Fig. 16.1
Simplified schema of the GnRH-LH-testosterone (hypothalamo-pituitary-Leydig cell) axis with feedback (−, inhibitory) and feedforward (+, stimulatory) interactions mediated via specific interface (dose-response) functions
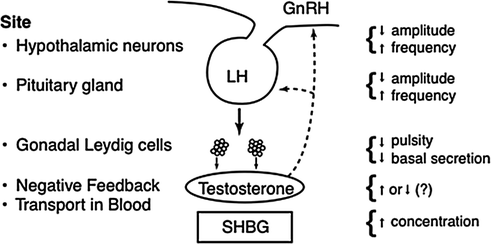
Fig. 16.2
Primary loci of postulated regulatory defects in the GnRH-LH-testosterone ensemble axis in aging men
Role of Intrinsic GnRH Deficiency in Mediating Low-amplitude LH Secretion
Experiments in the male mouse and rat support an important (but not exclusive) role of GnRH-deficient hypogonadotropism in the hypoandrogenemia of aging [48–51]. Evidence to this end includes: (i) decreased castration-, naloxone-, and restraint stress-induced LH release in the older rodent [30, 49, 52–54]; (ii) diminished in vivo LH pulse amplitude in senescent animals [50, 55]; (iii) reduced in vitro hypothalamic GnRH secretion [56, 57]; (iv) altered GnRH neuronal synaptology [58]; (v) preservation of the stimulatory efficacy of GnRH [50, 59]; and (vi) restoration of sexual activity in the impotent animal by fetal hypothalamic neuronal transplantation [60]. Although such observations afford important clues for clinical investigation, the regulation of GnRH outflow in the laboratory animal is complex, multifactorial, incompletely defined, and not necessarily equivalent to that of the human male.
The intuition that hypothalamic GnRH deficiency subserves relative hypogonadism in elderly men has not been established or refuted [28, 32, 34, 61–64]. However, the notion is congruent with many clinical observations, as highlighted in Table 16.1. A necessary (but not sufficient) prediction is that GnRH action on gonadotropes is preserved. A recent prospectively randomized, interventional study used 14 days of pulsatile, i.v., infusion of GnRH versus saline to stimulate LH and testosterone secretion. Older and young men treated with this regimen achieved an equivalent elevation of 24-h LH concentrations (Fig. 16.3a) consistent with retention of gonadotrope secretory responsiveness in the older male [34]. In young men, the increment in mean LH concentrations elicited a proportionate rise in testosterone production. On the other hand, the same average increase in LH immunoreactivity in aged individuals failed to augment total (Fig. 16.3b) as well as bioavailable and free testosterone concentrations equivalently. The latter age-related contrast would point to an abnormal pulsatile LH signal (of equivalent mean concentration only), relatively defective Leydig-cell responsiveness and/or accelerated testosterone metabolism in older men. The last (kinetic) consideration is excluded by the age-related fall in the metabolic clearance rate of testosterone that is partly due to higher SHBG concentrations [40, 42, 43, 65].
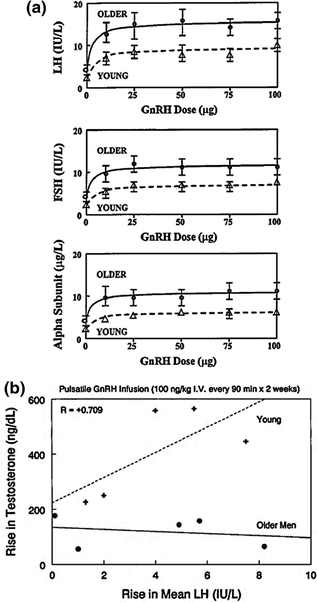
Table 16.1
Indirect evidence for impaired GnRH drive in the aging male
Human | Rodent | |
---|---|---|
1. Low-amplitude spontaneous LH pulses | ||
2. Normal or increased LH secretory responsiveness to, i.v., GnRH pulses | ||
3. Blunted unleashing of pulsatile LH secretion by sex-hormone depletion, opiate-receptor blockade, androgen receptor blockade or stress | ||
4. Age-comparable LH bioactivity | ||
5. Quantifiably disorderly LH release | ||
6. Asynchrony of neurohormone outflow | ||
7. Mathematical ensemble predictions |
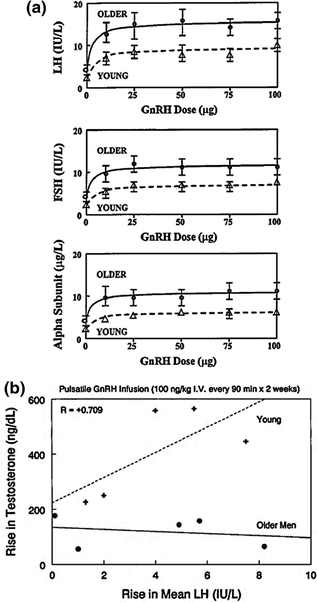
Fig. 16.3
a GnRH dose-response stimulation of (2-h mean) serum concentrations of LH (top), FSH (middle) and free (uncombined) alpha subunit (bottom) in young men (interrupted lines) and older volunteers (solid lines). Data are the mean ± SEM. Adapted with permission from [113]. b Relationship between the increment in 24-h mean concentrations of LH (x axis) and testosterone (y axis) induced by 14 days of uninterrupted pulsatile, i.v., GnRH infusion (100 ng/kg every 90 min) compared with saline in young men (upper interrupted curve and plus signs). There is no correlation in older men (lower continuous line and solid circles). Adapted from [34]
These earlier findings have been verified in a complementary experiment wherein 18 healthy men aged 23–72 years underwent graded suppression of LH secretion through four escalating doses of ganirelix (0, 0.1, 0.3, and 1 mg/m2), a specific GnRH receptor antagonist [66]. Sixteen hours after subcutaneous administration of ganirelix, a submaximal dose of intravenous GnRH (100 ng/kg) was injected, and blood sampling was continued for a further 3 h. Increasing age attenuated the ability of ganirelix to decrease endogenous pulsatile LH secretion, but did not impact competitive interaction between ganirelix and injected GnRH. These joint outcomes suggest that age diminishes GnRH outflow without impairing GnRH action in healthy men. Further modeling confirmed that advanced age was associated with lower GnRH efficacy normalized to free testosterone and also revealed potentiated (maximal and graded) negative feedback by testosterone on hypothalamic GnRH secretion [67].
Contribution of Primary Leydig-Cell Steroidogenic Failure
Anatomical studies document an attrition of Leydig-cell number in the older male [68]. From a functional perspective, pharmacological stimulation with hCG does not induce maximal young-adult concentrations of testosterone in many elderly men [24, 28, 69–72]. Nonetheless, current hCG paradigms are difficult to interpret on experimental and physiological grounds; for example, (i) unequal concomitant (endogenous) LH concentrations among individuals may confound testicular responses to hCG [13, 24, 30, 71, 72]; (ii) the half-life of circulating hCG is approximately 20–30 h compared with 0.75–1.5 h for LH; indeed, the former kinetics abrogate normal intermittent stimulation of the testis [45, 73, 74]. This distinction is relevant, since each pulse of LH normally evokes a prompt burst of testosterone release, as monitored directly in the human spermatic vein [75, 76]; (iii) the nearly irreversible binding of hCG to the gonadal LH receptor downregulates steroidogenesis in vitro and in vivo [77, 78]; and (iv) the magnitude of hCG drive limits clinical evaluation to maximal Leydig-cell, responsivity. An equivalently sustained and potent lutropic stimulus is never achieved physiologically in vivo, except under maternal hCG exposure in the developing fetus [40, 42].
A second means of appraising Leydig-cell responsiveness is cross-correlation analysis of LH and (time-delayed) testosterone concentrations in paired hormone time series [79]. Comparisons by age disclose a young adult-like time lag of 30–40 min but significantly reduced feedforward coupling between LH and testosterone in older men. The latter distinction is reflected in a 50% reduction in the cross-correlation coefficient [33, 35].
A third experimental strategy to examine Leydig-cell steroidogenic capacity is to downregulate gonadotropin secretion with a GnRH analog and then add back pulses of rh LH, i.v., to emulate physiological patterns of gonadotropin stimulation. In this paradigm, LH withdrawal enforced by pituitary down-regulation impairs testosterone secretory responsiveness profoundly in both age groups, albeit twofold more in elderly men [80].
A fourth-interventional model (Fig. 16.4) is to administer a maximal dose of a selective GnRH receptor antagonist (ganirelix) to suppress all endogenous LH secretion, and then infuse successive, i.v., pulses of rh LH to replicate normal LH on testosterone physiology [81–84]. Using this paradigm, pulsatile recombinant human (rh) LH infusions for 48 h designed to mimic both the pattern (every 2 h) and extent of young male LH secretion revealed lower mean and incremental and interpeak testosterone concentrations in 8 older (aged 60–73) compared with 13 young (aged 19–30) men [82]. These age relationships were confirmed in 15 healthy men aged 22–78 years across a tenfold range of amplitude varying, and not supraphysiological, pulses of rh LH administered across a 20-h period [83]. A further analysis of 92 healthy men aged 18–75 years who received various combinations of wide-ranging but not supraphysiological pulses of rh LH show that aging attenuates LH efficacy and testis sensitivity as well as augments Leydig cell downregulation [84].
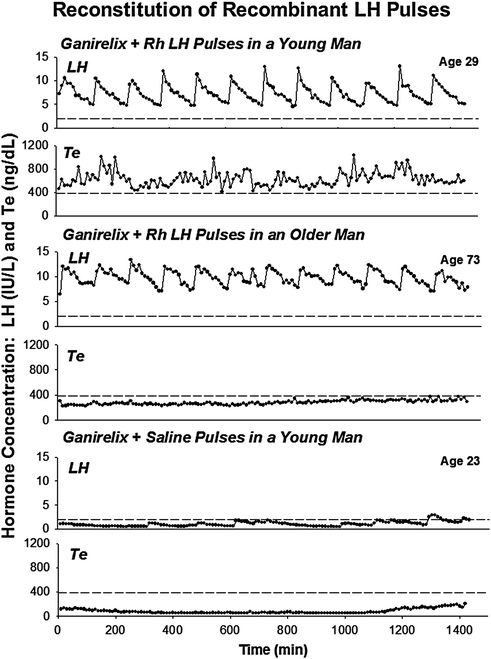
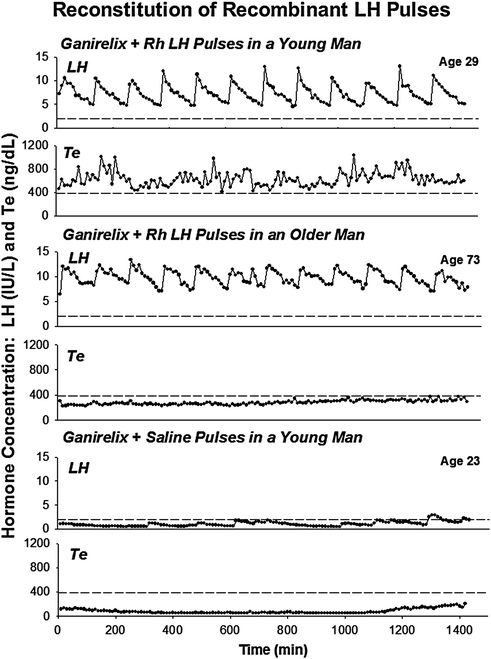
Fig. 16.4
Illustrative profiles of (paired) LH (top) and Te (bottom) concentrations sampled every 10 min for 24 h in a healthy young (upper) and older (middle) man on the second day of pulsatile iv infusion of rh LH and concomitant ganirelix administration [see text]. For comparison, LH and Te concentrations during ganirelix administration and iv addback of saline in a young subject are also shown (lower). Interrupted horizontal lines mark the lower 2.5% normal bounds of LH (2 IU/L) and Te (385 ng/dL) concentrations in young adults. Multiply Te values in ng/dL by 0.0347 to obtain units of nmol/L
Alterations in Testosterone Feedback Restraint
Whether or how sex-steroid negative feedback is altered in older men is controversial. For example, three clinical studies report heightened negative feedback by exogenous androgens in the aging male. These analyzes were based on short-term, i.v., infusion of testosterone or dihydrotestosterone (over days), longer term transscrotal delivery of testosterone (for 11 months), and transdermal supplementation with 5 alpha-dihydrotestosterone (for 15 months) [39, 44]. Two other investigations describe reduced androgen feedback efficacy in the older male after several weeks of intramuscular injection of a high dose of testosterone [61, 85]. Using a novel matched testosterone addback paradigm, experiments showed that aging may impair both positive and the negative actions of systemic testosterone on pulsatile LH secretion [86]. Specifically, stepwise testosterone repletion across the hypogonadal to eugonadal range in 23 men aged 19–71 years revealed that age attenuated both the ability for increasing testosterone concentration to increase LH secretory burst mass and to decrease LH secretory burst frequency.
Two interventional studies using an estrogen- or androgen-receptor antagonist, and one biomathematical construct, infer reduced LH secretory adaptations to muted sex-steroid feedback in elderly men [63, 87, 88]. Recent studies show that aging mutes unleashing of LH secretion by androgen-receptor blockade using a fourth-generation biomathematical construct [89, 90]. From another vantage, cross-correlation analysis of 24-h (paired) LH and testosterone concentration profiles identifies impaired negative feedback by endogenous androgen in older volunteers [35]. And based on immuno-cytochemical detection, androgen (and estrogen) receptors may decline in the brain and pituitary gland of aged rats, and in genital fibroblasts of older men [91, 92].
Prominent adaptations to experimental androgen depletion in young men are accelerated frequency and reduced incremental amplitude of LH pulses (Fig. 16.5a) and quantitatively irregular patterns of LH release [88, 93–95]. The neuroendocrine phenotype in older men includes each of the foregoing feedback adjustments [33, 34, 36, 37, 62]. Thus, a facile hypothesis is that lower bioavailable and free testosterone concentrations in the elderly male are the proximate cause of unleashing anomalous LH secretion patterns. However, quite unlike the feedback-deprived state, basal, pulsatile, and total LH secretion remain normal in many healthy older men [45, 46] (Fig. 16.5b). Accordingly, an important but unresolved mechanistic issue is how neuroendocrine disturbances in aging individuals reflect expected feedback withdrawal due to hypoandrogenemia [43]. This question becomes important, inasmuch as testosterone repletion in androgen-deficient young men reverses each of rapid-frequency, diminutive-amplitude, and disorderly LH secretion [88, 93, 94].
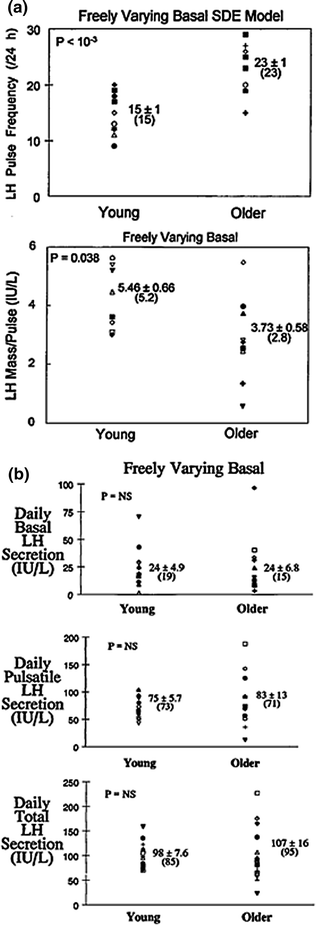
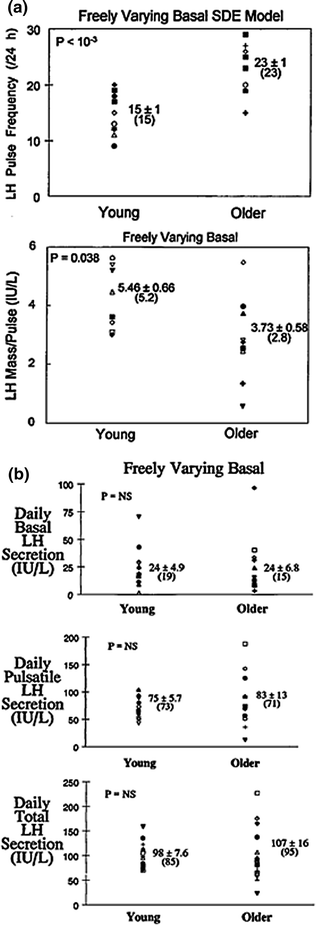
Fig. 16.5
Comparison of LH secretory attributes in young and older men based on a variable-waveform model that allows a freely varying basal secretion rate. a Impact of age on LH pulse frequency (events/day) and LH secretory burst mass (IU secreted per pulse per L of distribution volume). b Deconvolution estimates of basal (top), pulsatile (middle), and total (bottom) daily LH secretion in young and older men. Numerical values are the mean ± SEM (median). P values are estimated by the rank-sum test. P = NS denotes <0.05. Adapted from [45]
One potential mechanism might relate to inflammation. Infusion of IL-2, which is an important inflammatory mediator, seems to potentiate the proportionate feedback inhibition of testosterone on LH in older men [27]. If verified, these data point to a possible interaction between age and concomitant comorbidities that cause inflammation. These data support the hypothesis that both age and comorbidities are associated with the age-related decline in testosterone.
Regulation of the Ensemble Hypothalamo-Pituitary-Leydig Cell Axis
An emergent thesis in neuroendocrine research is that early disruption of (negative) feedback and/or (positive) feedforward activation may occur without any demonstrable change in overall hormone production [34, 36, 41, 46, 47]. The foregoing insight is relevant to understanding early disarray of the hypothalamo-pituitary-gonadal axis in aging. Therein, we adopt the basic precept that repeated, dose-dependent, time-delayed adjustments among regulatory sites govern systemic androgen availability in a homeostatic fashion [45, 76, 96–103]. To aid intuition, we have formalized core feedback connections among GnRH, LH, and testosterone mathematically [45, 95–97]. A simplified model structure predicts that jointly impaired feedforward by LH, and feedback by testosterone (in part through aromatization to estradiol), would yield a high-frequency, low-amplitude, and disorderly pattern of LH release. A recent adaptation allows, but does not enforce, pulse-by-pulse hysteresis wherein the dose-response relationship during the ascending and descending phase of the response pulse peak is allowed to differ (Fig. 16.6) [104, 105]. On the other hand, isolated failure of GnRH outflow would explain only inappropriately low LH concentrations and attendant hypoandrogenemia, but not an elevated LH pulse frequency or irregular release patterns [46, 47]. Exploration of this ensemble concept requires more focused assessment of physiological regulation, as highlighted next.
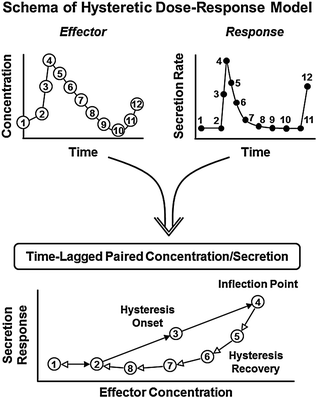
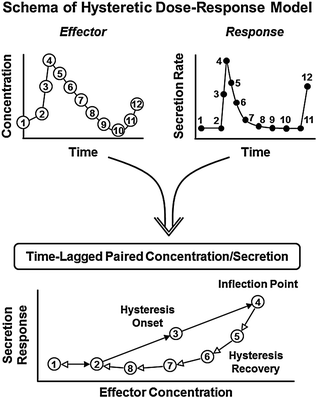
Fig. 16.6
Schema of dose-response hysteresis concept. Hormone (effector) concentrations within a pulse (top left) drive target-gland secretion rates (top right). The relationship between input (concentration) and output (secretion) is estimated as a dose-response logistic function (below). The onset (solid arrows) and recovery (open arrows) phases of the dose-response interface are estimated simultaneously with a hysteresis inflection point (delay time)
Sampling and Counting of Pulses
The frequency and amplitude of LH pulses provide a window to signals from the brain and pituitary gland. Withdrawal of blood at 5 or 10-min intervals for 12–24 h will capture the majority of discrete LH release episodes in healthy men [40, 106–110]. Suitable monitoring paradigms define an accelerated frequency and attenuated amplitude of pulsatile LH release in older men by independent methods of pulse detection, in separate gonadotropin assays and among different volunteer cohorts [34, 46, 47, 62, 111]. Figure 16.7 illustrates comparisons by age of immune-radiometric LH concentration times series collected every 10 min over 24 h [33]. Some, but not all, earlier analyzes using radioimmunoassay methods and/or less frequent (e.g., 20-min) blood-sampling protocols presaged the concept of lower amplitude and higher frequency LH peaks in older men [24, 40–42]. Recent biomathematical simulations establish that failure to detect either a loss of amplitude or a gain in frequency of LH pulses is a predictable technical artifact of insufficiently intensive blood sampling (Fig. 16.8).
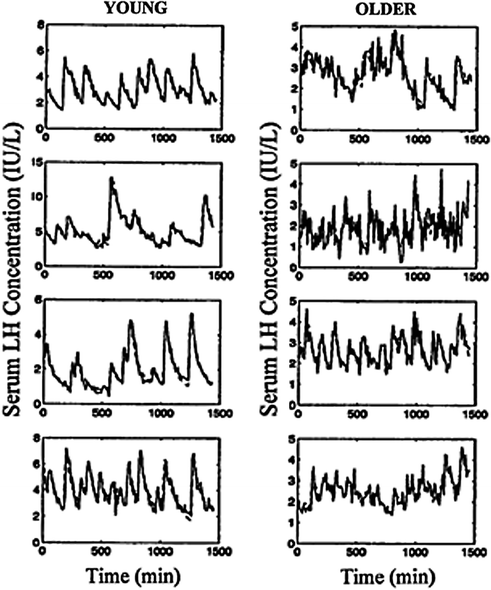
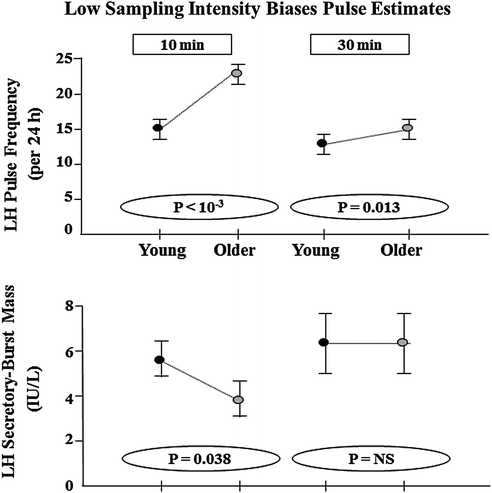
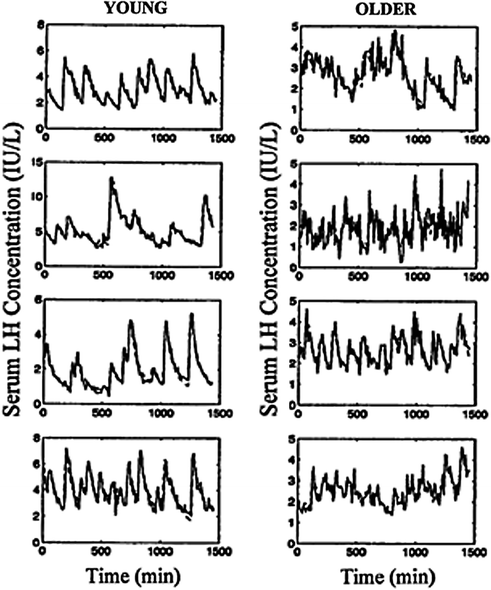
Fig. 16.7
Diminution in LH secretory burst mass and acceleration of LH pulse frequency in aging when compared with young men inferred by two deconvolution methods [47]. LH concentration profiles (± sample SD’s) measured in serum collected every 10 min for 24 h and analyzed by immuno-radiometric assays and variable-waveform (stochastic differential equation (SDE) based) deconvolution analysis (predicted continuous curve) [45]
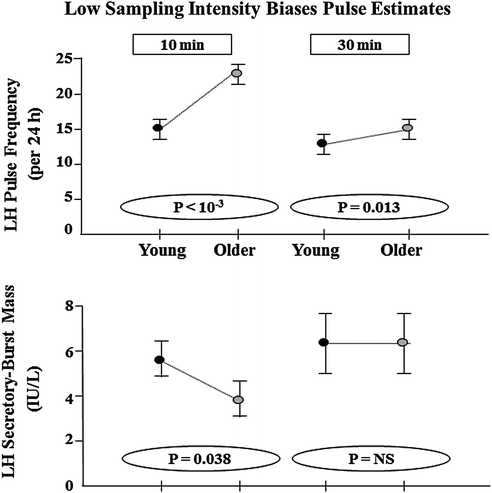
Fig. 16.8
Undersampling of LH release censors detection of LH pulses (top) and inflates estimates of LH secretory burst mass. The resultant technical bias limits the identification of age-related contrasts in pulse frequency and size. 24 LH concentration time series were edited from original 10-min sampling sets to yield 30-min daughter series (simple subsets) in 11 young and 13 older men. Unpublished reanalysis of data in [34, 35, 62] (Bradford, Kuipers and Veldhuis)
Gonadotrope Responsiveness to GnRH
Viewed mechanistically, a 50% reduction in incremental (peak minus nadir) LH pulse amplitude in aging men could denote a diminished amount of hypothalamic GnRH secreted per burst, an abnormal GnRH waveform and/or impaired gonadotrope-cell responsiveness to GnRH. Recent GnRH dose-response analyzes conducted in randomly assigned order on separate days establish normal maximal, and enhanced submaximal, acute and short-term (14-day) stimulatory effects of GnRH in the elderly male [34, 112, 113]. Heightened sensitivity to small amounts of GnRH occur predictably in the presence of augmented pituitary LH stores in older individuals, as inferred post mortem. Furthermore, pituitary sensitivity to a wide 300-fold range of intravenously administered pulses of GnRH is age-invariant when testosterone exposure is matched at a physiological concentration, or when a single submaximal GnRH dose is assessed across matching graded degrees of androgen repletion [86, 114].
Accelerated LH Pulse Frequency in the Aging Male
A basic, unresolved mechanistic issue is whether aging causes a primary hypothalamic disturbance that enforces a high frequency of GnRH pulses, and/or whether low bioavailable testosterone concentrations elevate LH pulse frequency via feedback withdrawal [33, 41–43, 47, 62]. This query is central to contemporary physiological assessments of aging, inasmuch as earlier investigations in healthy young men document that (i) infusion of testosterone or a nonaromatizable androgen suppress LH pulse frequency and elevate LH peak amplitude [30, 102, 115, 116]; and (ii) administration of ketoconazole (which depletes testosterone) or flutamide (which inhibits androgen-receptor binding) augments LH pulse frequency and reduces incremental LH peak (fractional) amplitude [93, 94, 117]. In the human, monkey, sheep and rat, a high frequency of GnRH stimuli reduces incremental (fractional) LH pulse amplitude [43, 55]. In view of this reciprocal control mechanism (albeit not fully understood), diminished androgen bioavailability in older men could contribute in principle to both more rapid and lower amplitude LH pulses. What remains unknown is whether hypoandrogenemia in aging fully accounts for the observed neuroendocrine changes.
The time pattern of LH secretion within the physiological range is now directly known to influence testosterone response [118, 119]. Nineteen healthy men aged 18–49 years received four infusions in random order: saline, and continuous, hourly and second hourly rh LH matched for total dose infused over the entire 12-h period. Hourly, rh LH exposure resulted in higher testicular secretory sensitivity, greater rh LH potency, greater pulsatile testosterone secretion and faster half-time of appearance of peak testosterone. These data show that the time pattern of LH secretion enforces quantifiable differences in testosterone secretory dynamics, and suggests but does not prove that the increased frequency of LH pulses with age is an adaptive response to maintain testosterone output.
Monitoring Unobserved Gonadotropin Secretion
Lower incremental LH peak amplitude in older men can be dissected further mechanistically under the premise that fluctuating hormone concentrations are driven by specific (unobserved) secretion and kinetic processes. Deconvolution analysis is a family of computer-assisted methods designed to quantitate underlying secretion and/or elimination. Some deconvolution procedures allow one to determine the following: (i) the frequency of secretory bursts; (ii) the amount of hormone released within each burst [mass of hormone discharged within the event normalized to unit distribution volume]; (iii) the subject- and condition-specific half-life of elimination of the hormone; and (iv) concomitant basal or (nonpulsatile) secretion.
There are several complementary deconvolution approaches to secretion analysis. Figure 16.9a illustrates one basic idea, wherein an unobserved Gaussian-shaped secretory burst creates each peak in serum hormone concentrations [120]. In this waveform–dependent concept, hormone concentrations increase rapidly due to the sharp onset of an underlying secretory burst, and then fall gradually after the burst, as the hormone is eliminated slowly from the circulation. The Gaussian-burst model allows one to calculate the number, location, duration, and amplitude of secretory bursts and predict the hormone half-life at the same time [121, 122]. Application of this methodology in healthy men quantitates a lower mass and higher frequency of LH secretory events in older than young men, but a comparable LH half-life [33, 41, 62]. The lower mass (IU) of LH released per secretory burst in the elderly male is due to attenuation of the amplitude (maximal secretion rate attained) rather than abbreviation of the duration (min) of release episodes.
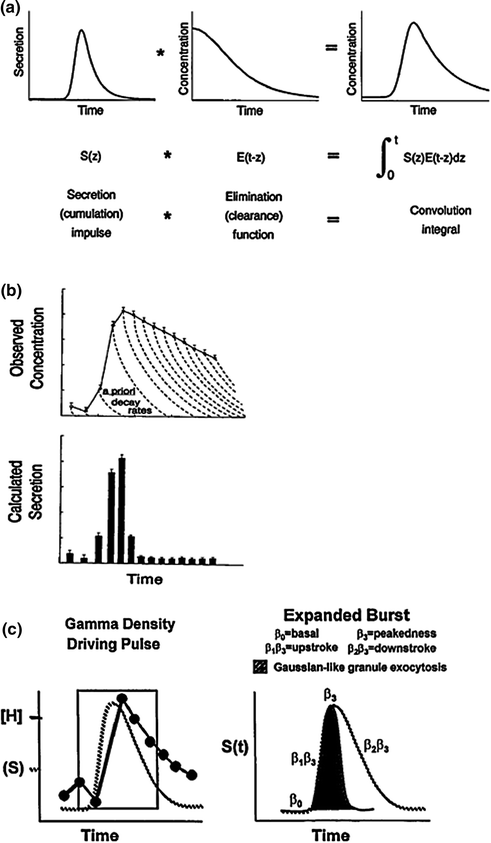
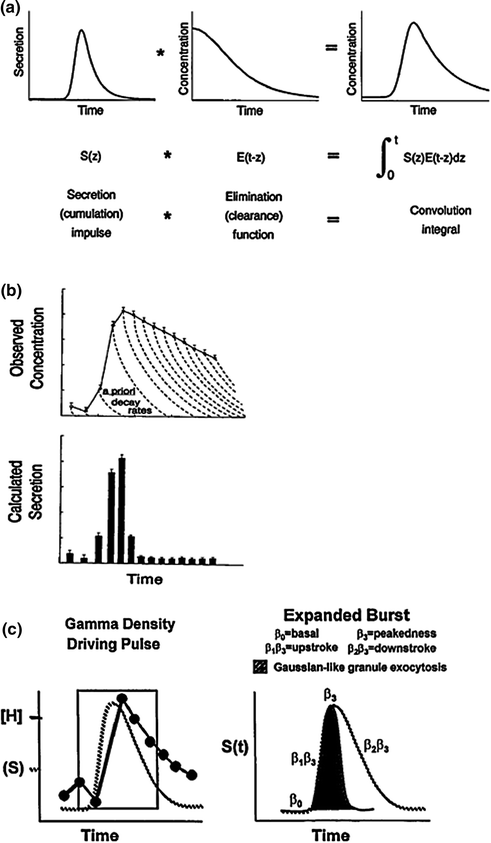
Fig. 16.9
Concept of deconvolution analysis as a family of methods designed to quantitate unobserved secretion and/or kinetics from fluctuating hormone concentrations. a A Gaussian or slightly skewed secretory burst model (top left) provides estimates of time-delimited, burst-like discharge of molecules with individual release velocities driving a pulse. A secretory burst is defined by its position in time, maximum (amplitude), and half-duration (duration at half-maximal height) [120, 122]. The time integral of the convolution product of the secretory burst (left) and disappearance function (middle) yields the pulsatile hormone concentration (right). The reverse process of deducing underlying secretion and elimination from observed concentrations is termed deconvolution analysis. b A waveform–independent approach allows one to calculate sample secretion rates (bottom) from measured hormone concentrations (top) based on a priori biexponential kinetics (interrupted decay curves). Experimental errors inherent in hormone measurements and the populational half-life estimate are combined to determine statistical confidence limits for each sample secretion rate [121, 123]. c Flexible deconvolution construct of a generalized Gamma–density (variable) secretory–pulse waveform is utilized to encapsulate burst timing, number, shape and size, basal secretion, and rapid and slow-phase hormone elimination kinetics simultaneously with random effects [95, 97]. Random effects arise from sampling uncertainty, assay error, stochastic admixture of secreted hormone in the bloodstream, unpredictable pulse times and varying effector-response interface properties [46, 96]
A second-generation deconvolution technology defines secretion as an admixture of unknown basal output and superimposed events of any height, width, shape, and number [121] (Fig. 16.9b). This deconvolution construct is therefore defined as waveform–independent. For statistical reasons, optimal analysis in this methodology requires a priori knowledge of the two-component half-life of hormone elimination [123]. Because the true waveform of secretory bursts is (definitionally) unknown, definitive pulse counting is analytically difficult unless assumptions are made in fact about event shape, smoothness, location, or number [122]. However, this mathematical strategy likewise predicts lower peak rates of LH secretion in older men.
A third-generation deconvolution strategy uses coupled, stochastic differential equations to reconstruct simultaneously the number, shape, size, and timing of (unknown) secretory bursts, the basal release rate, biexponential disappearance kinetics and random effects driving hormone release [96, 97] (Fig. 16.9c). This comprehensive statistical platform predicts a lower mass and higher frequency of LH secretory bursts in older than young men with no age difference in LH kinetics [45, 47, 95]. Estradiol but not age per se reduces LH bioactivity in vitro and prolongs LH metabolic clearance rate in vivo [73, 124]. The diminution in mass and acceleration in frequency of LH pulses tend to counterbalance closely, thus leaving the total and pulsatile daily LH secretion rate unchanged in aging individuals.
The fourth-generation deconvolution strategy allows for computer automation by comparing all possible pulse sets and objectively choosing the best model fit using mathematically validated criteria (e.g., Akaike Information Criterion) [105]. This method has been mathematically and empirically validated in three mammalian species [125, 126]. Future advances being investigated include allowing for pulse-by-pulse flexibility in pulse shape [105].
Contemporary secretion-based insights explain some earlier observations. For example, one method of discrete hormone peak detection, Cluster analysis, identifies a pulse simply as a statistical jump and drop in the hormone concentration [40, 127]. This approach is termed model-free, since few assumptions are made in defining a pulse. Deconvolution analysis illustrates that the peak increment is proportionate to the mass of hormone secreted in the burst ceterus paribus [122, 123, 128]. This point is important, since elderly men consistently exhibit a lower incremental LH peak amplitude compared with young counterparts [34, 95].
Regularity Analysis
Homeostasis within an endocrine axis requires repeated decremental and incremental adjustments in secretion [46, 96, 97] (Fig. 16.1). In the male gonadal axis, core homeostatic signals include (at least) GnRH, LH, and testosterone, which enforce adaptations over a rapid (minute-by-minute) time course [129]. Orderliness of the resultant subpatterns of hormone release can be quantitated objectively via a regularity statistic, approximate entropy (ApEn) [36, 130–132]. ApEn is calculated on a desktop computer as a single number, which exploits accurate probabilistic accounting to quantitate the reproducibility of successive measurements in a time series [133] (Fig. 16.10a). Higher ApEn denotes heightened irregularity of minute-by-minute hormone release, which in turn signifies deterioration or adaptation of feedback and feedforward adjustments [131, 134, 135].
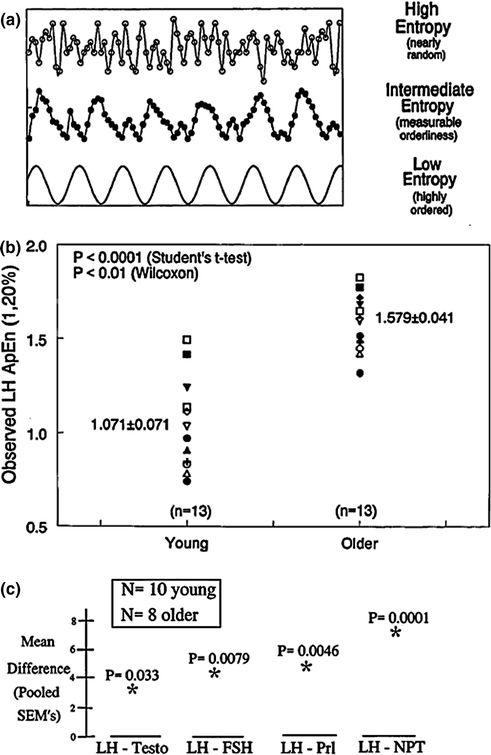
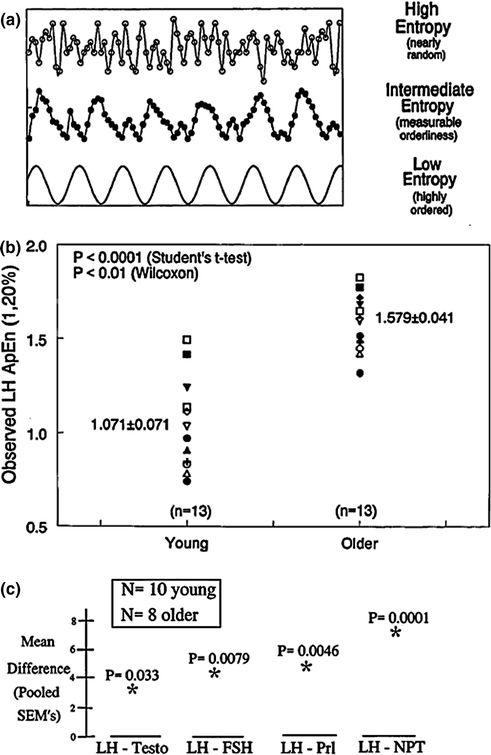
Fig. 16.10
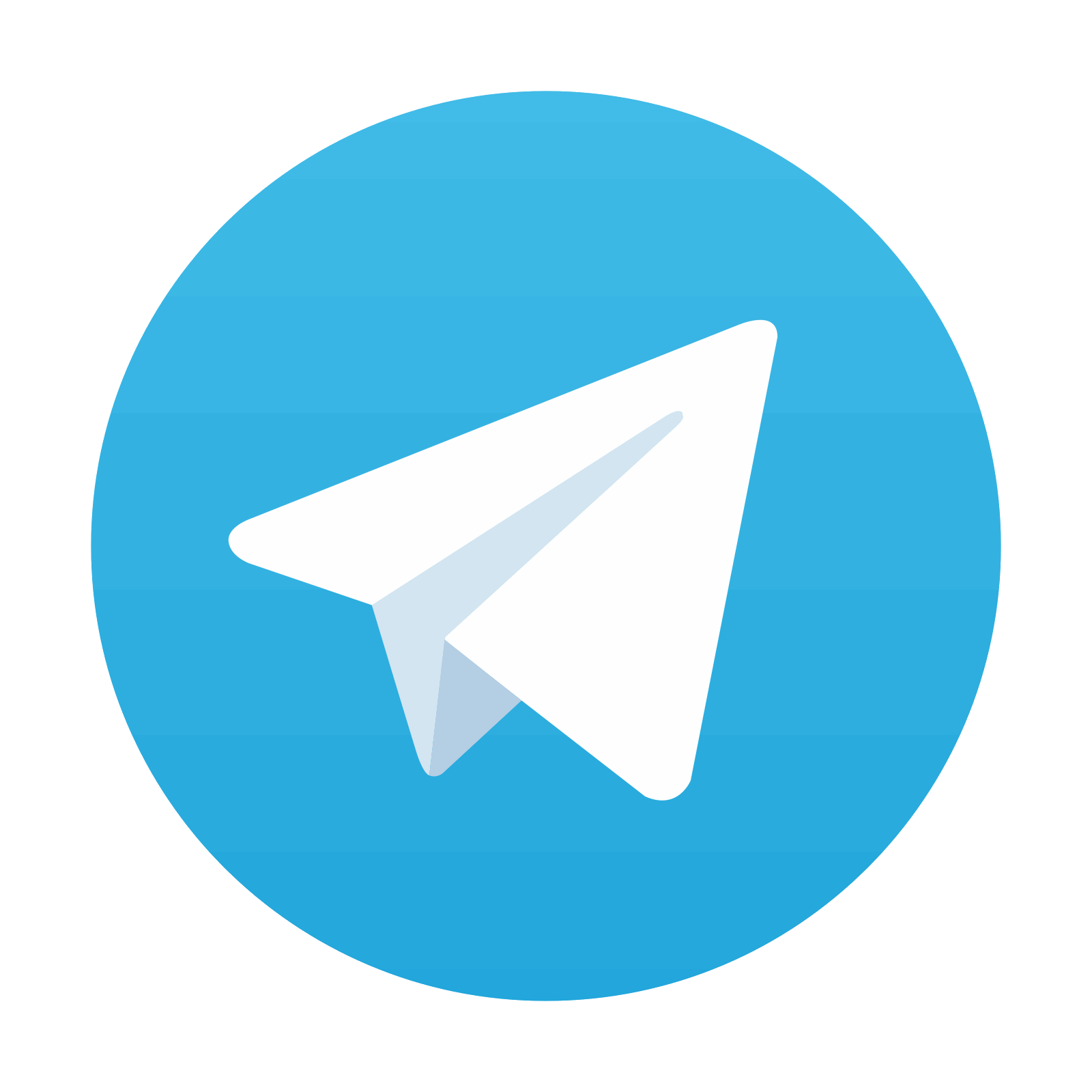
a Notion of the approximate entropy (ApEn) statistic to quantitate relative regularity of serial (sample-by-sample) measurements. The bottom curve gives a cosine function (low ApEn) to illustrate a well reproduced pattern; the middle curve shows partial degradation of regularity (intermediate ApEn); and, the top frame depicts marked erosion of orderliness (high ApEn). b Increased ApEn of overnight (2.5-min) LH release profiles in healthy older compared with young men. Higher ApEn points to less orderly outflow of GnRH and/or LH, or disruption of the GnRH-LH feedforward interface. Numerical values are the mean ± SEM. c Cross-approximate entropy (cross-ApEn) differences in older and young men. Differences exceeding three SEM’s (older minus young) denote significant deterioration of young-adult coupling between oscillations of LH and testosterone (leftmost), LH and FSH (left middle), LH and prolactin (PRL, right middle) and LH and nocturnal penile tumescence (NPT) (rightmost). P values denote the probability of falsely rejecting the null hypothesis of equivalent two-hormone synchrony in the two age groups. Unpublished compilation of data reported in [36–38, 111]
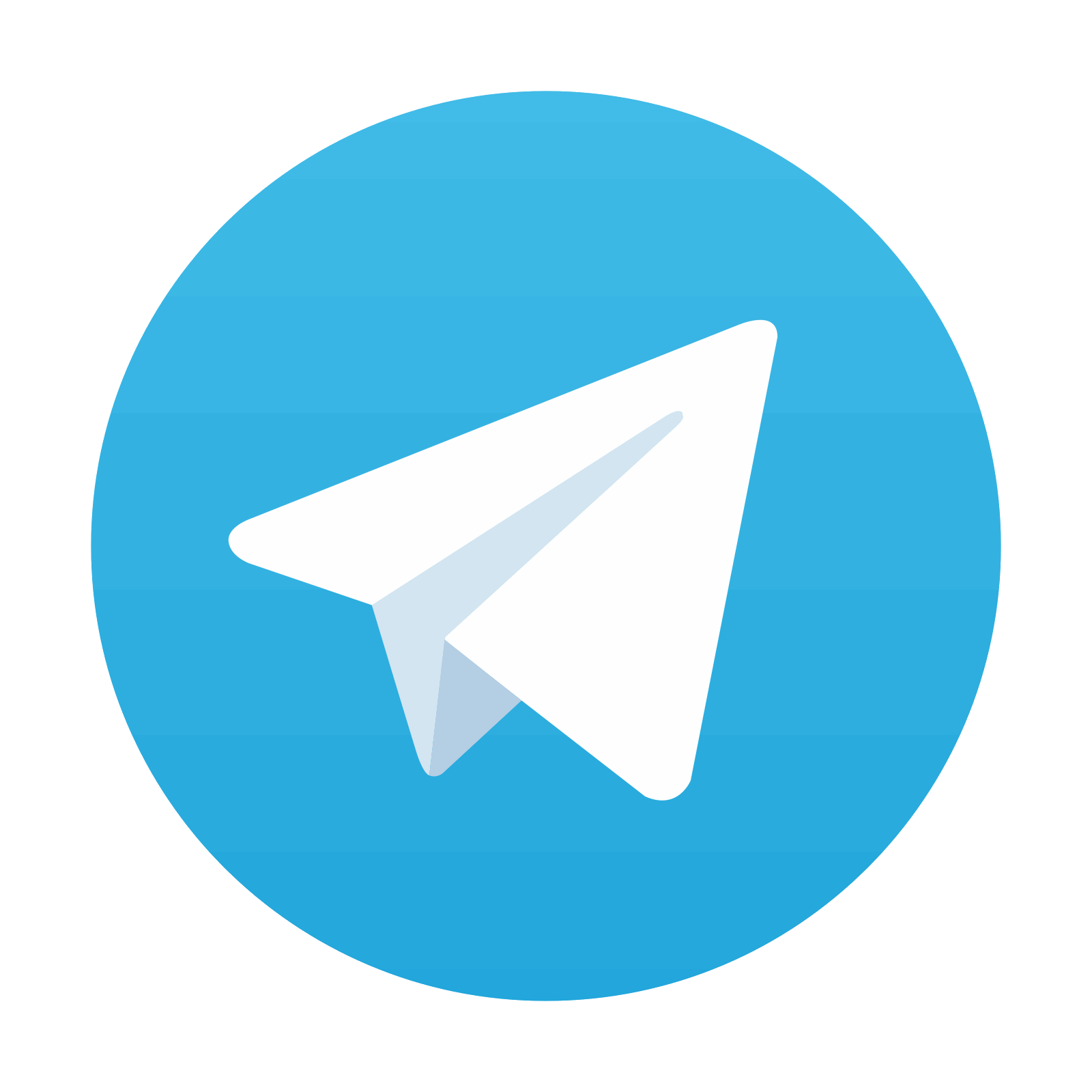
Stay updated, free articles. Join our Telegram channel

Full access? Get Clinical Tree
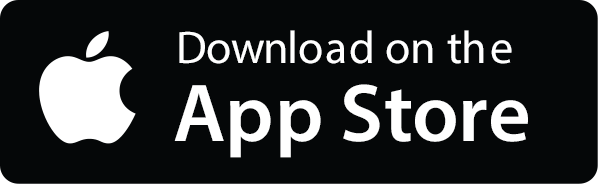
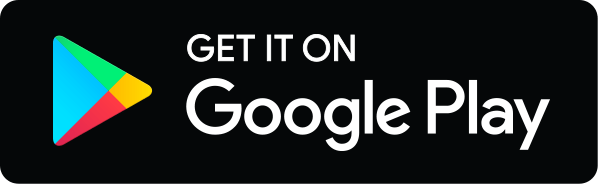
