Fig. 3.1
Proximity of enteric cholinergic and adrenergic nerve fibers to IgA-expressing plasma cells and secretory component (SC)-expressing epithelial cells in porcine ileum. Confocal micrographs are representative of observations in a minimum of three transverse tissue sections from each of five pigs. (a) Nerve fibers immunoreactive for the neural marker PGP 9.5 (red) are in close proximity to both IgA-immunoreactive plasma cells (green) and epithelial cells immunoreactive for SC (blue) in the ileal crypts. (b, c) Higher magnification shows nerve fibers (arrowheads) immunoreactive for the vesicular acetylcholine transporter (red, b) and dopamine beta-hydroxylase (red, c) closely apposed to B lymphocytes immunoreactive for IgA (green) and epithelial cells immunoreactive for SC (blue). Cr ileal crypt, L intestinal lumen, SM submucosa. Scale bars: (a) 100 μm; (b, c), 15 μm (L.D. Schmidt, L. Vulchanova and D.R. Brown, unpublished data)
3.2.2 A Few Words About Catecholamine Receptors
Norepinephrine, EPI and DA activate their cognate G protein-coupled receptors expressed on neurons, epithelial cells and other types of target cells which influence overall mucosal function and alter intestinal susceptibility to infection. Receptors for NE and DA have been defined over several decades through the use of highly selective receptor agonists and antagonists in functional pharmacological investigations and more recently, through molecular cloning and structure-function studies in isolated cells and transgenic animals.
The receptor concept was developed in the early twentieth century on the translational approach of defining binding interactions of endogenous substances or their synthetic homologs with specific sites (“receptive substances”) expressed by target cells that are linked to a biological response (Rang 2006). Biochemical analyses of selective binding site interactions with catecholamine-like drugs and other ligands that were developed many years later have provided crucial information on the affinities (Kd, dissociation constant) and competitive interactions of catecholamines and their synthetic derivatives at specific binding sites. However, these biochemical studies do not measure the biological activity of the ligands examined, and therefore they do not truly define a “receptor”, which is an entity functionally coupled to intracellular signal transduction pathways that mediates a biological function. In other words, biochemical analyses of binding sites cannot distinguish between agonist and antagonist ligands. Binding studies, if carefully executed, can provide information on a specific, high-affinity binding site for an endogenous or synthetic ligand that can serve as supporting evidence for the presence of a true receptor in a biological system. Through GTPγ35S binding assays (Harrison and Traynor 2003), it is possible to assess the effectiveness of ligands to activate G proteins coupled to a particular receptor and this approach affords a better approximation of ligand activity at the cellular level (e.g. it is possible to distinguish an agonist from an antagonist).
Functional criteria for a receptor-mediated effect include (1) selective antagonism, (2) a rank order of ligand potency (usually based on the 50 % effective concentration, EC50 for agonists or a pKB value for antagonists, which is determined through rightward shifts in an agonist concentration-effect relationship after pretreatment with a competitive antagonist) that is consistent with the pharmacological signature of a particular receptor type; and (3) stereospecificity (e.g. the levorotatory isomer of NE is much more potent than its dextrorotatory counterpart at ARs). The affinities and competitive interactions among ligands for ARs and dopamine receptors (DARs) measured by functional drug interactions are generally well correlated with the affinities and interactions of the same ligands determined biochemically at specific binding sites.
Presently, ARs are classified into alpha- and beta-AR types; this includes two subtypes of alpha-ARs (alpha 1 and alpha 2) having three isoforms each, and three subtypes of beta-ARs. An EPI-preferring beta 2-AR was the first drug receptor to be cloned and characterized in the modern era of molecular pharmacology (Dixon et al. 1986). Compared to EPI, NE has relatively higher binding affinity for alpha-ARs and beta 1– and beta 3-ARs, but a lower affinity for beta 2-ARs. Two main DAR types exist through gene duplication events in the vertebrate lineage (DA1R and DA2R); from these, five receptor subtypes have been defined. Our understanding of the nature of these receptors and their relationships to G proteins and downstream intracellular signaling cascades continues to grow. For example, there is accumulating evidence that ARs may form dimeric complexes on cell membranes that possess a pharmacological profile different from that of the monomeric receptor (Verburg-van Kemenade et al. 2013). Moreover, beta-ARs can signal differentially through intracellular G proteins and beta-arrestin, and thereby produce different ligand-dependent biological effects (Bock et al. 2014). This should be kept in mind when designing experiments involving the prolonged contact of catecholamine agonists with their receptors, as long-term stimulation of the receptor can often result in its phosphorylation, which in turn can increase interactions of the receptor with arrestin or other intracellular proteins associated with receptor down-regulation. The investigator is then left with the question of whether the biological effects examined were the result of receptor activation or the loss of receptors from cell membranes.
3.2.3 Localization of Catecholamine Receptors on Mucosal Epithelial Cells
As indicated above, pharmacologically-defined catecholamine receptors influencing epithelial function may be expressed on epithelial cells or on nerves innervating these cells. Specific, high-affinity binding sites for catecholamines have been detected on mucosal epithelial cells in some species, including the guinea pig and rat. These include alpha 1-, alpha 2– or beta-adrenergic binding sites as well as DA and non-adrenergic imidazoline binding sites (Chang et al. 1983; Nakaki et al. 1983; Cotterell et al. 1984; Paris et al. 1990; Senard et al. 1990; Valet et al. 1993; Baglole et al. 2005). Food deprivation appears to increase alpha 2-AR binding sites on rat jejunal epithelial cells (Lucas-Teixeira et al. 2000) and pro-inflammatory cytokines and short-chain fatty acids generated by colonic bacteria may decrease the rate of alpha 2-AR gene transcription and hence, the number of binding sites in human HT-29 colonic epithelial cells (Devedjian et al. 1996; Cayla et al. 2008).
In other species, alpha-ARs modulating epithelial functions appear to be expressed solely on nerve membranes, at least in some regions of the intestinal tract. Synaptosomal preparations enriched in 3H-saxitoxin binding sites were obtained from the submucous neural plexuses of the canine and porcine small intestine. In these neural preparations, saturation analyses indicated that highly-selective alpha2-AR antagonists bound specifically to these enteric neural membranes with a dissociation constant (KD; a measure of ligand affinity) of 3.5 nM [3H-rauwolscine, dog; Ahmad et al. 1989] and 0.4 nM, respectively [3H-yohimbine, pig; Hildebrand et al. 1993]. In a study with porcine small intestine, specific binding of 3H-yohimbine to intestinal epithelial cells was minimal (Hildebrand et al. 1993). On the other hand, in the porcine colonic mucosa, the functional effects of catecholamines on electrolyte transport are mediated by alpha 1-ARs and are resistant to the neural conduction blocker tetrodotoxin, indicating that these substances may act on epithelial cell receptors in this gut segment (Traynor et al. 1991). Whether or not specific alpha1-AR binding sites exist on colonic epithelial cell membranes in pigs has not been determined.
3.3 Catecholamines and Mucosal Interactions with Bacteria
Both NE and DA have been shown to alter the mucosal attachment or invasiveness of bacterial pathogens such as enterohemorrhagic Escherichia coli (EHEC) or serovars of Salmonella enterica by acting on the intestinal mucosa. The actions of these catecholamines on bacteria-mucosa interactions have been examined in mucosal explants from murine or porcine intestine mounted in Ussing chambers (Brown and O’Grady 2008). This apparatus has been used for decades in studies of transepithelial ion transport and more recently, in investigations of bacteria on intestinal transport (Lomasney and Hyland 2013). The Ussing chamber system extends the viability of mucosal explants under quasi-physiological conditions, allows for tangential flow of bacteria across a fixed mucosal surface area, and permits the selective contact of drugs and bacteria with the luminal or contraluminal surfaces of intestinal tissues. Epithelial cells in this system retain viability and active transport properties for periods >3 h (Söderholm et al. 1998). Alternative systems for measuring bacterial interactions with mucosal tissue, such as in vitro organ culture techniques (Hicks et al. 1996), rely on static interactions between bacteria and cells, and have often neglected to confirm the viability of mucosal epithelial cells after several hours or days in culture.
3.3.1 Norepinephrine and Dopamine, Their Receptors, and Bacterial Pathogen Invasion in the Small Intestinal Mucosa
In Peyer’s patches from the porcine small intestine, the invasion of enteropathogenic bacteria to the mucosa of the small intestine appears to be modulated by the enteric nervous system. Inhibition of neural conduction by the serosal side addition of the neuronal sodium channel blocker saxitoxin increases internalization of luminally-inoculated Salmonella enterica serovar Choleraesuis and EHEC by greater than sixfold in Peyer’s patch explants from the porcine jejunum. Internalization of a rodent commensal E. coli strain is unaffected by the toxin (Green et al. 2003) and that of S. enterica serovar Typhimurium is decreased by threefold (Brown and Price 2008).
The serosal application of 10 μM NE produced a six- to ninefold increase in luminal S. enterica serovar Choleraesuis and EHEC internalization in porcine Peyer’s patch explants. This effect was abolished in tissues pretreated with the alpha-AR antagonist phentolamine. Luminally-applied NE had no effect. Thus, this action of NE appears to be mediated by alpha-ARs that are likely localized to the basolateral aspect of Peyer’s patch epithelial cells (Green et al. 2003). In a study of S. enterica serovar Typhimurium internalization, the serosal application of DA or the sympathomimetic drugs cocaine and methamphetamine (which block the NE transporter, see above) decreased Salmonella recovery from Peyer’s patch explants (Brown and Price 2008). Although these neurally-mediated effects on Salmonella internalization may appear to be small, it should be emphasized that they were measured over a surface area of 2 cm2 in isolated tissues in a relatively short (90 min) time period. If extrapolated across the large surface area encompassed by the small intestine or even to the total area representing the Peyer’s patch epithelium, these changes in Salmonella uptake may be of considerable translational significance. At present, it is not known if these catecholamine effects occur in species other than swine, and studies of this phenomenon should be extended to other animal species and bacterial strains, including those represented in the gut microflora.
Electrical stimulation of enteric nerves in explants of non-Peyer’s patch mucosa from porcine jejunum more than doubled S. Typhimurium internalization, and neural conduction blockade decreased S. Typhimurium internalization by three- to fourfold. Pretreatment of explants with phentolamine also inhibited the electrically-induced increase in S. Typhimurium internalization (Schreiber et al. 2007).
3.3.2 Norepinephrine and Bacterial Adherence to the Cecal and Large Intestinal Mucosa
Norepinephrine and DA are equipotent in their ability to increase the number of EHEC adhering to the mucosal surface of cecal explants from mice. Their effects are prevented by AR and DAR antagonists respectively, suggesting that they are mediated by specific catecholamine receptors (Chen et al. 2003). The concentrations of NE applied to the basolateral aspect of the intestinal epithelium that are sufficient to promote EHEC adherence are lower than those necessary to promote epithelial EHEC adherence when incubated directly with the bacterium (Vlisidou et al. 2004; Bansal et al. 2007). Thus, the mechanisms underlying the mucosally-directed actions of catecholamines on EHEC adherence seem to differ from those responsible for the direct effects of NE on EHEC adherence (Freestone et al. 2007).
In mucosal explants of porcine cecum and colon, NE interacts with alpha 2-ARs to increase mucosal adherence of EHEC (Green et al. 2004). In contrast, increases in active anion secretion across the porcine colonic mucosa are mediated by alpha1-ARs (Traynor et al. 1991). Therefore, it appears that the actions of NE on ion transport and EHEC adherence are not linked through a common cellular mechanism. Alpha 2-ARs are negatively coupled to cyclic AMP production, which in turn leads to decreased intracellular protein kinase A activity. The adherence-promoting action of NE in the porcine colonic mucosa is inhibited by the protein kinase A activator Sp-cAMPS and mimicked by the protein kinase A inhibitor Rp-cAMPs (Green et al. 2004). From these data, it can be hypothesized that as yet unidentified downstream substrates in mucosal epithelial cells, which are subject to protein kinase A-induced phosphorylation, act to inhibit EHEC adherence. Accordingly, a decrease in kinase activity produced by NE would reduce this phosphorylation process and increase bacterial adherence. The effects of NE in the mouse and pig cecal mucosae are rapid (≤90 min) and experiments with EHEC eae and EspA deletion mutants suggest that NE and other sympathomimetic drugs enhance early, non-intimate bacterial adherence (Chen et al. 2003; Chen et al. 2006). This is further evidence supporting an epithelium-driven interaction rather than one mediated by bacterially-derived adherence factors.
Endogenous NE secreted from nerve terminals that are present in mucosal explants appears to modulate EHEC adherence as well. The monoamine oxidase inhibitor pargyline, which inhibits NE breakdown and inhibitors of NE reuptake into nerve terminals, including desipramine and cocaine, can mimic the actions of exogenous NE and the effects of these drugs are likewise inhibited by the alpha-AR antagonist phentolamine (Green et al. 2004; Chen et al. 2006). Catecholamines and in particular NE may play a physiological role in promoting bacterial colonization of the large intestine, perhaps as an element of competitive exclusion. Norepinephrine increased cecal adherence of a non-O157 commensal strain of E. coli, which was isolated from the porcine colonic mucosa (Chen et al. 2006). One interpretation of this result is that the action of NE, which is mediated by alpha-ARs in the intestinal mucosa, is not limited to a particular bacterial strain or species. Clearly, this is an area requiring further investigation.
3.4 Potential Host Mechanisms That May Underlie Catecholamine-Associated Mucosal Interactions with Bacteria
3.4.1 Catecholamines and Mucosal Ion and Water Transport
Catecholamines, and in particular NE, are poised to modulate mucosal associations with bacteria, however the mechanisms underlying catecholamine actions remain to be further defined. As indicated above, the actions of catecholamines on epithelial active ion transport are well known. Norepinephrine alters active, transepithelial ion transport in the intestinal mucosa through interactions with functionally-defined alpha-ARs and to a lesser extent, beta-ARs. Its action is mediated indirectly through enteric nerves or by direct effects on epithelial cells depending upon the animal species and intestinal segment examined (Brown and O’Grady 1997; Horger et al. 1998). Dopamine alters active ion transport through direct and indirect actions on enteric ARs and DARs (Donowitz et al. 1982, 1983; Vieira-Coelho and Soares-da-Silva 1998; Al-Jahmany et al. 2004; Zhang et al. 2007, 2008, 2010; Feng et al. 2013). The delivery of mucins and SIgA, which are secreted in the intestinal crypts, to the mucosal surface would be affected by changes in water movements across the mucosa that accompany the active transepithelial fluxes of electrolytes (Hecht 1999). As discussed below, both types of molecules contribute to the mucosal “glycobiome” (Ouwerkerk et al. 2013), which protects the mucosal surface and regulates the composition and spatial characteristics of the overlying microbial community. Moreover, in the small intestine, the movement of these proteins in the crypt to villous direction promotes sterility in the crypts, an area possessing limited barrier function and harboring a critical stem cell population involved in epithelial renewal (Johansson et al. 2013).
3.4.2 Catecholamines and Mucus Secretion
The layer of mucus coating the intestinal mucosal surface protects vulnerable epithelial cells from microbial infection, has important roles in the support of commensal microbial communities in the large intestine and, in association with active anion secretion and intestinal motility, facilitates the transit of microbes from the small intestine to the colon (Johansson et al. 2013). The principal structural components of mucus are gel-forming mucin glycoproteins, which are secreted by intestinal goblet cells (Johansson and Hansson 2013). Glycosylated molecules important for mucosal protection such as SIgA (see below) bind to carbohydrate domains in mucin and are retained in mucus layers (Meyer-Hoffert et al. 2008; Antoni et al. 2013).
In addition to secreted mucins, transmembrane mucins are expressed by enterocytes, where they contribute to the formation of an apical glycocalyx, which acts as an epithelial binding site for bacteria (Patsos and Corfield 2009). Furthermore, this class of membrane-tethered mucins may participate in cell to cell interactions and can be coupled to intracellular signaling pathways (Jonckheere et al. 2013).
Epinephrine and NE have long been known to regulate the secretion of gel-forming mucins in the airways of several animal species (but not humans) (Rogers 2002) and act through beta-ARs, with a smaller contribution from alpha-ARs, to stimulate mucin secretion from salivary glands (Quissell and Barzen 1980; Dohi et al. 1991; Taylor and McWhorter 1991). It is generally appreciated that enteric neurotransmitter substances can influence the secretion of gel-forming mucins from intestinal goblet cells (Neutra et al. 1984). However, surprisingly little is known about the regulation of this process by catecholamines. Beta-AR agonists have been shown to increase mucin secretion in the murine colonic mucosa (Smith and Butler 1974), but they have no effect on mucin output in rat small intestine (Mantle et al. 1991). Moreover short-term stress, which is generally associated with increased sympathetic outflow to the digestive tract, decreases mucin production in the rat colon. However, this effect remains unaltered in adrenalectomized rats or animals pre-treated with bretylium, which blocks NE release from sympathetic nerve terminals (Pfeiffer et al. 2001).
Some species of resident bacteria in the intestine express adhesins that recognize and bind to glycan residues on mucins (Etzold and Juge 2014), and some enteropathogens have developed virulence mechanisms that allow them to circumvent the mucus barrier (Johansson et al. 2013). Catecholamines could promote epithelial invasion by Salmonella or other entero-invasive bacteria or enhance adherence of entero-adherent pathogens like EHEC by directly altering the secretion of gel-forming mucins and the thickness of the mucus covering epithelial cells. Because ARs and DARs are coupled to intracellular changes in cyclic AMP, free calcium, arrestin and other downstream signaling pathways, it is conceivable that catecholamines could alter aspects of mucin gene expression, biosynthesis or turnover (Theodoropoulos and Carraway 2007). They could also affect mucus dynamics by altering transepithelial ion and fluid transport, which would affect the hydration state and protective capabilities of intestinal mucus. Indeed, catecholamines activate cystic fibrosis transmembrane regulator (CFTR) anion channels and CFTR-dependent bicarbonate transport is essential for proper mucous discharge (Joo et al. 2001; Ambort et al. 2012; Yang et al. 2013). A role of transmembrane mucins in the interactions between intestinal epithelial cells and bacteria has not been defined. Catecholamines may rapidly up-regulate particular classes of membrane-tethered mucins, which could serve as bacterial docking sites on epithelial cells. These possibilities await investigation.
In the Ussing chamber paradigm, the tangential flow of bathing media could physically disrupt mucus overlying the epithelium, thereby exposing epithelial cells to increased bacterial contact. This may occur particularly with small intestine explants that, in contrast to the large intestine, do not possess a firmly attached inner mucus layer at the mucosal surface that is relatively resistant to mechanical disruption (Johansson et al. 2013). In IVOC systems, on the other hand, mucosal specimens are reportedly covered with a thick coating of mucus, which can restrict early bacterial contact with epithelial cells (Haque et al. 2004). Because they lack goblet cells, cell culture systems including three-dimensional organotypic models do not possess visco-elastic mucus that would serve as a complicating variable in the interpretation of experiments involving epithelial-bacterial interactions (Barrila et al. 2010). Of course, due to their reduced cellular complexity, these preparations have their own limitations when one considers the several potential sites of catecholamine action in the intestinal mucosa.
3.4.3 Catecholamines and Secretory Immunoglobulin A (SIgA)
Immunoglobulin A in a dimeric form is synthesized in and secreted from plasma B lymphocytes residing in the lamina propria underlying the intestinal mucosa. The joining (J) peptide chain between the two IgA molecules serves as a ligand for the polymeric immunoglobulin receptor (pIgR), which is situated on the basolateral membrane of crypt epithelial cells and serves to transport dimeric IgA through epithelial cells and into the intestinal crypts. Dimeric IgA that has undergone transcytosis is proteolytically cleaved from pIgR, but retains a portion of the receptor that is known as secretory component. SIgA therefore consists of dimeric IgA complexed with secretory component (Brandtzaeg et al. 2008). Once delivered by ion and water fluxes to the mucosal surface, these molecules can bind to and distribute within the intestinal mucus and bind as well to bacteria because of their highly-glycosylated chemical structures. SIgA and free secretory component play important roles in mucosal protection by reducing contact of microbial pathogens with mucosal epithelial cells; decreasing mucosal inflammation, which could compromise epithelial barrier function; and regulating the gut microbiota (Corthésy 2013). They have also been found to bind to the apical glycocalyx on M cells in Peyer’s patch follicles, which serve as sites for contact between luminal bacteria and the follicle-associated epithelium (Kato 1990).
Within the digestive tract, it is well established that catecholaminergic nerves act on salivary glands to regulate SIgA secretion in saliva (Proctor and Carpenter 2007). In the intestine, my collaborators and I have reported that adrenergic nerve fibers immunoreactive for dopamine beta-hydroxylase are present throughout the submucosa and terminate near the basal membranes of crypt and villous/surface epithelial cells of the porcine ileum, distal colon and cecum (Schmidt et al. 2007; Green et al. 2004; Chen et al. 2006). In the porcine small and large bowel mucosa, dopamine beta-hydroxylase-immunoreactive nerves terminate near IgA-positive B lymphocytes and neighboring epithelial cells that display immunoreactivity for pIgR (large intestine: Schmidt et al. 2007; small intestine, Fig. 3.1: L.D. Schmidt, L. Vulchanova and D.R. Brown, unpublished observations). Norepinephrine stimulates the vectorial secretion of secretory IgA in mucosa explants from the porcine ileum (Fig. 3.2) and colon (Schmidt et al. 2007). This effect has been attributed to an alpha-AR-mediated increase in the epithelial turnover of pIgR resulting in the vectorial transport of SIgA as well as free secretory component towards the mucosal surface (Schmidt et al. 2007; L.D. Schmidt, Y. Xie, L. Vulchanova and D.R. Brown, unpublished observations).
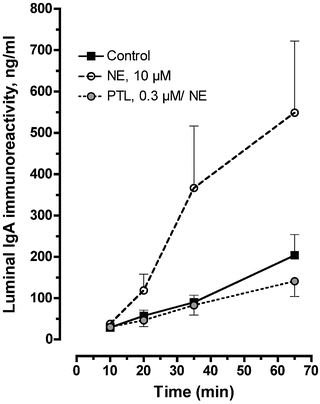
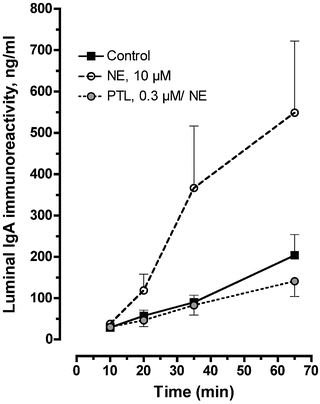
Fig. 3.2
Effect of norepinephrine (NE) on luminal IgA secretion from explants of porcine ileal mucosa. Tissues were treated with NE (10 μM) in the absence or presence of the alpha-adrenergic antagonist phentolamine (PTL) at a concentration of 0.3 μM in the contraluminal bathing medium. Tissues untreated with drugs served as controls in each experiment. NE was added to the contraluminal bathing medium 5 min after the start of each experiment (i.e. time = 5 min), and phentolamine was added immediately after collection of a luminal fluid sample at time = 0 min. Mean IgA immunoreactivity at 0 min was 92 ± 27, 80 ± 29 and 42 ± 10 ng/mL from control, NE-treated and PTL-/NE-treated tissues (n = one tissue from each of 5–7 pigs), respectively. The line elevation for NE alone was significantly different from control (F = 17.7, P = 0.02) as determined by linear regression analysis
In the rat small intestine, NE enhances the expression of pIgR mRNA (Reyna-Garfias et al. 2010). Restraint stress in rats increases IgA content in the lamina propria of the small intestine, an effect inhibited by chemical sympathectomy with 6-hydroxydopamine (Reyna-Garfias et al. 2010). Sympathectomy alone has been reported to reduce gut IgA responses to oral antigen exposure in adult rats (González-Ariki and Husband 1998) and decrease the number of IgA-positive lamina propria cells in the weanling rat (González-Ariki and Husband 2000).
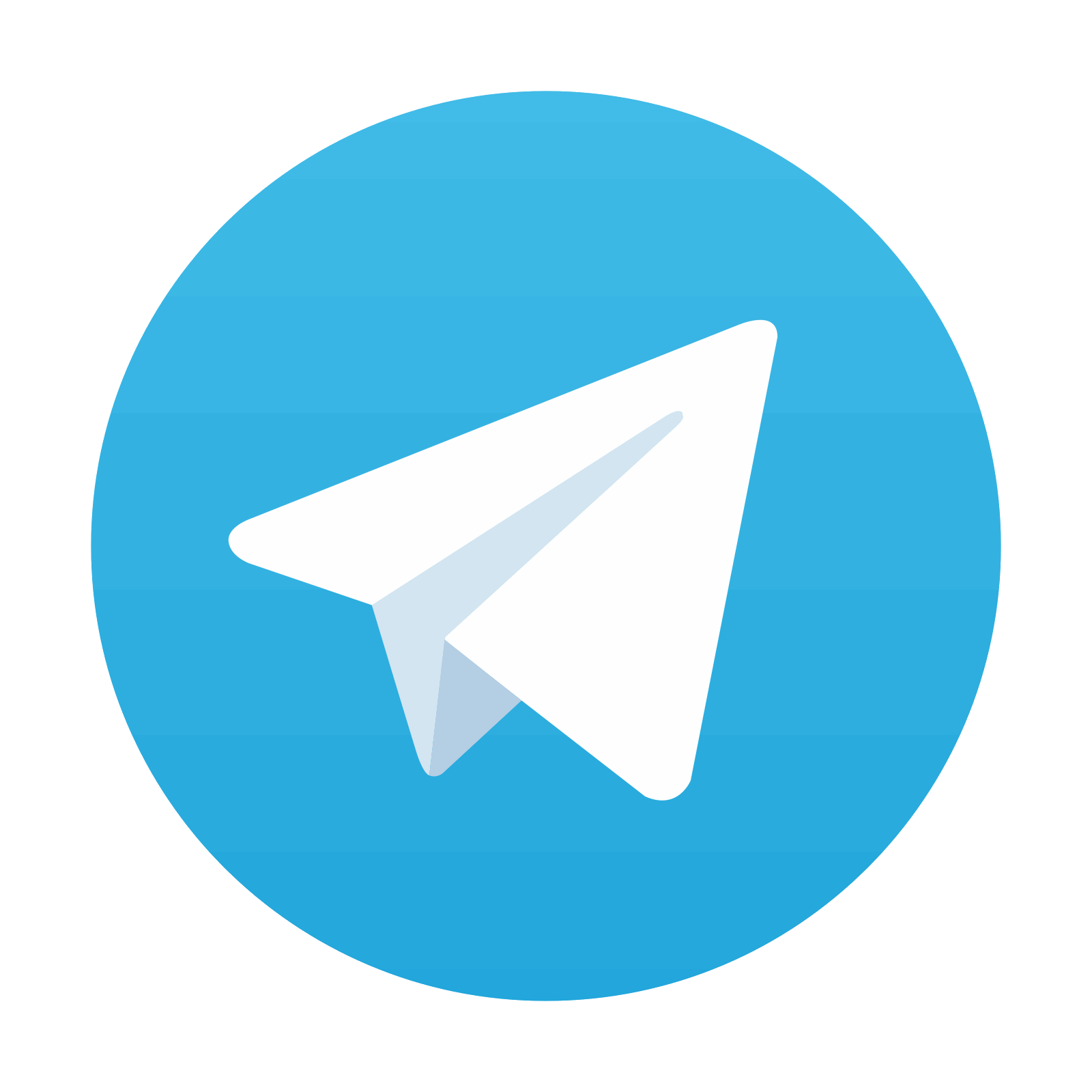
Stay updated, free articles. Join our Telegram channel

Full access? Get Clinical Tree
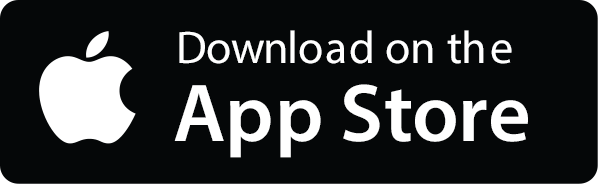
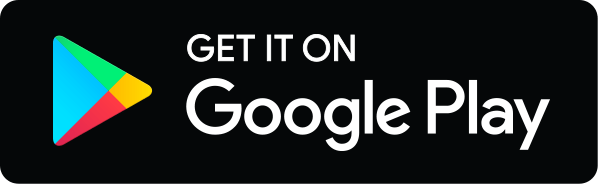