the face of elevated LH), but this requires direct confirmation in a properly designed study in which both LH and testosterone are measured in the same men. As to mechanism, there is some evidence that diesel exhaust might suppress expression of steroidogenic factor-1 in developmental animal studies [38].
Occupational/Environmental Exposure to Pesticides/Fungicides/PCBs
The occupational exposure associated with the greatest change in sperm count and fertility was workplace exposure to the nematicide, dibromochloropropane (DBCP; Table 14.1). This toxin induced azoospermia or oligospermia in a high percentage of exposed workers, both those involved in its manufacture and those involved in its application on crops [39–41]. In a substantial proportion of DBCP-exposed workers who became azoospermic, no recovery of sperm counts occurred following removal from exposure [39, 40]. This lone example is frequently cited as reassuring evidence of the rarity of such workplace effects on sperm counts and male fertility. In reality this reassurance is a veil. The effects of DBCP were revealed, even though the affected workforces comprised fairly low numbers, because it had catastrophic effects on sperm counts/fertility. We can be reassured that similar catastrophes will also reveal themselves, but more modest effects of occupation on sperm counts/fertility will remain difficult or impossible to identify in all but very large, well-controlled studies. The latter are hugely expensive and laborious, both of which act as strong deterrents to their application.
Table 14.1
Main examples of environmental chemicals that have been studied in relation to potential impacts on spermatogenesis or steroidogenesis in adult men
Chemical type | Uses | Nature of exposure | Possible effects |
---|---|---|---|
Dibromochloropropane (DBCP) | Nematicide | Occupational | Irreversible azoospermia |
DDT/DDE and related pesticides | Pesticides | Food chain and environmental | ?Changes in semen quality and/or LH and testosterone |
Polychlorinated Biphenyls (PCBs) | Electrical equipment + others | Food chain and environmental | ?Changes in semen quality and/or LH and testosterone |
Organophosphates | Pesticide | Occupational | ?Changes in semen quality and/or LH and testosterone |
Pyrethroids | Pesticide | Environmental | ?Changes in semen quality and/or LH and testosterone |
The DBCP experience has had several important repercussions, the most scientifically important being the impetus that it gave to studies of wider pesticide exposure and male reproductive function, especially changes in semen quality and male fertility. Conversely, it has also helped trigger the default view that pesticide exposure results in lowered sperm counts/infertility, which has become more or less accepted as dogma in some circles. There have also been numerous studies of pesticide exposure and hormone levels in men, which is the primary focus of this chapter. However, as there is clear evidence that men with low sperm counts are at increased risk of having compensated Leydig cell failure [42, 43], it makes sense to consider the evidence for pesticide effects on both aspects of male reproductive function together.
There are numerous studies that show significant associations between exposure to various pesticides/fungicides and reduced semen quality (Table 14.1), but there are nearly as many studies showing no significant association. As this is not the primary focus of this chapter, only a brief overview is given here, and readers are referred to a 2008 review [44]. This paper reviewed all studies spanning a 15-year period and reported that only 13 of 20 studies showed a significant negative association between environmental or occupational pesticide exposure and semen quality with similar disparity for other parameters (e.g., sperm DNA damage). When considering individual pesticides or classes of pesticides, the results are in general equally inconsistent, for example, for persistent organochlorine pesticides such as DDT/DDE [45–48]. Recent studies that have focused on other pesticides such as organophosphates [47, 49, 50] or pyrethroids [51] have perhaps shown a more consistent association between exposure and reduced semen quality.
In terms of pesticide exposure and sex hormone levels in men, the perspective is quite similar to that for semen quality, although not as many studies have investigated hormone levels. Thus, reduced blood testosterone levels with or without altered (up/down) LH levels have been reported in men exposed to persistent organochlorine pesticides such as DDT/DDE [52], to organophosphates [53] or pyrethroids [51, 54]. However, for each of these classes of compounds there are comparable studies (mostly larger in size) showing no significant alteration of testosterone and/or LH levels in association with exposure to DDT/DDE or other persistent organochlorine pesticides [55–59], to organophosphates [60] or pyrethroids [61].
The inconsistencies in studies that have assessed the association between pesticide exposure and male reproductive function may be partly attributed to inter-study variation in a range of factors, such as methods, country of study, exposure assessments and outcomes [44]. Perhaps more important may be that many of the studies are based on either male partners of infertile couples or farmers/occupationally exposed men, neither of which is representative of the general male population. It is perhaps noteworthy that the majority of studies unaffected by such limitations have mostly found no association between pesticide exposure and male reproductive dysfunction [46, 48, 58, 61], although there are exceptions [53, 62], bearing in mind also that there will be significant under-reporting of negative data in the published literature. Arguably, the most informative study was the US LIFE study which was prospective and involved 501 male partners of couples who were just stopping contraceptive use, and in whom exposure to 36 PCBs, 9 organochlorine pesticides and 10 polybrominated diethyl ethers was measured [48]. Although a range of statistically significant associations was found between exposure to individual chemicals or classes of chemicals and semen quality, most of the associations were positive not negative. In this study, as well as in all of the others cited, it should be emphasized that where significant associations have been found between exposure(s) and semen quality or testosterone levels, the ‘effect’ sizes, whether negative or positive, have been generally small and could be incidental. The tentative conclusion, based on present data, is that for the general population at current levels of pesticide exposure, there is unlikely to be any biologically meaningful effect on adult male reproductive function although there may be some risk for those who are more highly exposed via their occupations, such as farmers.
Occupational/Lifestyle Effects on Scrotal Temperature
Another situation in which occupational effects on male reproductive function might become apparent is if the ‘occupational effect’ applies to a large proportion of men; ‘scrotal heating’ may be one such example. Scrotal, and therefore testicular, temperature has to be maintained some 3–4 C lower than core body temperature if normal spermatogenesis is to occur, and this is achieved via a vascular heat exchanger system, the pampiniform plexus (Fig. 14.2). Interference with the normal scrotal cooling process can profoundly affect sperm production, quality and fertility [63–65], although there is no clear evidence for effects on testosterone levels. A straightforward example of this is the effects of repeated Finnish sauna exposure over 3 months, which lowered sperm counts and motility and induced adverse effects to sperm chromatin packaging but did not measurably alter testosterone levels; all of the adverse effects of the sauna were reversible within 6 months [66]. In theory, occupational or lifestyle factors that interfere with scrotal cooling/thermoregulation could be significant factors in lowering sperm counts [66, 67]. Examples of such potential factors are exposure to radiant heat, the wearing of tight trousers/underwear, time spent having hot baths and time spent seated (Fig. 14.2). Most of the older studies that sought to investigate such factors could rely only on establishing an association between such factors and poor semen quality, and perhaps not surprisingly the evidence proved equivocal or only marginally convincing in most cases [reviewed in ref. 25]. However, several epidemiological studies have shown that occupations involving prolonged sitting, and thus reduction in airflow around the scrotum [63, 66], are associated with significant reductions in sperm counts. An example is car or taxi driving [68, 69]. Away from occupational situations, the worst case might be paraplegic men who are confined to wheelchairs [70], although the poor semen quality in such men may have other contributory causes such as impaired accessory sex gland function, infection and increased proinflammatory cytokines [71].


Fig. 14.2
Schematic diagram to illustrate the central mechanisms involved in cooling of the testis, and the various occupational or lifestyle factors that can interfere with their function. The primary mechanism of cooling the testis is by heat loss from the scrotum, and this necessitates air flow around it. The resulting cooling of the testis means that testicular venous blood is also cool when it leaves the testis and this blood is used to cool incoming warm arterial blood within the pampiniform plexus via heat exchange and via the diversion of ~40% of incoming arterial blood into the testicular vein via numerous arterio-venous anastomoses; the presence of a varicocele may interfere with heat exchange within the pampiniform plexus
More objective studies became possible once the technical problems relating to measurement of changes in scrotal temperature had been overcome, as this enabled quantification of scrotal heating. Scrotal temperature was measured continuously in normal young men or in men from couples planning their first pregnancy, and a significant relationship between average daily scrotal temperature and sperm counts was established [72]. Moreover, the same studies linked scrotal temperature to the amount of time spent seated, i.e., the more sedentary the lifestyle/occupation, the higher the average scrotal temperature, and this has been confirmed in more extensive recent studies [73]. Application of continuous scrotal temperature monitoring in reasonably large numbers of healthy men has demonstrated unequivocally that increase in average scrotal temperature is clearly linked to lower sperm counts as well as to associated changes in blood levels of FSH and inhibin-B [72–74]. Such findings raised the expectation that our increasingly sedentary lifestyles in the West might induce negative effects on semen quality in a high proportion of men [25], but this expectation is proving to be overly simplistic. For example, although time spent seated is an important determinant of scrotal temperature, the former has only a weak relationship with semen quality [74] and fertility [75], and other factors such as obesity [76], night-time scrotal temperature [73] and genetic determinants of scrotal temperature [77] may prove to be more important. There is reasonably strong evidence that physical activity is associated with better semen quality [75, 78] and marginally higher testosterone levels [78], when compared to men who have sedentary lifestyles. While this may be related to lower scrotal temperature, as physical activity is likely to improve scrotal cooling, there may be other effects of physical activity that also impact testis function.
From a clinical perspective, the number of men in whom infertility is induced partly or totally by elevated scrotal temperature is likely to be low. However, there are three other points to be kept in mind. First, even quite mild elevations in scrotal temperature in animal studies can increase DNA damage and miscarriage rates [64, 79], so its effects on sperm can be qualitative as well as quantitative. Second, ‘treatment’ to lower overall scrotal temperature in patients who have poor semen quality, though being a ‘shot in the dark,’ is relatively noninvasive and can be quite effective in some cases [80]—and there are no side effects! The discovery that there are genetic determinants of scrotal temperature [77] also reinforces the possibility that some individuals may be predisposed to adverse effects of prolonged seating or radiant heat on semen quality. While such individuals cannot yet be recognized, careful attention to lifestyle factors to minimize scrotal heating in all men with fertility problems is therapeutically benign and can have only beneficial effects. Third, the well-established, if inconsistent, effect of a varicocele on semen quality in men, may itself induce such effects by interfering with normal cooling of incoming arterial blood in the pampiniform plexus [76] (Fig. 14.2), and it is possible that such an effect might be exacerbated by the time spent seated or make such individuals more susceptible to effects of radiant heat than men without a varicocele.
Other Lifestyle/Dietary Factors Affecting the Adult Male
The preceding section dealing with scrotal temperature obviously applies as much to sedentary lifestyles outside, as well as inside, of the workplace. Probably, the most important other factors are dietary habits, in particular obesity and alcohol intake, smoking, stress and recreational/sporting drug use.
Unlike the well-established relationship between calorific intake and maintenance of menstrual cycles in women, there is no such straightforward relationship in men regarding sperm counts. Underweight and overweight men both have a slightly increased risk of having low sperm counts, though there is considerable variation between studies, especially with regard to obesity [81, 82]. Moreover, there is only limited evidence that it is obesity per se that causes any semen quality changes (Fig. 14.3). Thus, although a 14-week residential weight loss program in 43 severely obese men was shown to increase semen volume and total sperm count [83], similar studies with small numbers of obese men undergoing bariatric surgery-induced weight loss have so far failed to find consistent benefits, and some studies even point to deleterious effects [84]. Nevertheless, the evidence that male obesity can negatively impact fertility is convincing. For example, obesity is over-represented among infertile men [80, 81], and in the setting of assisted reproduction, male obesity is associated with reduced embryo quality and pregnancy rate in the absence of any significant changes in semen quality [85]. Indeed, a recent meta-analysis of 30 studies (n = 115, 158 men) reported that male obesity was associated with a 66% increase in risk of infertility and a 35% reduction in live birth rate per cycle of assisted reproduction in the absence of significant differences in conventional semen parameters [86]. The underlying cause of obesity-related male infertility remains unclear and is likely to be multi-factorial [87]. However, there is compelling evidence emerging that points to changes in fatty acid composition of semen and sperm as being important [88, 89], and both human [90] and animal [91] studies provide evidence that the fat composition of our diets may be important in determining this and affecting semen quality (Fig. 14.3). Thus, it may be specific dietary factors in obese men that is important in relation to changes in semen quality and/or fertility, and that therefore not all obese men will be affected.


Fig. 14.3
Schematic representation of the major reproductive changes in men that are associated with obesity and current thinking as to what may be the key causal factors and pathways
In contrast to the equivocal data on semen quality, the evidence that visceral obesity in men is associated with a fall in total testosterone levels in both young [92] and older [93] men is much more compelling (Fig. 14.3), and it is now widely accepted that visceral obesity and its associated metabolic changes invariably impact testosterone levels, encapsulated in the metabolic syndrome [94]; however, metabolic syndrome-associated changes in testosterone levels are dissociated from any change in semen parameters [95]. The primary mechanism via which obesity/the metabolic syndrome reduces testosterone levels is via suppression of pituitary LH secretion, but the mechanisms that underpin this are complex and incompletely known, but certainly involve changes in estradiol and sex hormone-binding globulin levels [96, 97] (Fig. 14.3). These interplay with the effects of adipokines, such as leptin and adiponectin, which may act both centrally [97, 98] or directly on the testis [99], although a recent systematic review suggests that direct testicular effects are probably of minor importance in comparison with central effects [99]. As other chapters in this book deal with these aspects, in particular the relationship of hypogonadism to the metabolic syndrome, they will not be discussed further here. However, there is one emerging aspect that has been hitherto overlooked, and which may turn out in the long term to be an important player, the gut microbiome.
There are various lines of evidence that the gut microbiome can affect male reproductive function, although the number of studies is presently too few to enable assessment of how important this might be [100]. However, its potential importance is highlighted by a series of intervention studies in mice, which have shown that feeding them from 8 weeks of age (young adulthood) with the probiotic species Lactobacillus reuteri was able to protect the mice against diet-induced, age-related obesity [101]. However, far more interesting was that this intervention completely prevented the age-related development of impaired spermatogenesis, reduced testis weight and hypogonadism [102], although until such studies are repeated, it is too early to conclude that similar effects could apply to man. The protective effects of the probiotic treatment were equally apparent in mice maintained on either a standard (control) diet or maintained on a ‘Western style diet.’ A series of studies by these authors have shown that the beneficial effects of the probiotic feeding regime appear to result from modulation of specific proinflammatory cytokines, such as interleukin-10 and interleukin-17A [102, 103]. As obesity/metabolic syndrome and hypogonadism are linked intrinsically to a more proinflammatory cytokine profile in blood in men [94, 104], it raises the interesting possibility that such health-relevant changes could be ameliorated either via probiotic supplementation or by dietary changes that alter the gut microbiome, an area richly deserving of further study.
Smoking by men sometimes emerges from epidemiology studies as a risk factor for low sperm counts or altered sperm morphology, but this is an inconsistent finding and ‘effect’ sizes are small [105]. Conversely, smoking is consistently associated with increased rather than decreased total and free testosterone levels, the mechanism for which is uncertain [106, 107]. As outlined below, smoking by mothers during pregnancy is associated with a consistent and substantial (20–40%) reduction in sperm counts in resulting male offspring in adulthood [25], whereas testosterone levels are unaffected [108]. In a large population-based cross-sectional study of >8000 young men, moderate alcohol intake was not associated with any change in semen quality, but was positively associated with free testosterone levels [109], whereas heavy alcohol intake is associated with lowered total testosterone levels [110].
Psychological stress arising for various reasons is usually associated with reduced semen quality with effects both on sperm count and motility, and testosterone levels are also usually lowered [111, 112]. The mechanisms behind such changes involve a complex interplay at various levels between the hypothalamic–pituitary–adrenal and hypothalamic–pituitary–gonadal axes; among these, direct inhibitory effects of adrenal cortisol on testicular testosterone production and indirect effects via cortisol-induced decrease in SHBG levels are probably important [111]. It should be noted, however, that much of the available data stem from experimental animal studies, as studies in men are much more difficult to control for confounding factors [111].
Though Western males are generally less physically active today than 30 or so years ago, for those who do participate in sports, it is far more competitive and this has led to usage of performance-enhancing drugs, the most common being anabolic androgenic steroids [113]. Outside of sports, there has been an explosion in use of the same drugs in pursuit of improving physique and body image [113]. Such use is now considered to be perhaps the single most important determinant of hypogonadism in young men [114], although this will be dependent on factors such as type of steroid, dose administered and perhaps duration of administration [115]. Although much of the anabolic steroid use is clandestine, clinicians faced with athletic young men with hypogonadism should always bear this possibility in mind [113, 115]. Similar awareness should be practiced for other non-steroidal drugs, as we live in an age when use of a range of ‘recreational’ drugs is widespread. For example, 25% of young American men report using marijuana within the last month [116], and 45% of Danish young men within the past 3 months [117]. In the latter study, which was a population-based study (N = 1215), regular weekly use of marijuana was associated with ~30% decrease in sperm count, and if marijuana use was combined with other recreational drugs, the decrease was >50% [117]. Considering the scale of marijuana/other recreational drug use, these statistics suggest that this may be a very important determinant of low sperm counts in young men. As has been found with tobacco smoking, marijuana use is associated with a significant increase in total (but not free) testosterone levels [117]. In contrast, use of opiate-based drugs by young men is associated with a uniform and quite substantial decrease in testosterone levels, based on a meta-analysis of 17 studies [118].
Finally, vigorous exercise such as long-distance running may result in temporary suppression of testosterone levels in men and minor decrements in semen quality, though the changes are by no means as pronounced as the anti-reproductive effects that can occur in female athletes [119, 120]. In contrast, less severe exercise generally has no effect on testosterone levels [120] (see Chap. 13).
Environmental/Lifestyle Effects on the Adult Testis that Arise During Fetal Development
Testicular Dysgenesis Syndrome (TDS) and the Masculinization Programming Window
There is growing evidence that a syndrome of inter-connected disorders affecting the human male, so-called TDS, may have a common origin in fetal life during the period of masculinization [4, 6–8]. Manifestations of this syndrome in adulthood can include low sperm counts/reduced fertility and/or testicular germ cell cancer as well as a history of cryptorchidism and/or hypospadias (Fig. 14.4)—recent evidence suggests that lowered testosterone levels or compensated Leydig cell failure could also be a part of TDS [43, 121]. Some of these disorders appear to be increasing in incidence, with environmental/lifestyle causes implicated in this increase [4, 6]. An integral part of this syndrome of disorders is evidence for impaired testosterone production/action [7, 8], during a specific fetal period termed the ‘masculinization programming window’ (MPW; Fig. 14.4) [7]. This period has been shown in animal studies to be the critical period when sufficient fetal androgen action is required to program later development of all male reproductive organs, including their ultimate size [7, 8, 122, 123]. The available evidence suggests that an MPW also exists in humans and probably lies within the period 8- to 12-weeks gestation [7, 8], and based on the animal studies and emerging human data, the level of androgen exposure during the MPW could be deduced at any age by measurement of the anogenital distance (AGD) [7, 8].


Fig. 14.4
Schematic diagram to illustrate the cellular basis and general pathways via which the disorders that comprise ‘testicular dysgenesis syndrome’ (TDS) are thought to arise in the human. Abnormal testicular cell differentiation/function (=dysgenesis) appears to be an integral part of this syndrome of disorders, but several causal pathways might lead to this occurrence, including genetic, environmental and/or lifestyle factors. Note also that the resulting disorders that arise because of TDS occur with differing frequency, varying from quite common (poor semen quality, cryptorchidism) to rare (testicular germ cell cancer). Note also that some of the disorders may occur for reasons other than TDS (e.g., cryptorchidism, hypospadias) or because of effects after birth/in adulthood (e.g., poor semen quality). TDS disorders are thought to arise primarily as a consequence of, or associated with, subnormal testosterone production and/or action within a discrete fetal time window, termed the ‘masculinization programming window (MPW),’ which is reckoned to occur somewhere within the time period of 8- to 12-weeks gestation. In theory, androgen exposure within the MPW can be determined retrospectively by measurement of anogenital distance (AGD), though in practice such use may have limitations (see text)
As a result of the discovery of the MPW and the emerging credibility of the TDS hypothesis, the focus has shifted toward trying to identify what factors, acting via the pregnant mother, might adversely impact fetal testis testosterone production, a task that is severely constrained because of the inaccessibility of the human fetus (it is only a few cm in length during the MPW) and because fetal androgen production cannot be monitored directly [8]. Therefore, most of the available evidence derives from epidemiological studies that have looked for associations between occurrence of ‘TDS disorders’ (e.g., cryptorchidism, hypospadias) and specific maternal exposures or lifestyle factors. This literature is too extensive to discuss in detail here, so just the aspects that are most directly relevant to this chapter are presented. Further details and reference trails can be found elsewhere [4–8].
It is first worth making some general points, in particular to remind readers of just how common are the disorders that may comprise the TDS. For example, the two most common congenital malformations in children of either sex are both TDS disorders, namely cryptorchidism and hypospadias, which affect 2–9 and 0.3–1.0% of boys at birth, respectively [4, 6, 8]. This is not to imply that all cases of cryptorchidism and hypospadias are part of TDS, because they may arise as a consequence of other deficiencies/syndromes which might not be directly connected to TDS. One way in which some potential discrimination might be applied is via measurement of AGD, because if AGD is abnormally small, it would imply that there had been androgen deficiency/resistance during the period of the MPW, so that the case in question could be classified as likely to be TDS. Where this has been done for cases of cryptorchidism and hypospadias and reference made to population-based normal data for AGD in one particular center, it is clear that the majority (but not all) of cases of cryptorchidism and hypospadias have AGD measurements below the normal 50th centile [124]. Therefore, based on AGD it may be possible to at least putatively identify possible TDS and non-TDS cases, at least for cryptorchidism and hypospadias. Reduced AGD has also been associated with androgen receptor polymorphisms such as longer CAG repeat length, which impairs androgen signaling [125].
Extending the same line of thinking to adult-onset cases of suspected TDS, namely those with low sperm counts, low testosterone/compensated Leydig cell failure or testicular germ cell cancer, has only so far been done in a superficial way, and only in relation to sperm count [8]. The available studies support the view that men with lower sperm counts and/or infertility have an associated shorter AGD [126–129], although a recent Chinese study did not find this [130]. It seems unlikely, however, that AGD could be used clinically in any diagnostic or predictive way, because of considerable inter-individual variation and confounding by body size and adiposity [8, 131, 132].
Of more direct relevance to the present chapter is the potential association of AGD with blood testosterone levels and Leydig cell function. There is a single human [127] and a single experimental rat [122] study showing a similar magnitude, positive association between AGD and adult blood testosterone levels. However, several other pieces of data support a role for prenatal events, including fetal androgen exposure, as a determining factor for adult Leydig cell function. First, birth weight has been shown to be positively associated with blood testosterone levels in adult men across the full birth weight spectrum [133], and this is independent of adult obesity/BMI, which can itself be a determinant as discussed earlier. Second, a population-based study of >8000 European young men showed that low sperm counts, a TDS disorder that occurs in ~20% of young men [134], are not associated with any change in blood testosterone levels but are associated with compensated Leydig cell failure [43]. Evidence that such a change could emanate from fetal life as a consequence of subnormal fetal androgen action on adult Leydig stem cells, comes from an experimental animal study showing that compensated adult Leydig cell failure is induced in rats and mice when fetal androgen production/action is reduced [121]. The human health significance of compensated Leydig cell failure is not clear at present, although one line of thinking is that it might identify a subset of men whose Leydig cells may ‘wear out’ during aging due to their compensated ‘over-functioning,’ which might then lead to frank hypogonadism and its attendant morbidities. However, frank hypogonadism is not a very prevalent feature among aging men, whereas ~10% exhibit compensated Leydig cell failure [135].
An indirect but powerful argument in favor of environmental factors impacting testosterone levels in men is the evidence from three independent studies showing that the more recently a man has been born, the lower will be his testosterone level at any age [136–138]. This implies that something new in our lifestyle or exposures is exerting an adverse effect on testosterone levels in men, the most likely explanation being the increase in adiposity and its associated lowering of testosterone levels [92–94]. However, specific dietary factors and/or chemical exposures could be contributory factors, including those occurring in fetal life (via the mother). Unfortunately, it seems unlikely that it is feasible to design studies that could address the latter possibility in a meaningful way.
What Maternal Lifestyle Factors or Exposures Might Impact Reproductive Development/Androgen Exposure of the Male Fetus?
Of necessity, all studies that have addressed this question in human pregnancy are association studies. By far the largest and most consistent finding is that smoking by mothers during pregnancy is associated with reduced testis size and sperm counts in resulting male offspring [reviewed in ref 25]; the reductions reported are substantial, ranging from 20 to 40%. The mechanism underlying the presumed effect is unknown but is presumed to reflect a smoking-induced reduction in Sertoli cell number [25]. Maternal smoking has not been associated with any obvious change in adult male testosterone levels [108], although this has not been studied in as much depth as sperm counts. More recently, experimental evidence has emerged to suggest that protracted use of acetaminophen and/or other painkillers in early pregnancy might reduce testosterone production by the fetal human testis [139, 140]. This finding is supported by the fact that 4 independent epidemiological studies have shown a significantly increased risk (~twofold) of cryptorchidism in sons of mothers who reported protracted use of acetaminophen during pregnancy [139, 141–143]. Whether acetaminophen use in pregnancy is associated with adult-onset TDS disorders, and whether it might predispose to development of compensated Leydig cell failure have not been studied yet and will prove challenging to investigate given the time gap between exposure and sought for ‘effects.’ Nevertheless, this possibility is in urgent need of investigation as the majority of women report use of acetaminophen and/or other painkillers in pregnancy [144].
The issue of environmental ‘endocrine-active’ chemicals has received enormous attention over the past few decades, and there is widespread belief that such compounds do impact human health, and in particular male reproductive health; such effects are presumed to be mainly during fetal or early postnatal life and thus to affect the developing testis. The initial focus was on ‘environmental estrogens,’ because if pregnant rats or mice are exposed in utero to high levels of potent exogenous estrogens, such as diethylstilbestrol (DES) or ethinyl estradiol, testosterone production by the fetal testis is reduced by 80–90%, a change that will lead to irreversible impairment of masculinization and development of the male reproductive system [145–147]. However, there have been two important research developments which strongly suggest that, at least as far as the developing fetal human testis is concerned, environmental estrogenic chemicals are unlikely to pose any significant risk, irrespective of their estrogenic potency or the level of exposure. First, evidence from both in vitro cultures [148] and ex vivo xenografts [149] of human fetal testes into nude mice demonstrates that even after exposure to very high doses of DES, no inhibition of testosterone secretion is evident, whereas exposure of rat and mouse fetal testes to the same estrogen doses profoundly suppresses testosterone production [146, 147]. This dramatic difference in effect is presumably explained by the absence of ESR1 (estrogen receptor-α) from human fetal Leydig cells, whereas it is present in fetal rodent Leydig cells [146, 149]. Second, detailed studies by Fowler and colleagues of the human fetal testis have shown that it has enormously high levels of bioactive estrogens, far higher than in the fetal human ovary and ~1000 times higher than the already extremely high levels in maternal blood [150]. Why there should be such high bioactive estrogen levels in the fetal human testis is not known, but in their presence it makes sense to not have any endogenous system that would allow estrogens to suppress fetal Leydig cell steroidogenesis. Thus, rodents are not a good model for the human when considering whether or not estrogenic chemicals pose any threat to the steroidogenic function of the human fetal testis.
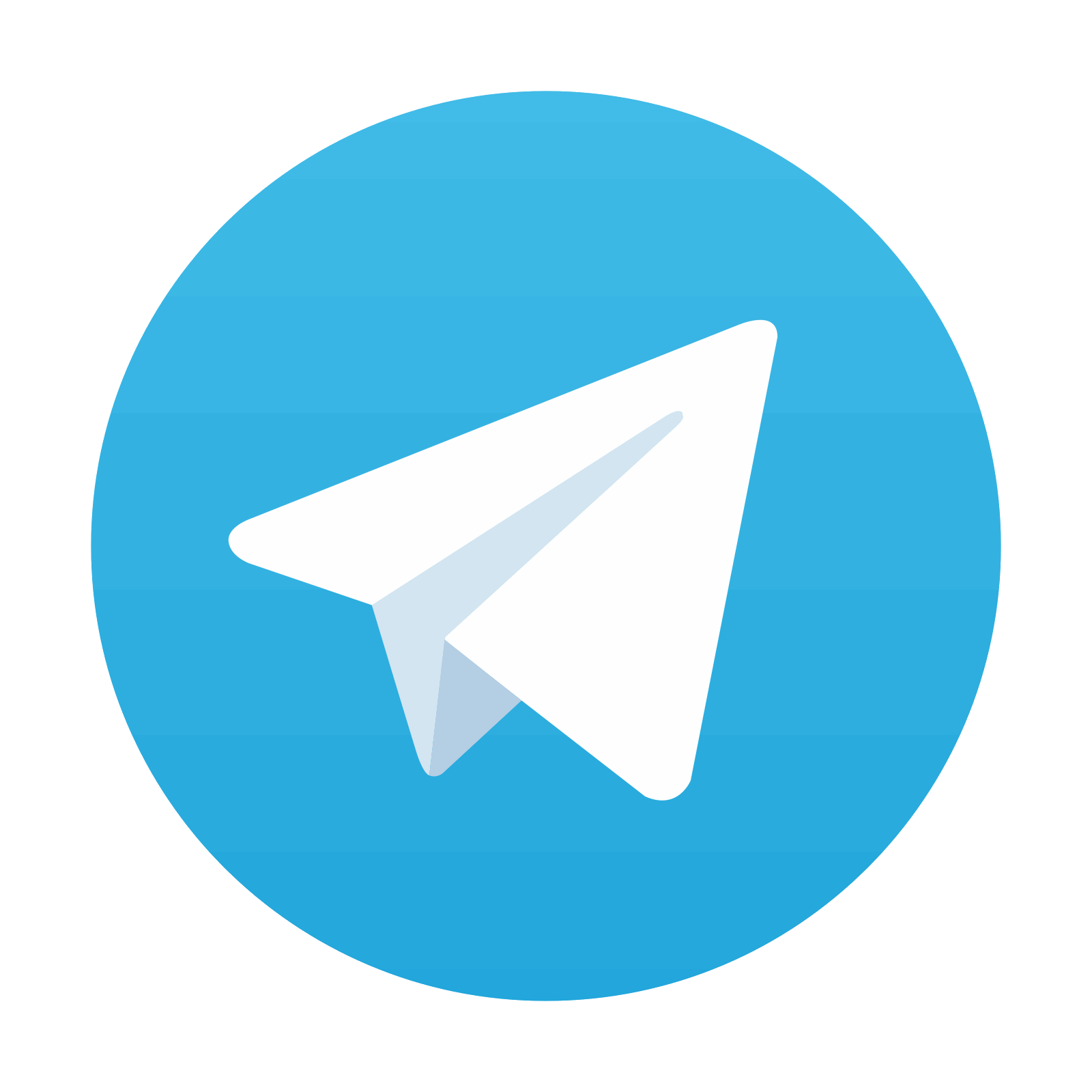
Stay updated, free articles. Join our Telegram channel

Full access? Get Clinical Tree
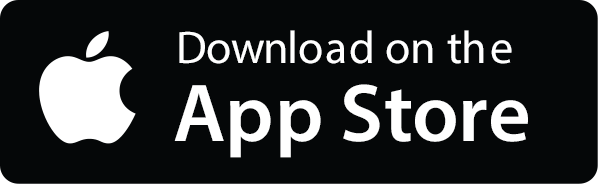
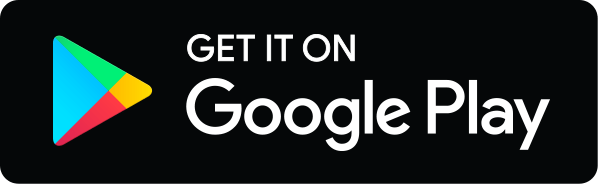