CRITICAL CARE OF CENTRAL NERVOUS SYSTEM INFECTIONS
JOHN ZURASKY, THOMAS O. MCPHARLIN, AND KYRA J. BECKER
Persons with infections of the central nervous system (CNS) are at risk for developing serious medical and neurologic complications related to their infection and often require a level of care that can only be delivered in an intensive care environment. Despite advances in care, infections of the CNS are still associated with significant morbidity and mortality. For broad ranges of medical and surgical disease, studies suggest that outcome from critical illness is improved when care is delivered by practitioners with specialized training and expertise in the condition being treated; these findings also extend to the treatment of patients with neurologic illnesses like stroke, intracranial hemorrhage (ICH), and subarachnoid hemorrhage (SAH) (1–5). Specific studies to assess the benefit of specialized care by neurointensivists in patients with CNS infections have not been done, but a benefit for this patient population also seems likely because the secondary response of the brain to injury is usually stereotyped and independent of the initial insult. Principles of the medical and neurologic management of CNS injury can therefore be generalized across broad populations of patients with different pathologic processes affecting the CNS.
STROKE AS A COMPLICATION OF MENINGITIS
Thrombosis of the vessels due to vascular inflammation leads to stroke in up to 25% of patients with bacterial meningitis and appears to be more common in pneumococcal meningitis than meningitis due to other bacteria (6–8). Curiously, lower cerebrospinal fluid (CSF) white blood cell counts appear to be prediction of infarction (7,8). Deep infarction due to inflammation of the lenticulostriate vessels is a common sequela of cryptococcal and tuberculous meningitis; stroke is seen in approximately 30% of patients with tuberculous meningitis (9,10). Patients with cryptococcal meningitis may also develop significant intracranial hypertension (11). Aspergillus is an angioinvasive fungus that can predispose to both ischemic and hemorrhagic strokes (12). Stroke is also seen in patients with cerebral toxoplasmosis, zoster vasculitis, and a variety of other more rare infections (13).
GENERAL PRINCIPLES GUIDING NEUROLOGIC CRITICAL CARE
Among the key principles that guide the care of the brain-injured patients are the relationships between cerebral blood flow (CBF), intracranial pressure (ICP), and mean arterial blood pressure (MABP); an understanding of the physiologic processes that regulate these variables is crucial for providing care to patients with neurologic illness. The CBF is determined by the cerebral perfusion pressure (CPP) and the cerebrovascular resistance (CVR). The CPP represents the difference between the MABP and the ICP.
CBF = CPP ÷ CVR
CPP = MABP − ICP
In cortical gray matter, the CBF is maintained constant at 50 mL/100 g of tissue per minute and is independent of the CPP (and hence MABP) over a broad range of values because of changes in the CVR, a process known as cerebral autoregulation (14). In normotensive individuals, autoregulation is lost when the MABP exceeds 150 mm Hg (15). Loss of cerebral autoregulation due to increased blood pressure is referred to as hypertensive encephalopathy. The ability to adequately regulate CBF is similarly lost in normotensive individuals when the MABP drops below 50 mm Hg (16). At MABPs less than 50 mm Hg, CBF decreases, and ischemia ensues. Under usual circumstances in a normotensive (120/80 mm Hg) individual, the CPP is approximately 83 mm Hg (assuming an MABP of 93 mm Hg and an ICP of 10 mm Hg). Thus, if the ICP increases to 50 mm Hg and MABP does not change, the CPP will become dangerously low (CPP = 93 mm Hg [MAPB] − 50 mm Hg [ICP] = 43 mm Hg) and cerebral ischemia will result. Perhaps more importantly, the ability to regulate CBF is lost in the injured brain (17,18); the CBF therefore becomes passively dependent on the CPP (and thus the MABP).
Another key principle that guides care of the neurologically ill patient is the pressure–volume relationship of the intracranial cavity. According to the Monro-Kellie doctrine, the skull is nondistensible and the contents of the intracranial cavity (brain, blood, and CSF) are noncompressible; any increase in the volume of one of the components of the intracranial cavity will lead to displacement of another, an increase in ICP, or a combination of the two (19). As a general rule, the brain accounts for approximately 80% of the intracranial contents and the CSF accounts for another 10%. Intravascular blood (blood within the arterial and venous circulation) comprises the remaining 10% of the intracranial contents. The ICP will therefore increase if there is an increase in the volume of CSF (hydrocephalus), intravascular blood (hypertensive encephalopathy, hyperemia, or cerebral vein thrombosis), extravascular blood (ICH), or interstitial fluid (edema). Each of these situations can occur in persons with infections of the CNS, and the guiding principles for treatment will be discussed.
HYDOCEPHALUS
High levels of protein and pleocytosis characterize the CSF that accompanies an infection of the brain. Both the elevated protein and the white blood cells impair CSF reabsorption through the arachnoid granulations and may lead to communicating hydrocephalus. In addition, pathogens themselves, especially Cryptococcus, may obstruct the arachnoid granulations. In patients with intracranial hypertension due to communicating hydrocephalus, the ICP can be lowered through serial lumbar punctures with drainage of high volumes of CSF; in some instances, a lumbar drain may be placed. If the flow of CSF through the third ventricle or the aqueduct of Sylvius is impaired (i.e., noncommunicating hydrocephalus), lumbar puncture is contraindicated and external ventricular drainage may be necessary. Even after successful treatment of acute bacterial or fungal meningitis, hydrocephalus may persist. For patients with communicating hydrocephalus, a finite number of lumbar punctures may be all that is needed to control the ICP. In some individuals with persistent communicating hydrocephalus and in all of those with persistent noncommunicating hydrocephalus, continuous extracranial shunting of CSF with a ventriculoperitoneal shunt (VPS) may be required. Whenever possible, placement of a VPS should be delayed until the CSF protein and cell count return to normal.
CEREBRAL VEIN THROMBOSIS
If the venous outflow of blood from the brain is impaired, there will be an increase in the volume of intravascular blood within the intracranial cavity because arterial inflow continues. Headache is the most common presenting feature of cerebral vein thrombosis (CVT), but given that headache is also seen routinely in persons with meningitis, this clinical feature is of little diagnostic value in the patient with CNS infection. When thrombosis occurs in the large draining veins of the brain, such as the sagittal or sigmoid sinus, increased ICP, venous infarction, hemorrhage, and seizures may occur. With the advent of modern antibiotic therapy, infections are no longer a common cause of CVT, but the possibility of CVT should still be considered in patients with meningitis, especially those who develop extensive cerebral infarction that does not respect a defined arterial territory. The one clinical situation in which CVT still occurs with some frequency is in mastoiditis where the transverse and sigmoid sinuses are particularly prone to thrombosis (Fig. 49.1). Thus, in patients who present with infections of the inner ear and mastoid sinuses, vigilance for CVT must be maintained.
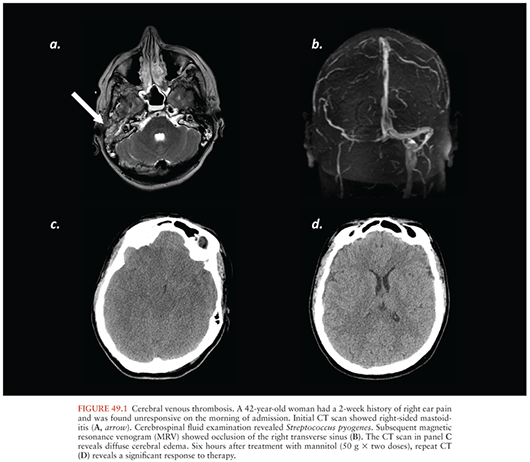
The diagnosis of CVT is now made most reliably by magnetic resonance venography (MRV) or computed tomography venography (CTV). Either MRI or computed tomography (CT) done with contrast may be diagnostic in sagittal sinus thrombosis because the contrast will not opacify the torcular herophili and thus reveals what is referred to as an “empty delta sign” on axial imaging. Noncontrast CT and MRI may also show clot within the sagittal sinus or other large veins. Gradient-echo (GRE) sequences on MRI may also reveal clot within the large veins and sinuses. In rare cases, conventional intraarterial angiography may still be needed to diagnose CVT. Systemic anticoagulation is generally the treatment of choice for CVT, even in patients with hemorrhagic infarcts (20,21). Endovascular clot retrieval and local thrombolysis have been used to treat patients with extensive CVT, but the use of these interventions remains unclear (21).
EDEMA
Independent of the mechanism of injury, an inflammatory response occurs within the brain as a result of that injury and contributes to it. In persons with infections of the CNS, however, the inflammatory response is generally the primary cause of brain injury. Inflammation represents the first or innate response of the immune system to infection or a breach of the body’s normal protective barriers. The inflammatory response in the brain is similar to that in other tissues, but the consequences can be much different. With inflammation, there is increased blood flow and compromise of the vascular integrity (blood–brain barrier [BBB]), leading to extravasation of fluid into the brain tissue as well as an influx of leukocytes into that tissue. Activated leukocytes release a number of biologically active compounds, including cytokines and toxic oxygen metabolites, that further compromise the integrity of the vasculature/BBB. These substances may also be neurotoxic. As a consequence of BBB breakdown and loss of microvascular integrity, cerebral edema occurs. Increasing cerebral edema leads to increasing ICP, and if severe enough, herniation and death. In meningitis, the infectious process, and therefore the inflammatory response, affects the meninges and underlying brain diffusely. Cerebral edema and the increase in ICP are therefore globally distributed without significant compartmentalization; thus, if herniation occurs, it is usually characterized by a central downward herniation of the brain through the tentorial incisure and foramen magnum. In herpes simplex encephalitis, however, because the temporal lobes are preferentially involved, uncal or transtentorial herniation is common. Patients with focal mass lesions, such as abscesses, are also prone to compartmental shifts and transtentorial herniation.
TREATMENT OF INTRACRANIAL HYPERTENSION
Intracranial hypertension contributes significantly to both the morbidity and mortality of patients with CNS infections (22–25). There is, however, no evidence to show that ICP monitoring translates into clinical benefit in these patients. Thus, clinical judgment must substitute for an evidence-based approach to therapy in patients with complicated CNS infections. If an ICP monitor is in place, the ICP, and hence CPP, are known. Knowledge of the ICP, however, should never replace for the neurologic examination. In patients with processes affecting their temporal lobes, for instance, uncal herniation can occur in spite of a normal ICP.
In patients at risk for herniation due either global or local increases in ICP, several simple measures may be taken in the intensive care unit (ICU) to help decrease ICP. First, the head of the bed should be elevated approximately 30 degrees above the horizontal plane to enhance cerebral venous outflow through the jugular veins. Second, ventilation should be optimized to maintain a normal arterial content of CO2 (PaCO2). Hypoventilation results in retention of CO2, which leads to an increase in CBF and therefore cerebral blood volume (CBV); an increase in CBV translates into an increase in ICP if compensatory mechanisms fail. Within the physiologic range, an increase in PaCO2 of 1 mm Hg leads to approximately a 4% increase in CBF and a 1% to 2% increase in CBV (26). Similarly, a 1 mm Hg decrease in PaCO2 leads to approximately a 4% decrease in CBF and a 1% to 2% decrease in CBV. In patients at risk of imminent death from herniation, hyperventilation may therefore be life saving because it will decrease the CBV and thus the ICP, but it does this at the expense of CBF. Clinical studies suggest that hyperventilation is detrimental and hypocapnia should be avoided or normalized as soon as possible (27,28).
In patients with CNS injury of any type, including infectious insults, hyponatremia is common. The relative contribution of the syndrome of inappropriate antidiuretic hormone (SIADH) secretion and cerebral salt wasting (CSW) to hyponatremia in patients with brain injury is debated. To make a diagnosis of SIADH, patients must be either hypervolemic or euvolemic; for a diagnosis of CSW, patients should be relatively hypovolemic and have evidence of excess sodium in their urine. Irrespective of the cause of hyponatremia, the situation can be particularly dangerous in patients with brain injury. Sodium is the primary determinant of plasma osmolality, as illustrated by this equation:
plasma osmolality (mOsm/kg) = 2 [Na+] + ([glucose] 4 18) + ([urea] 4 2.8)
The plasma osmolality determines the osmotic pressure or the force per unit area that must be applied to prevent water movement from an area of low solute concentration to an area of high solute concentration (osmosis). If the osmolality, and hence osmotic pressure, of plasma increases, it prevents the movement of water into the injured tissue with a lower osmolality. Further, if the osmolality (and hence osmotic pressure) of the injured tissue is lower than that of plasma, water will move from the brain into the more concentrated plasma, thereby dehydrating the brain and lowering ICP. Based on this equation, it is obvious that even small changes in plasma [Na+] can have profound effects on the plasma osmolality, and thus ICP. Plasma sodium should therefore be normalized in persons with cerebral edema, which is most easily accomplished by the administration of isotonic fluids. In instances of life-threatening cerebral edema, the plasma sodium can even be increased by administration of hypertonic fluids such as 3% saline. The osmolality of commonly used fluids is shown in Table 49.1. Given the large amounts of fluids required for administration of antibiotics to critically ill patients, in some instances, it may be advantageous to have the pharmacy mix intravenous medications in normal saline when possible. Because most patients in the ICU will receive nutrition in the form of tube feeding, choosing an alimental formula with minimal free water will also help to prevent hyponatremia.
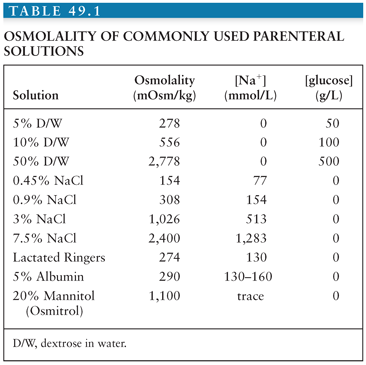
Normalization of plasma [Na+] is important for patients with CNS mass lesions and for patients with cerebral edema. Hyponatremia, and hence low plasma osmolality, allows for the movement of water from plasma into brain, exacerbating cerebral edema and increasing ICP. In patients with life-threatening cerebral edema, more extreme measures may be undertaken. Traditional osmotherapy is that of mannitol, and intermittent dosing of mannitol can have profound effects (Fig. 49.1C and D). For treatment of acute herniation, the standard dose of mannitol is 1 g/kg as a single bolus. Intermittent bolus dosing at doses of 0.25 g/kg to 0.50 g/kg also is used. Administration of hypertonic saline, either continuously or as a bolus, can also be used to drive the plasma [Na+], and thus the plasma osmolality, to abnormally high values to treat life-threatening cerebral edema. A prolonged increase in plasma osmolality results in the creation of idiogenic osmoles in the brain to alleviate the osmotic stress. Rapid normalization of plasma osmolality can thus result in “rebound edema.” It is thus important to normalize plasma osmolality over the course of several days to prevent the movement of water from plasma into brain tissue containing high concentrations of these idiogenic osmoles.
In patients with cerebral edema that is refractory to medical therapy, decompressive hemicraniectomy may be life saving. Decompressive hemicraniectomy allows for normalization of the ICP and pressure gradients within the intracranial cavity and thereby prevents herniation. Blood flow through the pial vessels may be compromised by increased ICP; a decompressive surgery will therefore improve blood flow and may lessen ischemic brain injury. Decompressive hemicraniectomy has been used to treat medically intractable intracranial hypertension in patients with meningoencephalitis (29–32).
GENERAL INTENSIVE CARE UNIT CARE
Blood Pressure
In patients with pneumococcal meningitis, concomitant sepsis is common. Meningitis caused by other bacterial pathogens may also be accompanied by bacteremia and, potentially, septic physiology. If significant hypotension is present, the CBF may become compromised, especially if the ICP is elevated. Care must therefore be taken to ensure that patients are euvolemic. As a rule, a MABP of at least 90 mm Hg should be maintained to ensure adequate CBF, using intravenous fluids and vasopressors as needed. In persons with marked cerebral edema, the MABP needs to be even higher to ensure that the CPP is sufficient to maintain CBF. Despite the lack of clinical evidence, ICP monitoring may be useful in patients with diffuse cerebral edema to ensure that CPP is adequate.
Glucose
Dexamethasone administration is beneficial in the treatment of meningitis, but this benefit has only been proven in the developed world for patients infected with Streptococcus pneumoniae (33). Hyperglycemia is common in persons stressed with serious medical illness and perhaps even more common in persons with brain injury; administration of dexamethasone will only increase the incidence and severity of hyperglycemia. Experimental studies show that hyperglycemia exacerbates brain injury, and observational studies show an association between hyperglycemia and increased morbidity and mortality following ischemic stroke, hemorrhagic stroke, subarachnoid hemorrhage, head trauma, and cardiac arrest (34–38). Whether or not normalization of glucose improves outcome in patients with brain injury, and patients with CNS infections in particular, is unknown. There is no consistent evidence that intensive insulin therapy improves outcome in critically ill patients, but the data are clear that intensive insulin therapy increases the risk of hypoglycemia and death (39–41). The only specific recommendations about glucose management that can be made in critically ill patients with brain injury—be it ischemic, traumatic, or infectious—are to avoid hypoglycemia and the extremes of hyperglycemia.
Fever
Fever is a classic component of the immune response, and hyperthermia augments the function of immune cells (42). The consequences of the immune response in the CNS, however, may be deleterious, as already discussed. Furthermore, fever is associated with increased ICP, and even mild elevations in body temperature predict poor outcome from brain injury (43). There is laboratory evidence of benefit for hypothermia in almost all forms of brain injury, and hypothermia is an effective treatment for elevated ICP (44). The clinical benefits of hypothermia, however, are only proven in the setting of cardiac arrest (45,46). Because induction of hypothermia is difficult and hypothermia may suppress the beneficial as well as the detrimental aspects of the immune response, hypothermia cannot yet be recommended as routine adjunctive therapy for patients with CNS infections. Aggressive maintenance of normothermia, however, should be strived for in these patients.
Delirium
Delirium is defined as fluctuation in the level and content of consciousness. Up to 80% of mechanically ventilated patients will develop delirium while in the ICU (47); the incidence of delirium in critically ill patients with infections of the CNS is likely higher. Patients who experience delirium have increased lengths of stay, increased rates of admission to long-term care facilities, and long-term impairment in cognitive function abilities (47,48). Further, delirium is associated with increased mortality (47,49).
Delirium, by definition, is related to a medical condition or side effects from medications. Prevention of delirium may be difficult in patients with CNS infections, where the brain is directly impacted by the pathogenic organisms, seizures are common, metabolic perturbations (like hyponatremia) are frequent, and sepsis may occur. These conditions in and of themselves can cause delirium, as can many of the drugs used to treat the conditions (i.e., antibiotics, anticonvulsants, sedative/hypnotic medications). Delirium is more common with advancing age, infection, metabolic acidosis, use of sedatives and analgesics, and uremia; the most important risk factors, however, are the presence of coma at the time of initial admission to the ICU (drug induced or other organic) and having an underlying neurologic diagnosis (50). To prevent delirium, aggressive correction of underlying medical conditions is paramount as is minimizing polypharmacy. In a single study, prophylactic haloperidol did not prevent delirium but did reduce the duration of delirium (51). The use of dexmedetomidine, as opposed to other sedatives, may be associated with less risk of delirium, but its use is limited by bradycardia and hypotension (52).
Patients should be screened for delirium routinely using a validated scale such as the Confusion Assessment Method for Intensive Care Unit (CAM-ICU), the Intensive Care Delirium Screening Checklist (ICDSC), the Richmond Agitation-Sedation Scale (RASS), or the Riker Sedation-Agitation Scale (SAS) (53,54). Once delirium occurs, the mainstay of pharmacologic therapy is the use of antipsychotics (55,56). Haloperidol is the most commonly used antipsychotic, but other antipsychotic agents, such as quetiapine and risperidone, may also be used. The major dose-limiting side effect of haloperidol is prolongation of the QT interval, which can lead to torsades de pointes; patients treated with high doses intravenous haloperidol should thus have daily electrocardiograms (ECGs). Nonpharmacologic therapies include early mobilization and physical therapy, which has been shown to shorten the duration of delirium and improve outcomes (57). For sedated and mechanically ventilated patients, daily trials of awakening and spontaneous breathing should also be undertaken (58).
SEIZURES IN THE INTENSIVE CARE UNIT
Anything that irritates the cortex of the brain, including inflammation of pial vessels or cortical infarction, may precipitate seizures. Seizures often occur in persons with meningitis and are even more common in persons with encephalitis (23). In addition to the underlying infectious process that precipitates seizures, hyponatremia, which is common in persons with CNS infections, will further lower the seizure threshold in an already irritable brain. Moreover, many antibiotics used for the treatment of CNS infections may also lower the seizure threshold (59). The risk of antibiotic-associated seizures is increased in patients with CNS pathology, renal insufficiency, and in those who inadvertently receive excessive doses of the antibiotic (59). Independent of seizures, some antibiotics may also cause encephalopathy; the risk factors for encephalopathy are similar to those for seizures. Table 49.2 provides a list of CNS toxicity associated with antibiotics. A review of all medications should therefore be performed daily in the ICU. Drugs with potential to cause seizures should be used with caution in patients at risk for convulsions and should be discontinued in patients who are actively seizing or at high risk for seizing.
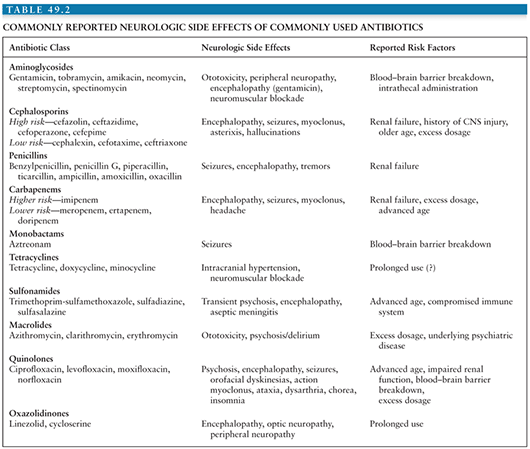
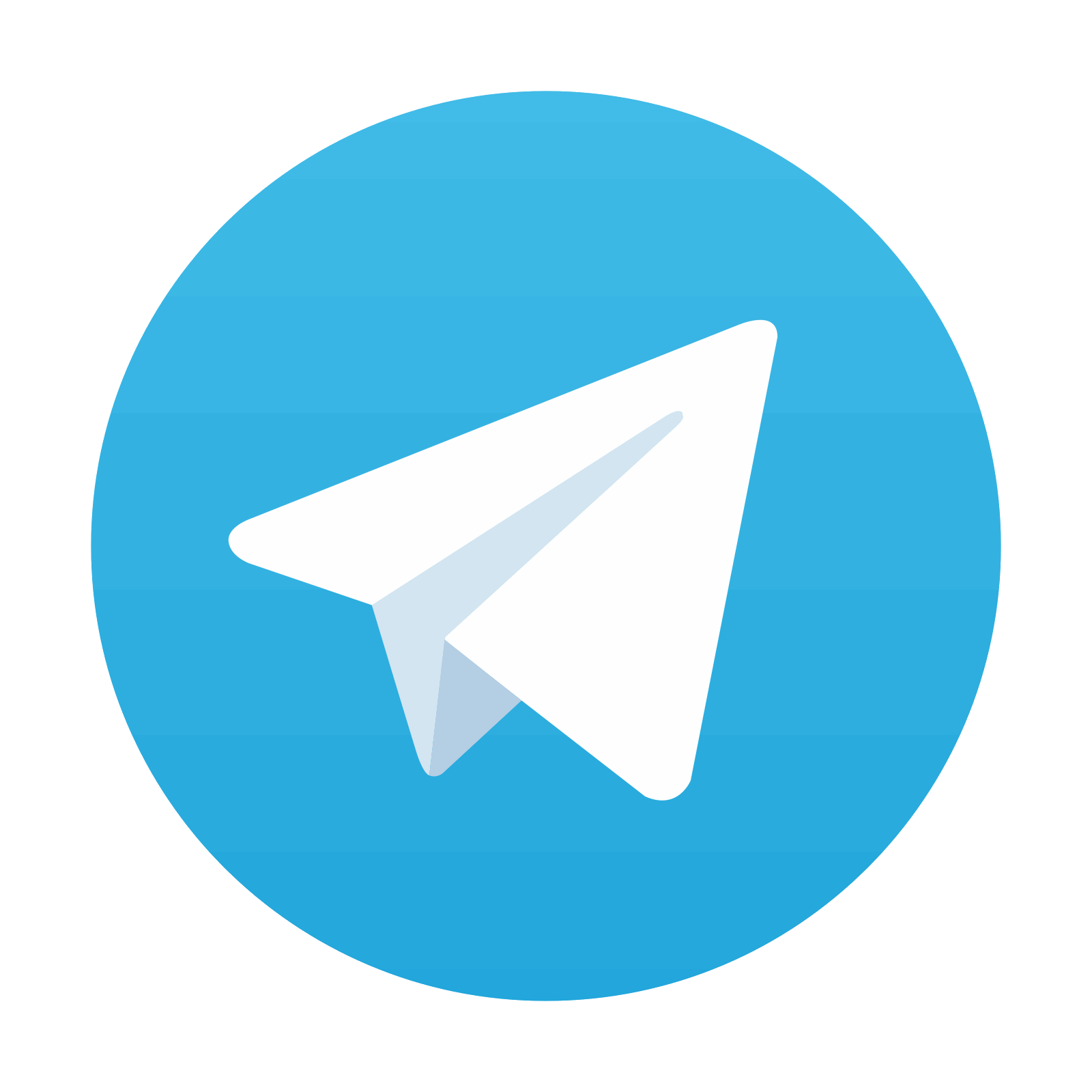
Stay updated, free articles. Join our Telegram channel

Full access? Get Clinical Tree
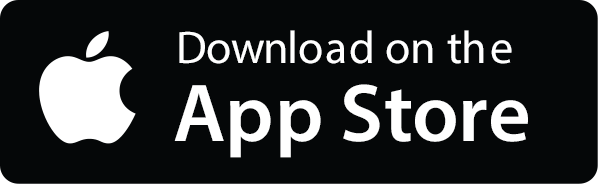
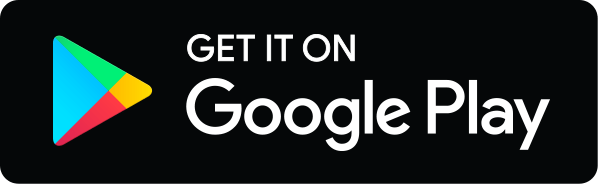