Fig. 5.1
Schematic presentation of the activity of the hypothalamic–pituitary–gonadal axis across the life cycle. During the neonatal window, pulsatile GnRH stimulates LH and FSH secretion which induce adult levels of testosterone and inhibin B. Childhood is marked by a low-amplitude LH secretion and very low T levels. Pubertal reactivation of the HPG axis triggers the onset of sexual maturation, initially in a sleep-entrained pattern
GnRH neurons originate outside the central nervous system (CNS), primarily from the neuro-ectodermally derived embryonic olfactory placode within a smaller subset of these pioneer cells that have recently been shown in murine studies to be of neural crest origin [1]. These developing GnRH neurons then migrate along the olfactory sensory axonal tracts as they enter the forebrain through the cribriform plate and eventually reach their final destination in the medial preoptic area of the hypothalamus.
GnRH neurons have been observed to be in place within the human hypothalamus by the ninth week of gestation, and secreted gonadotropins can be detected in the fetal circulation by 12–14 weeks of gestation [2]. In the neonatal period, the hypothalamo–pituitary–gonadal (HPG) axis is fully active when its pulsatile pattern of GnRH secretion initiates an initial rise in gonadotropin secretion that in turn initiates the first wave of systemic secretion of hormones from the gonads of fetuses of both sexes [3]. In male infants, this initial wave of HPG axis activity, termed the mini-puberty of infancy, declines by 6 months [3].
This initial neonatal HPG activity in male infants serves two key physiological functions: rapid proliferation of the Sertoli cells [4] and increase in germ cell number [5], both of which ultimately determine future successful spermatogenesis. If in-utero/neonatal activation of the HPG axis is defective, male infants may present with micropenis and cryptorchidism as seen in patients with CHH (see below). Following this early HPG axis activity in both sexes, the secretory activity of the HPG axis is dampened during most of childhood. However, the HPG axis is not completely quiescent since the pulsatile gonadotropin release, albeit at extremely low-amplitude pulses, has been shown to occur in prepubertal boys using ultrasensitive LH assays [6].
The onset of puberty is subsequently marked by a gradual “reawakening” of the dormant GnRH pulse generator, initially in a sleep-entrained manner, with a striking increase in the amplitude of LH pulses and less change in pulse frequency, resulting in stimulation of the gonads [7, 8]. As puberty progresses, GnRH-induced gonadotropin pulses occur during both the day and night, eventually concatenating in the development of secondary sexual characteristics and the changes in body composition characteristic of puberty. In adult males, GnRH-induced gonadotropin secretion averages one pulse approximately every 2 h (Fig. 5.2), but there is considerable variability among men in both the frequency and amplitude of LH pulses as well as in the ensuing T levels. Indeed, 15% of normal men exhibit long interpulse intervals between LH secretory pulses, resulting in a transient decrease in serum testosterone (T) levels to as low as 3.5 nmol/L (Fig. 5.3) [9].
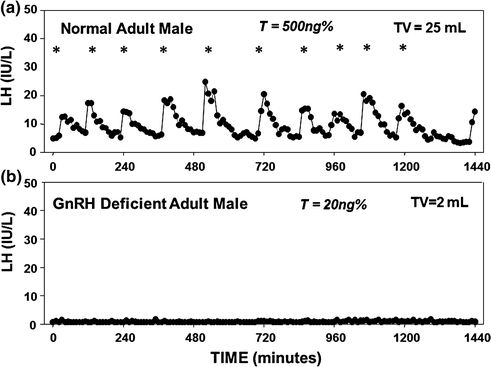
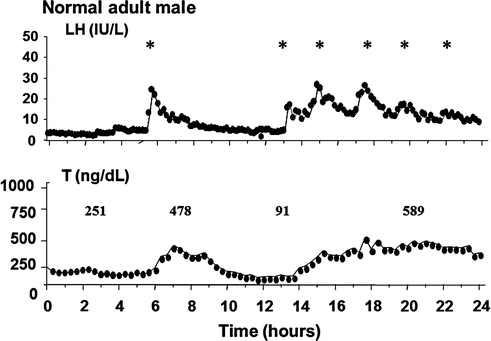
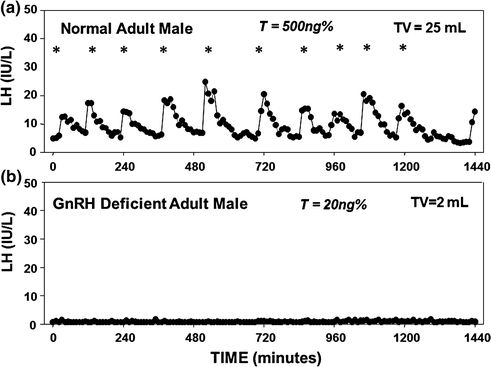
Fig. 5.2
Frequent blood sampling (every 10 min for 24 h) for LH in a normal adult male and a man with CHH. a Normal adult male pattern of GnRH secretion with high-amplitude LH pulsations (10 pulses in 24 h), normal serum T level, and normal testicular volume; b Apulsatile pattern of GnRH secretion in an CHH male as assessed by a complete absence of endogenous LH pulsations, low serum T levels, and prepubertal-sized testis
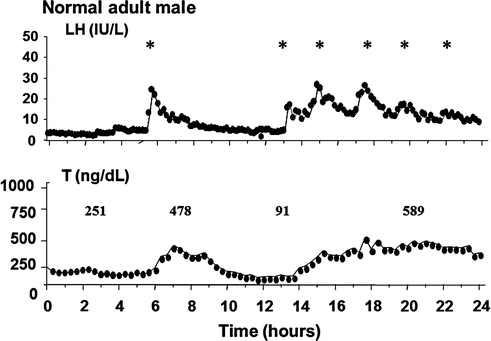
Fig. 5.3
Frequent blood sampling (q 10 min for 24 h) of serum T and LH in a normal adult man revealing a nadir T level of 91 ng/dl (to convert to nmol/L, multiply by 0.03467) after a long interpulse interval of LH secretion. Adapted from Spratt et al. [9]
Normal Gonadal Development in the Male
The gonadal sex, i.e., initial development of the fetal testes from undifferentiated gonad, is initiated by the expression of the SRY (sex-determining region Y) gene. Fetal testicular Leydig cells, stimulated by placental hCG, produce T that induces the growth, stabilization, and differentiation of the gonadal Wolffian structures (epididymis, vas deferens, and seminal vesicles). The 5α-reductase enzyme converts T to the more potent androgen, 5α-dihydrotestosterone, which induces the formation of the prostate and the external male genitalia. Secretion of anti-Müllerian hormone (AMH) from fetal testicular Sertoli cells causes regression of the Müllerian structures. During the later stages of gestation and in the “neonatal window of mini-puberty,” GnRH-induced LH secretion drives T and insulin-like factor 3 (INSL3) secretion by the Leydig cells (T levels reaching adult levels in the neonate), directs the completion of the inguino-scrotal phase of testicular descent, and induces further growth of the phallus [10]. This first wave of postnatal Sertoli cell proliferation in response to GnRH-induced FSH secretion results in increases in Sertoli cell number during infancy [11], and this developmental phase is critical for setting the stage for future spermatogenic success in adulthood. Prior to the onset of puberty, there is a gradual threefold increase in testis size as evidenced by postmortem studies in childhood trauma victims [12]. This increase is caused by a lengthening of the seminiferous tubules, due mainly to intense proliferation of Sertoli cells and, to a lesser degree, of immature germ cells [11, 12].
A second phase of Sertoli cell proliferation occurs early in puberty with further increase in number after which gonadal cellular proliferation ceases, Sertoli cells mature and the blood–testis barrier is established, the seminiferous tubular lumen forms, and spermatogenesis is initiated [13, 14]. Both the neonatal and pubertal Sertoli cell maturation phases are marked by discrete developmental windows of neuroendocrine activity [15]. Ultimately, this final complement of mature Sertoli cells is the major determinant of testes size, seminiferous tubular development, and sperm count, as each Sertoli cell can only support a finite number of germ cells [16–18]. Thereafter, a dramatic increase in meiotic germ cells promotes the most significant increase in testis size that occurs during puberty. Finally, the improvement of spermatogenic efficiency leads to complete maturation of the seminiferous tubules. While intratesticular T plays a central role in stimulating spermatogenesis both directly and in concert with FSH (see Chap. 3), the subsequent increase in systemic T levels induces the secondary sexual characteristics of the adult male. The specific role of FSH in adult males is not yet well established. However, based on the phenotype of the males with mutations in either the FSHβ or FSH receptor (see Chap. 6), it appears that FSH is necessary to maintain both qualitative and quantitative normal spermatogenesis.
Idiopathic or Congenital Hypogonadotropic Hypogonadism
HH (i.e., secondary hypogonadotropic hypogonadism) is differentiated from primary testicular disease (i.e., primary hypergonadotropic hypogonadism) by the demonstration of low/inappropriately normal gonadotropin levels in the setting of low sex steroid levels and impaired spermatogenesis. Congenital abnormalities that lead to HH are rare but are well described and result from either hypothalamic GnRH deficiency or pituitary disorders that affect the gonadotropes.
Differential Diagnosis of Congenital Hypogonadotropic Hypogonadism
Hypothalamic Causes
Idiopathic Hypogonadotropic Hypogonadism (IHH)
Anosmic form: Kallmann syndrome
Non-ansomic form: Normosmic IHH (nIHH)
Variant forms of IHH:
Fertile eunuch syndrome
Reversible form
Adult-onset HH
Complex syndromic forms of IHH
Adrenal hypoplasia congenita and IHH
Morbid obesity syndromes: Prader–Willi syndrome; leptin pathway mutations
CHARGE syndrome
TUBB3 E410 K syndrome
Waardenburg syndrome
Gordon-Holmes syndrome Boucher-Neuhauser syndrome
Bardet-Biedl syndrome
Pituitary Causes (see also Chap. 8):
HH associated with other pituitary hormone deficiencies
Genetic defects of the gonadotropin subunits and pituitary transcription factors
Congenital HH (CHH), also referred in the literature as idiopathic hypogonadotropic hypogonadism, results from genetic mutations that lead to abnormalities in GnRH development, secretion, and/or action. CHH presents in two major phenotypic forms: (i) Kallmann syndrome [KS], a syndromic form characterized by GnRH deficiency accompanied by an absence of olfaction (anosmia) with or without other non-reproductive features such as midline defects, skeletal abnormalities, and other somatic features; and (ii) normosmic CHH, characterized by GnRH deficiency with normal sense of smell [19, 20]. Until the discovery of GnRH, CHH was thought to be secondary to pituitary gonadotropin deficiency. However, when GnRH became available, increases in the serum levels of LH and FSH following administration of a single bolus of GnRH in these subjects suggested a hypothalamic defect [21–23] which was subsequently unequivocally confirmed when administration of exogenous GnRH to these patients in a pulsatile fashion completely restored a physiologic pattern and levels of gonadotropins and sex steroids [24, 25].
CHH can also be a component of complex, severe syndromes with multiple somatic abnormalities in which the reproductive phenotype is but a component of a larger spectrum of phenotypes (see Complex Syndromic Forms of CHH section below). GnRH deficiency may also result from functional causes (e.g., critical illness, excessive exercise, nutritional deprivation, and drugs—especially opiates) (see Functional Cause of HH section below). CHH resulting from impaired production of pituitary gonadotropins with or without other pituitary hormone deficiencies includes genetic mutations in genes encoding LHβ (LHB) or FSHβ (FSHB) subunits (see Chap. 6). In addition, mutations in pituitary transcription factors such as POU1F1, GLI2, FGF8, PROP1, LHX3, LHX4, HESX1, SOX3, OTX2, TBX19, TCF7L1 and SOX2 and structural lesions/tumors involving sellar/pituitary anatomy can also present with hypogonadotropism as part of their complex syndromes. Pituitary disorders causing hypogonadism are discussed in Chap. 8.
Idiopathic Hypogonadotropic Hypogonadism (CHH)
Definition and Incidence
CHH is characterized by an isolated defect in the secretion or function of GnRH. Typically, CHH is diagnosed in adolescence due to a failure of puberty, although rarely it may be possible to diagnose this condition in the male neonates (see below). Diagnostic criteria for CHH in males include (i) absence or failure of completion of puberty by age 18 years, (ii) decreased T levels in the hypogonadal range (e.g., <3.5 nmol/L; 100 ng/dL), (iii) low/normal gonadotropin levels representing absent or reduced GnRH-induced gonadotropin secretion, (iv) otherwise normal hormonal testing of the anterior pituitary, (v) a normal ferritin level to eliminate hemochromatosis, and (vi) normal radiographic imaging of the hypothalamic-pituitary region.
Two distinct clinical forms of CHH have been recognized historically. Franz Kallmann reported a familial form of this condition in 1944, in which CHH was seen in males who also had congenital anosmia, and this simple syndromic form has since been referred to as Kallmann syndrome (KS) [26]. However, it was subsequently recognized that some patients with CHH presented with a normal sense of smell, and these patients are termed as normosmic CHH (nCHH). The KS form of CHH accounts for nearly 60% of patients while the remaining 40% present with nCHH [27, 28]. Since CHH is a rare clinical condition, definitive data on its prevalence are limited. However, a recent population-based epidemiological study from Finland showed a minimal prevalence estimate of the KS to be 1:48,000 with a clear difference between males (1:30,000) and females (1:125,000) [29]. The prevalence of the normosmic form is likely to be similar with a similar M:F ratio, but epidemiological data will be required to confirm this assertion.
Clinical Features
While the dichotomous classification of CHH into KS and nCHH based upon the presence or absence of sense of smell is frequently used in the literature, a substantial clinical phenotypic overlap is seen between these two forms [30]. Moreover, different family members within a single sibship may manifest either KS, nCHH, or isolated anosmia without reproductive failure [31]. Therefore, stratifying patients according to reproductive phenotypes that relate to the timing of onset of HH and the severity of HH provides greater insight into the clinical spectrum of CHH [30]. Similarly, the presence of specific non-reproductive phenotypes often provides developmental insights into the various organ systems affected by specific genetic forms of CHH [32].
Reproductive Features of CHH Relating Timing of Onset of GnRH Deficiency and Severity of GnRH Deficiency
Neonatal Period
As mentioned above, the diagnosis of CHH is typically delayed until adolescence. However, severe GnRH deficiency disrupting the normal neonatal period of HPG activity (i.e., mini-puberty) may provide early diagnostic clues for the diagnosis of CHH in newborn male infants. The presence of cryptorchidism and/or micropenis (also referred to as microphallus) in a male infant may indicate CHH, although GnRH deficiency may still be present even if these two phenotypic features are absent. Typically, gonadotropin, sex steroid, and inhibin B levels are inappropriately low in such infants reflecting their inability to normally activate the HPG axis during the neonatal window [33]. Notably, these neonates have otherwise normal sexual differentiation without any urogenital sinus malformations such as hypospadias since early fetal testicular T production from fetal Leydig cells (see Chap. 2) and testicular fetal AMH production are normal [34]. However, cryptorchidism and micropenis in CHH patients indicate that during the late fetal/early neonatal period, endogenous secretion of GnRH is necessary for inguino-scrotal descent of the testes and full growth of the external genitalia [10].
Adolescence
In the absence of the hallmark neonatal reproductive features of micropenis and/or cryptorchidism, a definitive clinical diagnosis of CHH can only be made when isolated gonadotropin deficiency is manifested at the time of adolescence when boys present with delayed puberty. The clinical hallmark of CHH is the failure of onset of puberty. However, it is important to recall that CHH is rare, and that most adolescents with pubertal delay will represent those with constitutional delay of growth and puberty [CDGP] in whom spontaneous endogenous GnRH activity eventually ensues and puberty is subsequently initiated and completed (see delayed puberty section below and Chap. 4). While distinct from CHH, CDGP is frequently reported among the family members of CHH patients [31]. In our series of 106 patients with CHH, 12% had relatives with a history of delayed puberty [3] versus 1% in the general population [35]. These observations suggest that delayed puberty may represent the mildest end of the phenotypic spectrum of CHH. In keeping with this notion, recent studies have also reported a shared genetic basis between the two conditions [36].
Considerable clinical heterogeneity is observed in CHH patients at the time of presentation. The vast majority (~75%) of CHH patients are severely testosterone deficient and fail to develop any signs of puberty (Fig. 5.4). Their clinical features include no signs of virilization, (lack of any secondary sex characteristics), absence of an adolescent growth spurt but the presence of normal childhood growth but with delayed epiphyseal fusion resulting in eunuchoidal body proportions (upper/lower body ratio <1; with an arm span exceeding standing height by >6cm) [37], a high-pitched voice, slight anemia, delayed bone age, and prepubertal-sized testes (<4 mL). A large proportion of these severe GnRH-deficient subjects also report a history of cryptorchidism (40%) or microphallus (20%), providing evidence for the lack of activity of the HPG axis during the late fetal/neonatal window [38]. It is, however, important to recognize that the prevalence of microphallus among these severely affected CHH men with no prior puberty might be underestimated given the reported successful induction of phallus growth with androgen therapy during childhood [39].
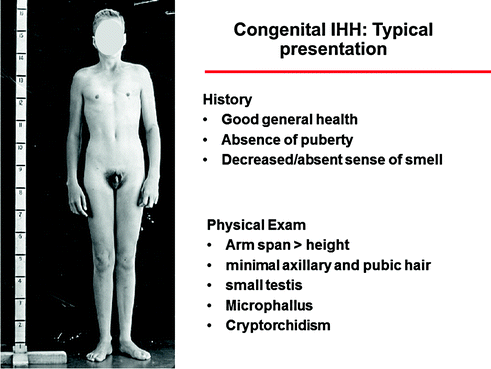
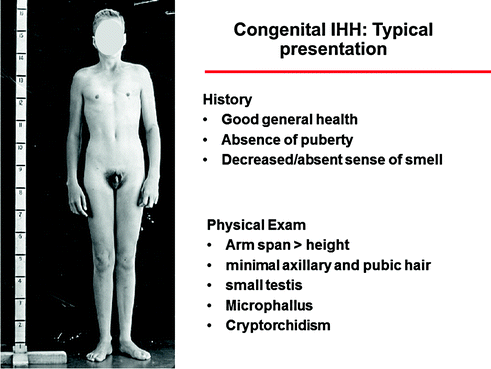
Fig. 5.4
Typical presentation of a CHH man with the most severe phenotype, including absence of puberty, eunuchoidal proportions, prepubertal testis, microphallus, and cryptorchidism
The remainder of CHH subjects (~25%) present with signs of partial puberty, displaying some degree of spontaneous puberty as assessed by at least two of these criteria: (i) some testicular growth, (ii) a growth spurt, (iii) an increase in the number of erections, and (iv) the initiation of shaving. However, these subjects, in contrast to boys with CDGP, fail to complete sexual maturation and remain hypogonadal. Low libido, lack of erectile function, low energy, and poor muscle bulk are the common somatic symptoms reported by CHH men. Gynecomastia is seen in 20–30% of CHH men and is often related to prior therapy with gonadotropins [30]. The key clinical features are depicted in Fig. 5.4.
Non-reproductive Features of CHH
Many CHH subjects, especially those with KS, may also exhibit a spectrum of non-reproductive features which may indicate a more complex syndromic genetic etiology (see below). One of the common non-reproductive phenotypes commonly encountered in KS subjects is bimanual synkinesia (also called “mirror movements”) [28, 40]. Bimanual synkinesia is detected when voluntary movements of one hand are associated with similar involuntary movements of the opposite hand. Abnormal fast-conducting ipsilateral corticospinal tract projections are reported in these patients and are hypothesized to underlie the observed mirror movements [41, 42]. Defects in spatial perception have also been found in KS patients with synkinesia [43]. KS patients may also display unilateral renal agenesis [28, 44] and midline craniofacial and palate abnormalities [45]. Other common non-reproductive features include short metacarpals (short fingers, especially the fourth finger) [46], split hand/foot malformation [46], dental agenesis [47], congenital sensorineural hearing loss [32, 48], skin pigmentation anomalies [48], eye movement abnormalities [49], and rarely, cardiovascular defects [50, 51].
Variant Forms of CHH
Fertile Eunuch Syndrome
The first report of a man with the fertile eunuch variant of CHH was in 1950 by McCullagh [52]. These subjects display eunuchoidal proportions and are undervirilized but have normal gonadal size and preserved spermatogenesis. In this disorder, endogenous GnRH secretion, although decreased and insufficient to stimulate full virilization, appears adequate to produce sufficient intra-gonadal T levels to support spermatogenesis and testicular growth. Indeed, these patients can be fertile either spontaneously or with T or hCG therapy without the addition of FSH [53, 54]. The clinical picture of the fertile eunuch thus resembles that of normal mid-pubertal boys; indeed, a nocturnal rise of LH and T secretion synchronous with sleep, as seen normally in mid-puberty, was demonstrated in two men with the fertile eunuch syndrome [54]. These cases may have resulted from defects in GnRH regulation. In contrast, a patient with the fertile eunuch variant has also been described who had detectable, but apulsatile LH secretion associated with a partially inactivating mutation of the GnRH receptor (GnRH-R) [55]. These data underscore the clinical, genetic, and pathophysiological heterogeneity that exists in this syndrome.
Adult-Onset Hypogonadotropic Hypogonadism
A more delayed adult-onset presentation of nCHH, termed adult-onset hypogonadotropic hypogonadism, has been recognized in men with otherwise completely normal sexual development at puberty but in whom secretion of GnRH subsequently ceased during adulthood [56]. The robust responsiveness of these adult-onset subjects to exogenous GnRH administration demonstrates the hypothalamic nature of their defect. With long-term follow-up, these men fail to recover suggesting that they have a permanent defect in GnRH secretion [57].
Reversal of CHH
Although congenital CHH has been thought to be a lifelong condition, spontaneous recovery of endogenous GnRH secretion, as heralded by spontaneous testicular growth and normalization of sex steroid levels off therapy, has been demonstrated to occur in 10–15% of CHH men [58]. This reversal phenotype was seen following the therapy with a variety of treatments that effectively normalize sex steroid levels, suggesting that this prior exposure to sex steroid hormones is a key element to the milieu in which these “reversals” occur. The precise mechanisms behind this reversal process remain unknown. This phenomenon of “reversal of CHH,” even in those with severe GnRH deficiency such as KS subjects with absent olfactory structures, strongly suggests a hitherto underappreciated plasticity of the GnRH neuronal network that can override developmental and neuroendocrine genetic defects that resulted in the initial hypogonadotropic state at the time of puberty.
In one recent single-center study, nearly 45% of reversal subjects had demonstrable CHH- related gene mutations but a significant enrichment was seen for mutations that disrupt neurokinin B signaling suggesting that the neurokinin B pathway, although critical for puberty, may be dispensable later in life [59]. Based on other reports from the literature, mutation in the GnRH receptor (GNRHR) also seems to be overrepresented in reversal subjects. More recent longitudinal review of these reversal subjects has also revealed a fragility of this reversal phenomenon as some patients slip back into a hypogonadotropic state requiring the resumption of therapy. Hence, it is important for clinicians to periodically reassess CHH subjects for evidence of reversal but also to assess the persistence of this reversal state and reintroduce therapy if required.
Pathophysiology of Congenital CHH
Biochemical Studies
Despite variation in the clinical phenotype of CHH men, their biochemical findings are very similar. By definition, all patients have T levels in the hypogonadal range. Gonadotropins are low/normal in all subjects, which in the setting of low T levels indicates hypogonadotropic hypogonadism (Fig. 5.5). Interestingly, mean LH and FSH levels are significantly higher in those patients with some degree of spontaneous puberty (as reflected by larger testicular sizes, for example) as compared with those men with no prior puberty [30].
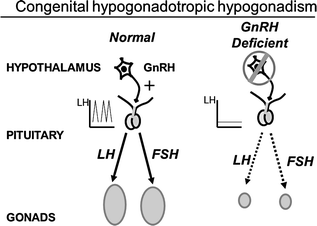
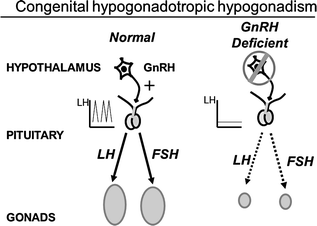
Fig. 5.5
Schematic of the hypothalamic–pituitary–gonadal axis in a normal adult male and in congenital hypogonadotropic hypogonadism
Serum levels of the Sertoli cell products, inhibin B and AMH, provide additional gonadal markers of the onset and extent of GnRH deficiency. In the normal ontogeny of inhibin B secretion, levels rise during the neonatal activation of the HPG axis [60] and then decline, but remain readily measurable throughout childhood despite low levels of FSH. During the early stages of puberty, inhibin B levels increase, plateau at stage II of puberty, and remain constant unless spermatogenesis is disrupted [61]. In contrast to normal men, CHH men with absent pubertal development display low/undetectable inhibin B levels [62] which are well below those of normal children [61]. Therefore, prior gonadotropin exposure appears to be required for normal inhibin B production during childhood. Thus, inhibin B levels represent a marker of reproductive axis activity during the fetal/neonatal period among patients with no sexual maturation. In CHH men with partial puberty, baseline inhibin B levels reach the normal range despite low gonadotropins, presumably reflecting adequate Sertoli cell proliferation during the neonatal window and early puberty. AMH, the first detectable secretory product of fetal Sertoli cells [63], is another biochemical marker of pubertal onset [64, 65]. Indeed, AMH levels are high throughout fetal and postnatal life and decline thereafter with the onset of spermatogenesis and the activation of LH-Leydig cell function [65]. Accordingly, AMH levels are high among CHH men with no pubertal development and are lower in those with partial GnRH deficiency [30]. Leydig cells also secrete INSL3 during the fetal and immediate postnatal periods, and INSL3 secretion ceases in childhood until puberty, peaking during adulthood [66]. Similar to inhibin B, baseline INSL3 levels are low in male CHH subjects and increase under gonadotropin stimulation [67]. It remains to be seen whether INSL3 levels and/or other physiological stimulation tests (e.g., kisspeptin stimulation) are able to distinguish adolescent subjects with CDGP from those who may progress to CHH.
Patterns of GnRH Secretion in CHH Subjects
Due to low circulating levels and a short half-life (2–4 min), GnRH in the peripheral circulation cannot be measured, and levels do not accurately reflect GnRH secretion [68]. Consequently, the study of GnRH secretion is limited to inferential approaches. Traditionally, LH is the most commonly used surrogate marker of the GnRH pulse generator [69, 70]. A spectrum of abnormalities in the neuroendocrine pattern of GnRH secretion is observed among CHH men. In the MGH’s retrospective study of 78 CHH men, using 10-min blood sampling for LH, 75% of CHH patients failed to exhibit any detectable LH pulses, implying a lack of GnRH pulse generator activity (Figs. 5.2 and 5.6) [30]. The remaining 25% demonstrated abnormal but detectable LH pulses. Among the latter group, the majority of patients displayed a normal LH pulse frequency with a low LH pulse amplitude (Fig. 5.6). Although a higher prevalence (80%) of apulsatile LH secretion is found among those who lack sexual development, detectable LH pulses were identified in up to 20% of subjects in this group [71]. It may be that the initiation of puberty requires higher levels of GnRH stimulation than that are required to maintain a neuroendocrine axis after puberty [72]. Also, 50% of CHH patients with some pubertal development do not exhibit any LH pulses [30]. This finding suggests that transient pubertal activation of the HPG axis may have occurred that was followed by a failure of the GnRH secretory program in these patients. Alternatively, this may reflect a failure to detect a suppressed pulsatile pattern of GnRH because the LH assay was not sufficiently sensitive. From these data, it appears that neither mean gonadotropin levels nor the current pattern of LH secretion is a reliable surrogate marker for complete versus partial CHH.
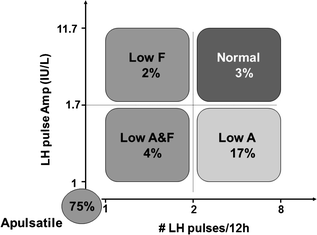
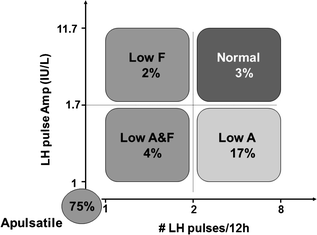
Fig. 5.6
Spectrum of GnRH-induced LH secretion abnormalities in men with CHH based on our retrospective study on 78 CHH men. 75% of CHH men exhibit no detectable LH pulses. 17% had a normal LH frequency but a low LH amplitude. 3% have a low LH amplitude and low frequency, 2% have a normal LH amplitude but low frequency. Finally, 2% have normal LH amplitude and frequency, deemed pathologic in the setting of hypogonadal T levels
The responsiveness of LH and FSH to 7 days of GnRH stimulation has been studied in CHH men and has been shown to correlate with the extent of seminiferous tubular development [73]. CHH men with no evidence of prior spontaneous pubertal development (TV ≤3 ml) showed sharp increases in serum FSH compared to men with some prior evidence of partial puberty (TV >3 ml), and inhibin B levels remained much lower in the former group. Conversely, the same group exhibited a decreased LH response to GnRH compared to the latter group who displayed robust LH responses to GnRH throughout the 7-day study. These observations underscore the role of negative feedback by inhibin B with increasing seminiferous tubule maturity on FSH responsiveness to GnRH while the LH response to GnRH is governed primarily by the extent of GnRH exposure.
Genetics of Congenital KS and NCHH
Patterns of Inheritance
Mirroring the phenotypic heterogeneity detailed above, the underlying genetic architecture of CHH is equally heterogeneous with mutations in several developmental genes causing KS, and mutations in neuroendocrine genes causing nCHH. Interestingly, some genes participate in the etiology of both KS and nCHH, and some subjects with KS have family members with nCHH, suggesting that the stratification between KS and nCHH might be oversimplified, and in fact may share common genetic mechanisms. The genetic complexity of CHH is also reflected in its different inheritance patterns. Given the reproductive failure associated with CHH, nearly two-thirds of CHH patients initially present as isolated or sporadic cases [27, 74]. Among familial cases, CHH can be inherited as an X-linked, autosomal dominant, or autosomal recessive trait [27]. Interestingly, in our cohort, the familial cases display a more severe phenotype with 95% of affected subjects presenting with absent pubertal development and a higher prevalence of cryptorchidism (71%) and microphallus (55%) [30]. While the excess of sporadic cases may suggest high rates of spontaneous de novo mutations causing the disease, closer review of their pedigrees, with detailed reproductive and non-reproductive phenotyping, often reveals subtle phenotypes that suggest familial inheritance patterns with variable penetrance [27]. In addition, as more subjects are successfully treated to enhance fertility, the incidence of familial cases may increase. In addition to these Mendelian modes of inheritance, a complex genetic architecture for CHH (occurring in 10–15% of cases) has now been documented wherein mutations in two or more CHH genes can be identified in CHH patients [75]. Almost all of the known CHH genes partake in the oligogenic inheritance mode. These oligogenic mutations presumably act in a synergistic manner, potentially accounting for some of the variable expressivity and incomplete penetrance that is characteristic of CHH.
Over the last three decades, several research groups have used a combination of clinical investigational strategies and contemporary genetic approaches to unravel the growing genetic complexity of CHH and to expand our understanding of the neurobiology of reproduction. To date, nearly 25 causal/contributory genes for the non-syndromic forms of CHH have been identified for this condition with varied modes of inheritance that affect either the development and/or functional integrity of the GnRH neurons (Fig. 5.7).
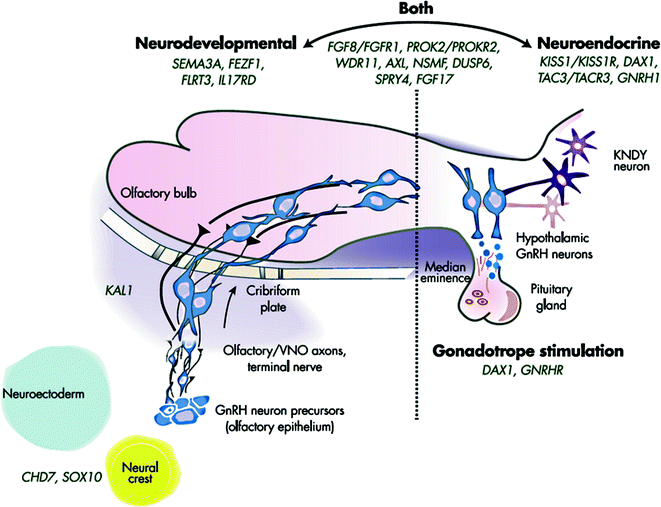
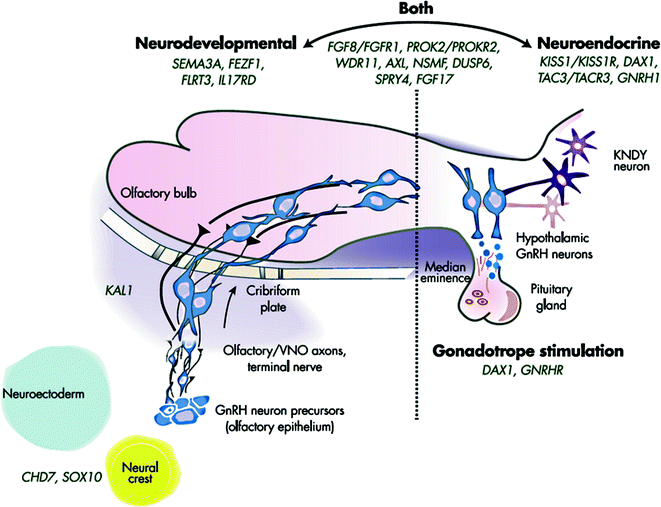
Fig. 5.7
Genetic causes of CHH showing neurodevelopmental and neuroendocrine regulation of GnRH neurons. Genes to the left are critical to GnRH neuronal migration, whereas those on the right are involved in proper GnRH function. A number of IGD genes (in the center) are involved in both processes. Adapted from Stamou et al. [213]
Genes Exclusively Causing KS Form of CHH
- (i)
X-linked recessive inheritance
ANOS1 (previously called KAL1 ), encoding anosmin-1 protein
Since the description of affected men by Kallmann et al. [27] in 1944, both X-linked (X-KS) and autosomal KS pedigrees have been recognized. Though the majority of familial cases of KS are inherited autosomally [76], initial attention was focused on several X-KS pedigrees with a complex syndromic presentation that included KS accompanied by ichthyosis, chondroplasia punctata, and choanal atresia. Karyotype analysis revealed a deletion in the tip of the X-chromosome at Xp22.3, suggestive of a contiguous gene deletion syndrome [77]. Eventually, the first KS gene, KAL1 (now called ANOS1), encoding an extracellular matrix protein, anosmin-1, was identified as the cause of KS using positional cloning of Xp22.3 and reported simultaneously by two independent groups [78, 79]. Subsequently, several groups have described many ANOS1 point mutations and deletions in non-syndromic KS patients [80].
Anosmin-1 is a secreted multi-domain protein consisting of an N-terminal signal peptide followed by a cysteine-rich region (cys-box), a whey acidic protein (WAP)-like four disulfide core motif, four tandem fibronectin type III (FnIII) repeats, and a C-terminal histidine-rich region. ANOS1 mutations occur across the length of the gene, and most mutations are loss of function (LOF) in nature (alteration of essential splicing, frameshift, or stop codons) resulting in the lack of synthesis of biologically active anosmin-1 protein. Missense ANOS1 mutations are rare [80]. The pathophysiology of disruption of anosmin-1 function in humans was studied in a 19-week gestation KS fetus known to harbor a deletion from Xp22.31 to Xpter, i.e., including the entire ANOS1 gene [81]. Histologic studies revealed arrested migration of GnRH neurons and olfactory neurons at the cribriform plate [81]. This defect of GnRH neuronal migration results in the failure of normal GnRH neuronal axonal communication with the median eminence that is imperative for the activation of the HPG axis. In phenotypic studies of men with ANOS1 mutations both in our cohort and in others, a severe pubertal phenotype was evident with complete absence of pubertal development, a high frequency of cryptorchidism and microphallus, an apulsatile pattern of LH secretion, low inhibin B levels, and histologically immature testes [30]. Despite the critical developmental role played by anosmin-1 in GnRH neuronal migration, mutations in ANOS1 account for only 10–14% of familial KS [76] and 8–11% of sporadic KS patients [28]. Human subjects with ANOS1 mutations, in addition to KS, display several specific non-reproductive features including, unilateral renal agenesis and synkinesia or mirror movements [28, 82]. In keeping with these clinical signatures, anosmin-1 is expressed in non-reproductive tissues such as mesonephric tubules, ureteric bud, digestive tract, large blood vessels, and inner ear [83]. Although several mutations of the ANOS1 gene cluster in the four fibronectin type III repeat domains [84, 85], no phenotype–genotype correlation has been demonstrated between the non-reproductive phenotypes and location of the mutation.
- (ii)
Autosomal dominant KS, with variable penetrance in some pedigrees
- a.
SOX10 , encoding a transcription factor, SRY (sex-determining region Y) box 10
The candidacy of SOX10 as a genetic cause of KS came from radiological observations in humans with SOX10 mutation-related Waardenburg syndrome (WS), a heterogeneous human “neurocristopathy” (i.e., disorders of cells from the neural crest origin) characterized by variable sensorineural deafness, abnormal skin and iris pigmentations, and Hirschsprung’s disease [86]. Imaging of temporal bone abnormalities of WS patients revealed an unexpected frequency of olfactory bulb agenesis, a finding reminiscent of KS [86]. In addition, one male with WS-related olfactory bulb agenesis also exhibited KS (anosmia and CHH). In 2013, Pingault et al. [87] studied 17 KS subjects of both sexes with at least one WS-related feature and 86 random KS cases, and identified eight subjects with a spectrum of SOX10 mutations (1 initiator codon, 1 frameshift, 1 splice site, and 6 missense mutations), all subsequently shown to be loss-of-function SOX10 mutations. In addition, study of Sox10 −/− mutant mice revealed a developmental defect in olfactory ensheathing cells, the specialized glial cell population normally found along the olfactory nerve pathway [87, 88]. In their absence, these mice misrouted their olfactory fibers, with impaired migration of GnRH cells and disorganization of the olfactory nerve layer of the olfactory bulb [55]. A third of the subcohort of KS with hearing loss was enriched for SOX10 mutations similar to the WS patients who also exhibit hearing loss [56]. This phenotypic overlap between KS and WS is also strongly suggestive of the presumptive role for neural crest cells in GnRH neuronal ontogeny. These observations are in keeping with murine [89] and zebra fish [90] data previously suggesting a critical role for neural crest cells in GnRH neurogenesis. Thus, this important gene provides compelling evidence for a subset of KS to be considered a human neurocristopathy disorder.
- b.
SEMA3A, encoding semaphorin 3A protein
Semaphorin 3A is a secreted guidance cue of the class 3 semaphorin family that had been initially shown to be important for the development of the GnRH neuron system in mice [91]. Loss of SEMA3A signaling alters the targeting of vomeronasal nerves and the migration of GnRH neurons into the brain, and hence recapitulates the human KS phenotype [91]. Subsequently, SEMA3A human mutations have provided validating evidence linking SEMA3A signaling and GnRH ontogeny in humans [92, 93]. In a single pedigree, heterozygous deletion of 11–17 SEMA3A exons was first identified to cause an autosomal dominant form of KS [92]. Independently, heterozygous SEMA3A mutations (1 frameshift mutation and 7 different missense mutations [R66W, N153S, I400V, V435I, T688A, R730Q, and R733H]) were found in a total of 24/386 (6.2%) KS subjects [93]. These mutations either showed impaired secretion of SEMA3A protein or reduced signaling of the secreted protein in GN11 cell lines (a neurodevelopmental GnRH cell line) [93]. Taken together, these observations suggest that mutations in SEMA3A cause KS via a loss-of-function (LOF) mechanism. In addition, in 5/24 patients with SEMA3A missense mutations, additional CHH gene mutations were identified, again confirming an oligogenic pattern of inheritance in CHH [93]. While several non-reproductive features were present, no significant enrichment for any specific non-reproductive feature was apparent in this group of patients.
- c.
IL17RD , encoding interleukin 17 receptor D protein
Once FGF8/FGFR1 mutations were documented in both KS and nCHH forms of CHH patients (see below), a bioinformatic interactome search-based association study (IBAS) to identify other gene mutations in a “FGF8 synexpression group” revealed 2 genes, IL7RD, and FGF17 as top candidate CHH genes [94]. In 8 KS subjects, seven IL17RD missense mutations (2 subjects with homozygous mutations and 6 subjects with heterozygous mutations) were found [94]. The functional effect of the IL7RD mutations was tested in a cell-based reporter assay in which cells co-expressing the mutants either displayed loss-of-function activity or decreased cell surface expression. IL17RD is a single transmembrane glycoprotein that is one of the major antagonists of FGF downstream signaling in vivo and in vitro [95–97]. High IL17RD expression occurs in the olfactory epithelium at E10.5, i.e., a time frame coinciding with GnRH neuronal fate specification thus suggesting a potential role for this gene in the early development of both GnRH and olfactory neurons [94]. Intriguingly, the IL17RD mutations identified were shown to cause loss of function. Given that IL17RD is an inhibitor of the FGF8 signaling system, these mutations should putatively increase FGF8 signaling in vitro. While this observation might appear counterintuitive given that FGF8 and FGFR1 LOF mutations cause CHH, it has been previously shown that both complete LOF and overexpression of FGF8 increase apoptosis in olfactory progenitor cells whereas a lesser decrease in functional FGF8 expression results in cell survival, suggesting nonlinear dosage effects for FGF8 signaling. Six out of eight (75%) IL17RD mutation subjects also displayed hearing loss, an observation consistent with FGF8’s role as a morphogen for otic development in both chickens and mice [98]. In 2 heterozygous IL17RD mutation subjects, additional missense variants in FGFR1 and KISS1R, two known CHH genes, were identified, thus attesting to an oligogenic mode of inheritance in these pedigrees [94].
- (iii)
Autosomal recessive KS
FEZF1, encoding FEZ family zinc finger 1
CCDC141, encoding a coiled-coil domain containing protein 141
In a consanguineous family with two affected KS subjects, whole exome sequencing (WES) and autozygosity mapping discovered 2 different homozygous mutations in FEZF1 (Missense: His278Tyr) and CCDC141 (Stop-codon: R724X) [99, 100]. In another consanguineous family, an additional homozygous frameshift mutation leading to a premature stop (Ala327fsX13) was identified whose transcript is likely to be sensitive for nonsense-mediated decay [99]. The transcriptional activity of the missense FEZF1 His278Tyr mutation was studied using a HEK293 cell assay with a Hes5p-DsRed reporter that was previously known to be repressed during cortical neurogenesis. This missense mutation resulted in intermediate accumulation of the mutated reporter compared to WT FEZF1 suggesting a partial LOF [99].
FEZF1 is a zinc-finger gene encoding a transcriptional repressor selectively present during embryogenesis in the olfactory epithelium [101–103], and Fezf1-deficient mice have impaired axonal projection of pioneer olfactory receptor neurons (ORNs) exhibiting a failure of their neurons to penetrate the basal lamina of the CNS [101–103]. These mice have smaller olfactory bulbs and have complete absence of GnRH neurons in the brain [102]. When these experiments were repeated with the basal lamina surgically removed, GnRH neurons were able to reenter the brain suggesting that the WT FEZF1 product may promote a protease expression that will enable the olfactory sensory neurons to make synaptic connections with the olfactory nerve layer of the olfactory bulbs, providing a conduit for the migration of the GnRH neurons into the brain [102]. The presence of two homozygous mutations in each of FEZF1 and CCDC141 in the first family suggested an oligogenic inheritance especially since the His278Tyr mutation was only a partial LOF mutation [99]. In a subsequent study, the same authors showed in murine models that Ccdc141 is expressed in GnRH neurons and olfactory fibers, and that knockdown of Ccdc141 reduces GnRH neuronal migration, suggesting that CCDC141 participates in the embryonic migration of GnRH neurons enabling them to form a hypothalamic neuronal network [95].
- (iv)
Indeterminate inheritance/oligogenic KS
SEMA3E, encoding semaphorin 3E protein
Similar to SEMA3A, semaphorin 3E is another secreted guidance cue of the class 3 semaphorin family [104]. Using a combination of exome sequencing and computational modeling, a recent study implicated a shared point mutation in exon 16 of the SEMA3E gene leading to a missense SEMA3E R619C Ig-like domain substitution in 2 KS siblings [105]. While recombinant wild-type SEMA3E protected maturing GnRH neurons from cell death by triggering a Plexin D1-dependent (PLXND1-dependent) activation of PI3 K-mediated survival signaling, recombinant mutant SEMA3E carrying the KS-associated mutation did not protect GnRH neurons from death [105]. In the same study, SEMA3E or PLXND1 deficiency in mice was shown to increase apoptosis of GnRH neurons in the developing brain, reducing GnRH-positive neurite innervation of the adult median eminence with phenotypic recapitulation of the human hypogonadal state. In addition to the SEMA3E mutations, a heterozygous p.F1019C mutation substitution in CHD7, a known CHH gene, was also present in the affected siblings, suggesting an oligogenic interaction [105]. The results from this single study strongly suggest a novel neurotrophic function for SEMA3E/PLXND1/PI3 K signaling in GnRH neuron development and a role for SEMA3E mutations in oligogenic inheritance of KS. Additional SEMA3E mutational screening in KS subjects will be required to evaluate the prevalence of SEMA3E mutations in CHH.
Genes Exclusively Causing nCHH
- (i)
Autosomal recessive nCHH
- a.
GNRHR, encoding the GnRH-receptor protein
A functional GnRH receptor (GNRHR) is crucial for both pubertal development and reproductive function. Indeed, hypothalamic GnRH secreted into the hypophyseal portal blood interacts with its high-affinity cognate GNRHR expressed in the cell membranes of gonadotrophs. Defects in the GNRHR emerged as the first autosomal cause of CHH [106, 107]. GNRHR is a G protein-coupled receptor with seven transmembrane segments that activates phospholipase C, leading to the intracellular increase in inositol phosphate [108]. While patients with a GNRHR mutation were expected to present with complete HH and unresponsiveness to GnRH stimulation, milder variants have been described. In the first family with a partially inactivating GNRHR mutation [106], the affected male had limited testicular growth (8 mL testes), detectable gonadotropins, and a normal response to a single pharmacologic dose of GnRH. Genetic analysis revealed a compound heterozygous mutation (Gln106Arg and Arg 262Gln substitutions). The parents were phenotypically normal, and each was heterozygous for one of the mutations. In CHO cells, Gln106Arg substitution (localized in the first extracellular loop of the GnRH receptor) markedly reduced the level of GnRH binding and stimulation of IP3 activity. In contrast, in the Arg262Gln substitution (localized in the third intracellular loop of the GnRH receptor), hormone binding was normal, but IP3 activation was impaired [106]. Several additional GnRH-R mutations (either homozygous or compound heterozygous) have been found which significantly impair GnRH binding and/or signaling to varying degrees [109]. Interestingly, a spectrum of pubertal development has been observed among CHH males with GNRHR mutations depending upon the genotype, ranging from the fertile eunuch syndrome [55] to the most severe form of GnRH deficiency characterized by cryptorchidism, microphallus, undetectable gonadotropins, and absent pubertal development [109]. In addition to autosomal recessive inheritance, the prevalence of heterozygous rare sequence variants in GNRHR has been shown to be significantly higher in CHH subjects. However, as expected, biallelic recessive GNRHR mutations resulted in more severe phenotypes when compared to the monoallelic heterozygous GNRHR mutation individuals who exhibit variable penetrance of phenotypes suggesting as-yet-unidentified genetic and/or environmental factors may combine with heterozygous LOF GNRHR alleles to produce reproductive phenotypes [109].
- b.
GNRH1, encoding gonadotropin-releasing hormone
The GnRH gene is located at 8p21–8p11.2 and is the most obvious autosomal candidate gene in CHH. Indeed, the hypogonadal (hpg) mouse, in which the GnRH gene has been homozygously deleted, presents with CHH [110]. Surprisingly, after several previous failed attempts to identify GNRH1 mutations, and 25 years after the discovery of the human sequence, homozygous frameshift mutations have now been reported in the GNRH1 gene. Bouligand et al. identified an insertion of a single base pair between the 6th and 7th codons of the GNRH1 gene that resulted in a frameshift that disrupted the primary sequence of the GnRH preprohormone starting at the 7th amino acid [111]. Indeed, cells transfected with the mutant GNRH1 allele failed to produce measurable amounts of GnRH [111]. Independently, Chan et al. [112] reported a single base pair deletion in the codon for the 6th amino acid of the GnRH decapeptide, that leads to a frameshift that disrupts the C-terminus of GnRH, a region essential for its function.
In both reports, GNRH1-deficient patients were born healthy at term countering the earlier speculation that GnRH may be essential for placental function and fetal survival. However, both patients had severe pubertal delay as well as micropenis and/or cryptorchidism, confirming the severity of these mutations. Moreover, no significant non-reproductive phenotypes were seen arguing against a major function for GnRH in other organ systems.
- c.
KISS1R, encoding the kisspeptin-1 receptor
In 2003, using linkage analysis and homozygosity mapping in inbred families, two groups independently identified inactivating mutations in KISS1R (then called GPR54), a G protein-coupled receptor, in nCHH patients [113, 114]. This observation, coupled with complementary mouse genetics, revealed that Kiss1r −/− mice were a faithful nCHH mouse model [114], providing compelling evidence that the KISS1R receptor and its cognate ligand, kisspeptin, were upstream gatekeepers of GnRH neurons. Since then, a number of mutations in the KISSR1 have been described in nCHH patients [115]. KISS1R is a member of the rhodopsin family of G protein-coupled receptors, and kisspeptin is the endogenous ligand for KISS1R and a potent stimulator of gonadotropin release in all mammalian species studied [116, 117]. Several key observations have emerged from the study of human GnRH-deficient subjects with KISS1R mutations. Neuroendocrine profiling of probands with KISS1R mutations reveal low-amplitude LH pulses [114, 118], suggesting some degree of persistence of endogenous but dampened GnRH secretion. In addition, in a subject with a KISS1R compound heterozygous mutation, exogenous GnRH induced a striking leftward shift in its GnRH-LH dose–response relationship suggesting some degree of endogenous pituitary priming by intact but dampened GnRH pulsatility [114]. Mutations in KISS1R account for ~2% of nCHH patients with the presence of cryptorchidism and/or micropenis in the majority of men with biallelic KISS1R mutations [119]. These observations strongly suggest that the kisspeptin signaling system is essential for prenatal GnRH secretion and early sexual development [119]. This observation is in keeping with a another published report of a male with KISS1R mutation who presented with cryptorchidism and micropenis in infancy, in whom neuroendocrine evaluation at 2 months of age revealed undetectable gonadotropins also suggesting a role for KISS1/KISS1R system in the “mini-puberty” of infancy [120]. While KISS1R inactivating mutations result in CHH, a single heterozygous activating KISS1R was shown to potentially contribute to central precocious puberty [121].
- d.
KISS1, encoding kisspeptin 1 peptideStay updated, free articles. Join our Telegram channel
Full access? Get Clinical Tree
