Fig. 5.1
Schematic structures of human TBK1-associated protein in the endolysosomes (TAPE), mouse TAPE and Drosophila Lethal (2) giant discs (Lgd). The identity and similarity of the amino acid sequence between human TAPE and mouse TAPE or Drosophila Lgd is analyzed. The structures of TAPE and Lgd contain four Drosophila melanogaster 14 (DM14) domains in the N-terminal region, coiled-coil motifs, and a C2 domain in the C-terminus
TAPE has been shown to be located in several subcellular compartments, including the nucleus, endolysosomes, and centrosomes, to perform its different biological roles. Lethal (2) giant discs (Lgd), a Drosophila ortholog of TAPE, is shown to regulate endosomal trafficking (Childress et al. 2006; Gallagher and Knoblich 2006; Jaekel and Klein 2006). Notch and other transmembrane proteins are accumulated in enlarged early endosomes in Lgd mutant cells. The C2 domain of Lgd is possibly involved in binding phospholipid. A genome-wide screening by small interference RNA (siRNA) indicates that TAPE is one of cellular factors involved in endocytosis (Collinet et al. 2010). Evidence from confocal microscopy reveals that TAPE is co-localized with an endosomal marker Rab5 and a lysosomal marker Lamp1 in mammalian cells (Chang et al. 2011). Furthermore, ectopic expression of TAPE mutants results in aberrant distribution of Rab5 and Lamp1 (Chang et al. 2011). Our unpublished results showed that recombinant TAPE, like other membrane-binding proteins, was able to bind several phospholipid species. These findings suggest a regulatory role for TAPE in endosomal trafficking. The ESCRT machinery is required for multivesicular body biogenesis, cytokinesis, virus budding, and autophagy (Hurley and Hanson 2010). Recent findings noted that both TAPE and Lgd utilize their DM14 domains to interact with CHMP4B, a component of the ESCRT-III complex in mammals and flies (Usami et al. 2012; Troost et al. 2012; Martinelli et al. 2012). Furthermore, TAPE negatively regulates CHMP4B function during HIV-1 budding (Usami et al. 2012). Together, these lines of genetic and biochemical evidence reinforce the importance of TAPE in regulating endosomal trafficking and membrane modification.
5.2.2 Roles of TAPE in the TLR3 and TLR4 Pathways
Early studies first demonstrated that TAPE is an activator of the NF-κB pathway (Matsuda et al. 2003; Zhao et al. 2010). Proteomics research indicates that TBK1 and TAPE are present in a signaling complex containing the nuclear hormone receptor co-activator SRC-2 (Jung et al. 2005). Subsequent biochemical analyses further confirmed that TAPE interacts with TBK1 and IKKε via its N-terminal region in mammalian cells (Chang et al. 2011). Ectopic expression of TAPE in mammalian cells leads to the activation of the IFN-β, NF-κB and Erk pathways, and the decrease of viral replication (Chang et al. 2011), implying a key role for TAPE in antiviral immunity. As a TBK1-interacting protein and its endosomal location, TAPE was then demonstrated to be involved in the endosomal TLR3 and TLR4 pathways but not other surface TLR pathways (Chang et al. 2011). TAPE is able to interact with Trif and potently enhance Trif-mediated IFN-β activation. Of interest, silencing of TAPE by siRNA impairs TLR3-mediated IFN-β activation but not Trif-mediated IFN-β activation, suggesting that TAPE functions upstream of Trif in the TLR3 pathway (Fig. 5.2). Likewise, silencing of TAPE blocks the TLR4–Trif pathway to IFN-β activation. Conversely, TAPE knockdown has no obvious blocking effect on MyD88-mediated or surface TLR-mediated NF-κB activation. These findings link the TAPE endosomal location to its regulatory role in the TLR3 and TLR4 pathways. Currently, the mechanistic mechanism by which TAPE links TLR3 and TLR4 to Trif-mediated downstream pathways has yet to be explored. Further research to confirm the in vivo function of TAPE in the TLR3 and TLR4 pathways is warranted.
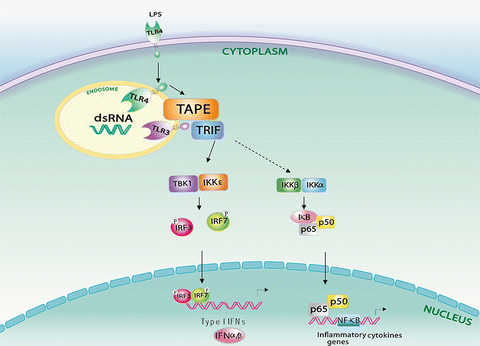
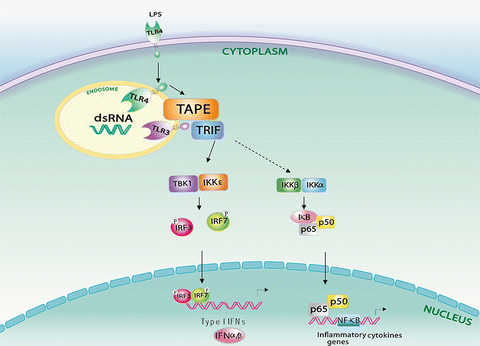
Fig. 5.2
Model for TBK1-associated protein in the endolysosomes (TAPE) in endosomal Toll-like receptor (TLR) 3 and TLR4 signaling. TAPE bridges activated TLR3 and endocytic TLR4 to Trif-mediated downstream signaling
5.2.3 Roles of TAPE in the RLR Pathways
Since TBK1 is a key protein kinase implicated in multiple PRR signaling mentioned above, it is tempting to speculate that TAPE might participate in other PRR pathways in addition to TLR3 and TLR4. A recent study provides biochemical and genetic evidence to reveal a functional role for TAPE in the RIG-I and MDA5 pathways (Chen et al. 2012). TAPE forms a complex with MAVS and RIG-I or MDA5 in mammalian cells. Silencing of TAPE impairs RIG-I and MDA5-mediated IFN-β activation but not MAVS-mediated IFN-β activation, suggesting that TAPE functions upstream of MAVS during RLR signaling. This kind of regulation was also confirmed by the genetic knockout approach. TAPE-deficient mouse embryonic fibroblasts and macrophages are impaired in type I IFN and inflammatory cytokine production upon RLR ligand stimulation. Furthermore, TAPE deficiency or knockdown diminishes cytokine production and antiviral immunity against RNA virus infection, including influenza A virus and vesicular stomatitis virus. These data reveal a vital role for TAPE in linking the RLR pathways to antiviral immunity. Further investigation using genetic knockout mice is essential to validate the in vivo role of TAPE in this kind of regulation. Studies from independent groups show that disruption of TAPE leads to a neonatal lethality in newborn mice (Chen et al. 2012; Zhao et al. 2011; Al-Tawashi et al. 2012). The conditional knockout approach will be an alternative to study the in vivo role of TAPE. Another key issue is the underlying mechanism of how an endolysosomal adaptor such as TAPE might participate in cytosolic RLR signaling. Two hypothetical models are proposed to explain this role of TAPE in RLR signaling (Fig. 5.3). One possibility is that upon signaling transduction, TAPE translocate onto mitochondria to couple RLRs to MAVS. The other possibility is that activated RLRs first engage with TAPE at endolysosomes and then form a complex with MAVS on mitochondria. Further research on this regulation is key to understanding the spatial and temporal control of RLR signaling.
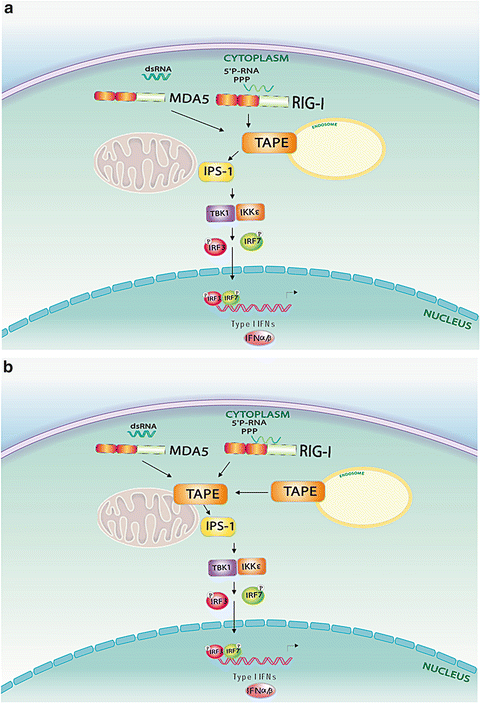
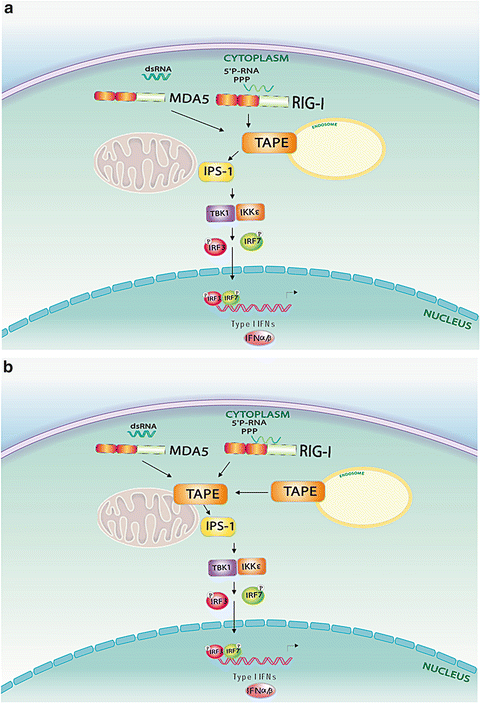
Fig. 5.3
Two proposed signalling models for TBK1-associated protein in the endolysosomes (TAPE) in retinoic acid-inducible gene (RIG)-I and melanoma differentiation-associated gene (MDA) 5 signaling. (a) TAPE engages with RIG-I and MDA5 at endosomes first, and then links them to MAVS-mediated downstream signaling at mitochondria. (b) Both TAPE and activated RIG-I-like receptors (RLRs) are recruited onto mitochondria to engage with MAVS for downstream signaling
5.3 Concluding Remarks and Perspectives
Identification of novel PRRs and regulators in the innate immune system has advanced our understanding of the activation of innate immunity over the past decade. In addition to plasma membrane, subcellular compartments such as endolysosomes, peroxisomes, and mitochondria have also emerged as signaling platforms for relaying innate immune signals. It is, however, less clear how PRRs and innate immune regulators interact with these subcellular compartments to enact their roles in innate immune sensing and signaling during pathogen infection. Pathogens frequently invade host cells to utilize different subcellular compartments or organelles to facilitate their propagation. Thus, it is also important to understand how pathogens develop strategies to target innate immune regulators or subcellular compartments to intercept innate immune signaling. TAPE is an endolysosomal adaptor recently shown to involve in the endosomal TLR (TLR3 and TLR4) and cytosolic RLR pathways. Several features regarding this TAPE-mediated innate immune regulation are of interest. First, it is noted that TAPE acts upstream of Trif/Ticam-1 or MAVS to regulate TLRs or RLRs, respectively. To the best of our knowledge, TAPE is the first regulator implicated in both the endosomal TLR and cytosolic RLR pathways at such an early step. The second feature is that TAPE interacts with the different innate immune sensors and regulators mentioned above (Chang et al. 2011; Chen et al. 2012) and other host and viral factors (our unpublished results). These proteins share very little similarity in structural features. Thus, it is unlikely that TAPE uses its limited protein domains to directly interact with diverse host and viral proteins; instead, it may interact with them through a common subcellular compartment such as endolysosomes. The third feature is that although TAPE is a relatively weaker IFN-β activator, it potently enhances the activation of IFN-β by other innate immune regulators, including Trif/Ticam-1, MAVS, and TBK1. Taken together, these features suggest that TAPE represents a new type of innate immune regulator, which controls a key cellular mechanism essential for both endosomal TLR signaling and cytosolic RLR signaling. Several outstanding questions need to be addressed to appreciate the importance of TAPE in innate immunity. First of all, in vivo functions of TAPE in the TLR3, TLR4, and RLR pathways have to be further confirmed by the conditional knockout approach. Secondly, it is critical to explore the mechanistic mechanisms by which TAPE regulates these TLR and RLR pathways. Particular attention is needed to reconcile the current idea of RLR signaling, centered on MAVS-located mitochondria, with the emerging RLR signaling model (Fig. 5.3), which is involved in TAPE-containing endolysosomes. Lastly, it will be interesting to explore the potential roles of TAPE in other innate immune pathways, such as endosomal TLR7/8, TLR9, and cytosolic DNA sensors. Better understanding of TAPE-mediated innate immune signaling may reveal novel regulatory mechanisms in innate immunity, and also provide insights into targeting the TLR and RLR pathways when they are deregulated in infectious or inflammatory diseases.
References
Al-Tawashi A, Jung SY, Liu D, Su B, Qin J (2012) Protein implicated in nonsyndromic mental retardation regulates protein kinase A (PKA) activity. J Biol Chem 287(18):14644–14658. doi:10.1074/jbc.M111.261875 CrossRefPubMedCentralPubMed
Barton GM, Kagan JC (2009) A cell biological view of Toll-like receptor function: regulation through compartmentalization. Nat Rev Immunol 9(8):535–542. doi:10.1038/nri2587 CrossRefPubMedCentralPubMed
Blasius AL, Arnold CN, Georgel P, Rutschmann S, Xia Y, Lin P, Ross C, Li X, Smart NG, Beutler B (2010) Slc15a4, AP-3, and Hermansky-Pudlak syndrome proteins are required for Toll-like receptor signaling in plasmacytoid dendritic cells. Proc Natl Acad Sci U S A 107(46):19973–19978. doi:10.1073/pnas.1014051107 CrossRefPubMedCentralPubMed
Bozym RA, Delorme-Axford E, Harris K, Morosky S, Ikizler M, Dermody TS, Sarkar SN, Coyne CB (2012) Focal adhesion kinase is a component of antiviral RIG-I-like receptor signaling. Cell Host Microbe 11(2):153–166. doi:10.1016/j.chom.2012.01.008 CrossRefPubMedCentralPubMed
Broz P, Monack DM (2013) Newly described pattern recognition receptors team up against intracellular pathogens. Nat Rev Immunol 13(8):551–565. doi:10.1038/nri3479 CrossRefPubMed
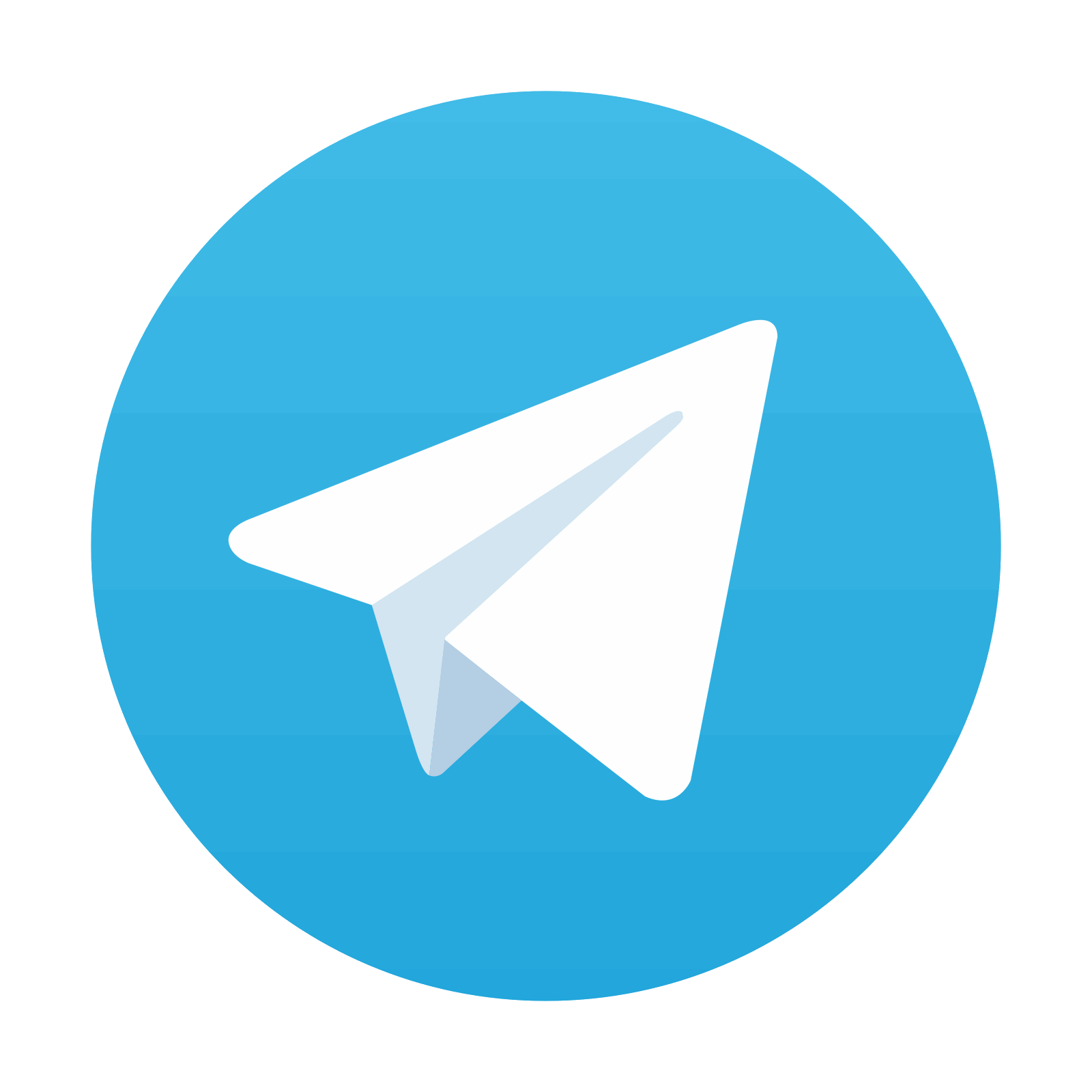
Stay updated, free articles. Join our Telegram channel

Full access? Get Clinical Tree
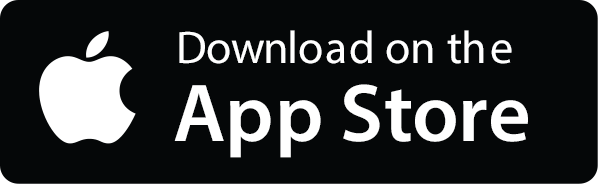
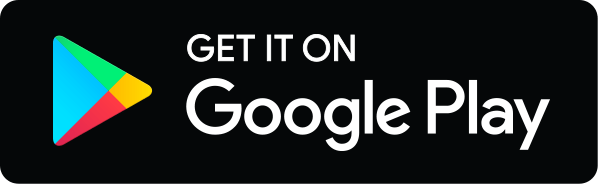