(1)
Children’s Heart Center, The Children’s Hospital at Montefiore/Albert Einstein College of Medicine, 3415 Bainbridge Avenue, R-1, Bronx, NY 10467, USA
(2)
University of Kansas Medical Center, 3901 Rainbow Boulevard, Kansas City, KS 66160, USA
(3)
Division of Pediatric Cardiology, New York-Presbyterian/Morgan Stanley Children’s Hospital, 3959 Broadway #517;, New York, NY 10032, USA
(4)
Departments of Internal Medicine and Pediatrics, University of Miami Miller School of Medicine, 1611 NW 12th Avenue C600D, Miami, FL 33136, USA
(5)
Medicine (Cardiology), Oncology, Obstetrics and Gynecology, Molecular Biology and Genetics, Family Medicine and Public Health Sciences, and Pharmacology, Wayne State University School of Medicine, Detroit, MI, USA
(6)
Center for Urban Responses to Environmental Stressors, Wayne State University School of Medicine, Detroit, MI, USA
(7)
Carman and Ann Adams Department of Pediatrics, Children’s Research Center of Michigan, Children’s Hospital of Michigan, Wayne State University School of Medicine, Detroit, MI, USA
(8)
NCI-designated Comprehensive Cancer Center, Karmanos Cancer Institute, 3901 Beaubien Boulevard, 1K40, Detroit, MI 48201-2196, USA
10.1 Pathophysiology
10.2.1 Chemotherapy
10.2.1.1 Anthracyclines
10.2.1.2 Alkylating Agents
10.2.1.3 Tyrosine Kinase Inhibitors
10.2.1.4 Other
10.2.2 Radiation
10.3.1.1 Drug-Related Risk Factors
10.3.1.2 Drug Delivery
10.3.1.3 Liposomal Anthracyclines
10.3.1.4 Novel Anthracycline Analogues
10.3.2.1 Dose Reduction
10.3.2.2 Targeted Therapy
10.4.1 Cardiac Considerations
10.4.1.4 Cardiometabolic Screening
10.4.1.5 Exercise Stress Testing
10.4.1.6 Post-radiation
10.4.2 Noncardiac Considerations
10.4.2.1 Patient-Related Risk Factors
10.4.2.2 Obesity
10.4.2.3 Diabetes Mellitus
10.4.2.4 Hypertension
10.5.1 Pharmacologic Management
10.5.2 Cardioprotective Agents
10.5.3 Nutritional Supplements
10.5.4 Non-pharmacologic Management
10.5.5 Continuous Infusion
10.6 Conclusions
During the past 40 years, advances in cancer treatment have drastically improved survival rates for children with cancer. Of the 325,000 childhood cancer survivors in the United States, 24 % have survived greater than 30 years from diagnosis [1]. This growing population of survivors brings the wide array of potential late effects of cancer therapy to light [2]. The developing cardiovascular system is particularly vulnerable to cardiotoxic cancer therapies such as anthracyclines and radiation. These treatment modalities have been found to result in chronic, progressive cardiac dysfunction occurring months to decades after treatment [3–6].
While contemporary treatment protocols seek to balance oncologic efficacy with cardiotoxic risk, via cardioprotective agents, lower cumulative doses of anthracyclines, and reduced dose, field and volume of radiation, long-term survivors of childhood cancer continue to experience the consequence of earlier treatment approaches. For example, a 5-year-old girl treated in the 1970s for acute lymphoblastic leukemia (ALL) with multimodality therapy including 465 mg/m2 cumulative dose of doxorubicin and cranial radiation developed congestive heart failure (CHF) after 34 months of complete remission, and dilated cardiomyopathy at the age of 16. Bouts of CHF were treated with beta-blockers, diuretics, digoxin, angiotensin-converting enzyme (ACE) inhibitors, an implantable cardiac defibrillator, and ultimately a cardiac transplant at age 36. Unfortunately, she discontinued her medications 6 months posttransplant and suffered cardiac death [7]. In this patient and others, the late-occurring effects of childhood cancer treatment include diastolic and systolic dysfunction, conduction abnormalities, cardiomyocyte necrosis, heart failure, heart transplantation, medication noncompliance, depression, and suicide. In addition, even subclinical, treatment-induced changes could predispose to or exacerbate premature onset of hypertension [8, 9], diabetes mellitus [10], obesity [11], and cardiovascular disease (CVD) [3, 12–17].
Early detection and prevention are crucial to reduce the cardiovascular damage of modern treatment modalities. Although the late cytotoxic effects of cancer therapy may not be completely avoidable, continued development of pediatric protocols is crucial to reducing negative treatment outcomes.
10.1 Pathophysiology
The heart begins developing at week 3 of gestation and completes primary morphogenesis at the end of week 8. Early in this time period, myocardial cell replication is high and then tapers off as the septum develops [18]. Cell size remains constant with most embryonic growth occurring through hyperplasia instead of hypertrophy [19]. Late prenatal and early postnatal increases in cardiac mass are marked by both hyperplasia and hypertrophy [20]. By 6 months of age, with the adult number of cardiomyocytes nearly present, subsequent increases in cardiac mass occur via hypertrophy. In order to maintain healthy cardiac output after cardiomyocyte necrosis, the surviving cardiomyocytes undergo compensatory hypertrophy [21]. Hypertrophy of the cardiomyocytes is often observed along with easily determined echocardiographic parameters such as reduced wall thickness and interstitial fibrosis [21]. Therefore, late cardiac failure could be a result of the surviving cardiomyocytes’ inability to meet the cardiac demands of a growing body.
The pumping action of the heart is accomplished by the synchronized contraction of cardiomyocytes. An action potential is initiated at the sinus node located in the right atrium. The action potential then travels to the atrioventricular node and His-Purkinje system and ultimately onto the cardiomyocytes in the ventricles. In order for the heart to pump optimally, multiple factors must integrate properly. The actin and myosin filaments in the cardiomyocyte must be in their relaxed state when the action potential arrives. This allows for optimal cross bridging and contractility. Also, there must be calcium in the sarcoplasmic reticulum available to catalyze the cross bridging. Finally, the cardiomyocytes must return to their relaxed state before the next action potential arrives. Anthracyclines and radiation are known to impede the myofibrils from returning to their resting state.
10.2 Cytotoxic Effects of Therapy
10.2.1 Chemotherapy
10.2.1.1 Anthracyclines
Anthracycline-induced acute cardiac damage is characterized by swelling of the sarcoplasmic reticulum, distortion of myofibrils, and necrosis of cardiomyocytes [22]. The exact mechanism involved with myocardial toxicity from anthracyclines has yet to be completely established. However, studies suggest that highly reactive oxygen species (ROS) play a role in damaging cardiomyocytes [23]. Decreasing numbers of functional cardiomyocytes are associated with diastolic and systolic dysfunction found later in the survivors’ lives [24].
The antineoplastic effect of anthracyclines depends on several mechanisms involving inhibition of topoisomerase II, high binding affinity to DNA thus blocking synthesis, altered fluidity and ion channels of cellular membranes, the generation of free ROS, and the disruption of mitochondria [25]. Anthracyclines form complexes with intercellular iron and generate ROS, such as hydrogen peroxide, which readily cause lipid peroxidation and subsequently cellular membrane damage [25, 26]. Due to low levels of catalase, cardiomyocytes are especially vulnerable to ROS. Also, cardiolipin found in the inner membrane of cardiac mitochondria has a high affinity for anthracyclines, which allows higher concentrations of anthracycline in cardiomyocytes [27, 28]. Anthracyclines have also been found to suppress antioxidant enzymes, such as myocardial glutathione oxidase, making cardiomyocytes even more susceptible to damage. Increased oxidant stress results in cardiomyocyte apoptosis via mitogen-activated protein kinase pathways [26]. Changes independent of oxidative stress include impaired apoptotic signaling [29], cardiac stem cell depletion [30], altered gene expression [31], and inhibited release of calcium in the sarcoplasmic reticulum [32].
Alteration of mitochondrial structure and function induced by anthracyclines also affects contractility. Mitochondrial membrane permeability alterations cause intra- and extramitochondrial calcium ion concentrations to change [33]. Additionally, anthracyclines decrease the concentration of subunits in the respiratory chain [34–36]. These abnormalities of the respiratory chain could produce more ROS, further damaging the mitochondria ultimately resulting in cardiomyocyte death [36].
10.2.1.2 Alkylating Agents
Alkylating drugs such as cyclophosphamide, cisplatin, and ifosfamide have been associated with cardiotoxicity (Table 10.1). Clinical manifestations include intramyocardial edema in conjunction with serosanguinous pericardial effusion [37] and are associated with left ventricular (LV) wall thickness and mass increases.
Table 10.1
Potential cardiotoxicity of cancer therapy
Drug | Toxic dose range | Acute toxicity | Chronic toxicity |
---|---|---|---|
Doxorubicin (Adriamycin RDF, Doxil) | >550 mg/m2 (total dose) | Arrhythmias, pericarditis-myocarditis syndrome, myocardial infarction, sudden cardiac death | Cardiomyopathy/CHF, conduction abnormalities/arrhythmias |
Mitoxantrone (Novantrone) | >100–140 mg/m2 (total dose) | CHF, decreases in left ventricular ejection fraction, myocardial infarction, ECG changes, arrhythmias | |
Cyclophosphamide (Cytoxan) | >100–120 mg/kg (over 2 days) | Hemorrhagic cardiac necrosis, reversible systolic dysfunction, ECG changes, CHF | |
Ifosfamide (Ifex) | ECG changes, CHF, arrhythmias | ||
Cisplatin (Platinol) | Conventional dose | Myocardial ischemia, Raynaud’s phenomenon, ECG changes | |
Fluorouracil (Adrucil, Efudex) | Conventional dose | Myocardial infarction, angina, cardiogenic shock/sudden death, dilated cardiomyopathy | |
Trastuzumab (Herceptin) | Ventricular dysfunction, CHF | Cardiomyopathy | |
Paclitaxel (Taxol) | Conventional dose | Sudden death, bradyarrhythmia, myocardial dysfunction, myocardial infarction | |
Amsacrine (Amsa-PD) | Conventional dose | Ventricular arrhythmia, ECG changes | Cardiomyopathy |
Cytarabine (Cytosar-U) | CHF, pericarditis, arrhythmias | ||
Arsenic trioxide (Trisenox) | Arrhythmias, pericardial effusion | ||
Interferon alpha-2A (Roferon) | Conventional dose | Exacerbates underlying cardiac disease, hypotension, arrhythmias | Cardiomyopathy |
Interleukin-2 (Aldesleukin) | Conventional dose | Myocardial injury/myopericarditis, ventricular arrhythmias, hypotension, sudden death | Dilated cardiomyopathy |
Mitomycin (Mutamycin) | Conventional dose | CHF | Congestive heart failure |
Vincristine (Oncovin) | Conventional dose | Myocardial infarction, hypotension, cardiovascular autonomic neuropathy | |
Vinblastine (Velban) | Conventional dose | Myocardial infarction, Raynaud’s phenomenon | |
Busulfan (Busulfex) | Conventional oral daily dose | Endocardial fibrosis, pulmonary fibrosis, pulmonary hypertension, cardiac tamponade | |
Tyrosine kinase inhibitor (Imatinib) | Conventional dose | CHF |
The proposed mechanism is direct vascular endothelial injury followed by the leaking of toxic metabolites, interstitial hemorrhage, and edema [38]. Cyclophosphamide may also initiate ischemic cardiomyocyte damage through the formation of intracapillary microemboli [39]. Cyclophosphamide can accentuate cardiotoxicity induced by anthracyclines due in part to an associated myopericarditis [40]. Sequential dosing may also cause the cardiomyocytes to become more sensitive to cyclophosphamide [40]. Ifosfamide is another potentially cardiotoxic alkylating agent with a similar structure to cyclophosphamide. High doses of ifosfamide are associated with cardiac dysfunction analogous to that of cyclophosphamide [41]; cardiac damage resulting in CHF and arrhythmias has been reported in adults [42].
10.2.1.3 Tyrosine Kinase Inhibitors
Tyrosine kinase inhibitors (TKI), such as imatinib, interfere with targeted pathways in neoplasms on an intracellular level [43]. However, TKIs also inhibit kinase enzymes in cardiac tissue. Cardiac biopsies of mice treated with imatinib revealed abnormalities of mitochondria, vacuoles, and the endoplasmic reticulum [44]. TKIs are a drug class only recently implemented as part of pediatric protocols. One small retrospective strategy found that of 36 pediatric leukemia patients exposed to imatinib, only 2 children developed cardiomyopathy (these 2 patients were also exposed to anthracyclines, cyclophosphamide, or radiation) [45]. Further studies are needed to determine what screening tools should be put in place before and after receiving TKI therapy.
10.2.2 Radiation
Exposure to radiation can have many effects on the heart including, but not limited to, valvular injury, conduction and autonomic defects, CAD, pericarditis, myocarditis, and cardiomyopathy [49]. The extent of damage to the heart is dependent on the techniques used, the radiation dose administered (including the size of the daily fractions), and the volume of the heart that is irradiated, in addition to the concomitant use of cardiotoxic agents such as anthracyclines.
Microcirculatory damage to the myocardium is characterized by diffuse, nonspecific interstitial fibrosis. Lesions are often localized and can be millimeters to several centimeters in size. Fibrosis can differ from one area of the myocardium to the next [50, 51]. The proportion of type I collagen increases compared to type III collagen leading to altered compliance of the myocardium and ultimately diastolic dysfunction [52]. Myocardial cells involved with conduction are also damaged by radiation [53–55]. Injury to the capillary endothelial cells leads to obstructed capillary lumens, which engenders thrombus formation.
Inflammatory pathways are thought to be important in the pathogenesis of atherosclerosis, ischemic stroke, and myocardial infarction [56]. Exposure to radiation can activate the pathways leading to inflammation [57]; chronic inflammation increases the risk of developing atherosclerosis and can impair the vasculature of the heart which damages the cardiomyocytes and leads to fibrosis [58]. Radiation can also lead to the production of ROS, which cause oxidative damage to cardiomyocytes [59].
Historically, thickening of the pericardium was a significant risk for patients exposed to radiotherapy [60]. Pericardial adipose tissue was replaced with collagen and fibrin. Similar to the myocardium, small blood vessels with increased permeability propagate in the pericardium. Ischemia and fibrosis occurs due to the damage of the vascular system. Dysfunction of the lymphatic and venous systems of the heart then leads to buildup of extracellular fluids [50]. Thickening of the pericardium has become less prevalent with modern day techniques.
Radiation may cause fibrosis in valves with or without calcification [60, 61]. The pathogenesis of valvular damage is not completely understood. The valves of the heart are avascular, and therefore the mechanism cannot be attributed to microvascular damage. Studies have found that the prevalence and severity of damage are higher in valves on the left side of the heart than valves found on the right [61, 62].
Even with few risk factors other than radiotherapy, many patients develop subsequent CAD. The left anterior descending and right coronary arteries are the most commonly affected vessels. However, with a mean radiation dose of 42 ± 7 Gy, McEniery et al. demonstrated 8 out of 15 patients developed at least a 50 % stenosis of the left main coronary artery [63]. This could be a result of the older technique of anterior-weighted irradiation (due to lower energies that necessitated high superficial doses in order to deposit sufficient dose at depth). Proximal narrowing of the vessel occurs and frequently includes the coronary ostia [60, 61, 63–65]. The exact mechanism of radiation-associated CAD is unknown and, histologically, may be similar to atherosclerotic-induced CAD. However, radiotherapy-associated CAD appears to have increased media and adventitial destruction as well as more loss of smooth muscle cells [66].
10.3 Clinical Manifestations
10.3.1 Anthracycline-Induced Cardiomyopathy
Anthracyclines are one of the most well-known classes of chemotherapeutic agents associated with cardiovascular toxicity. Therapy involving anthracyclines has three categories of cardiotoxicity identified by the time signs or symptoms become apparent and how quickly CHF symptoms worsen. The categories include acute, early onset, and late onset (Table 10.2). Late-onset cardiotoxicity occurs any time after the first year following treatment and is the focus of this chapter.
Table 10.2
Characteristics of different types of anthracycline cardiotoxicity
Characteristic | Acute cardiotoxicity | Early-onset chronic progressive cardiotoxicity | Late-onset chronic progressive cardiotoxicity |
---|---|---|---|
Onset | Within the first week of anthracycline treatment | <1 year after the completion of anthracycline treatment | ≥1 year after the completion of anthracycline treatment |
Risk factor dependence | Unknown | Yes | Yes |
Clinical features in adults | Transient depression of myocardial contractility | Dilated cardiomyopathy | Dilated cardiomyopathy |
Clinical features in children | Transient depression of myocardial contractility; myocardial necrosis (cTnT elevation); arrhythmia | Restrictive cardiomyopathy and/or dilated cardiomyopathy; arrhythmia | Restrictive cardiomyopathy and/or dilated cardiomyopathy; arrhythmia |
Course | Usually reversible on discontinuation of anthracycline | Can be progressive | Can be progressive |
Chronic CHF, decreased exercise therapy, and LV dysfunction are all potential indicators of these three cardiotoxicity categories [67]. Signs of acute cardiotoxicity can span from small abnormalities in electrocardiographic or echocardiographic readings to severe CHF. QTc interval prolongation has been noted in the acute phase following doxorubicin administration, but no adverse sequelae are attributed to this [68]. Acute changes are generally transient and are indicated by systolic dysfunction of the LV observed by echocardiography in less than 1 % of patients [16, 69, 70]. Although most patients recover from acute changes, those who received a high cumulative dose may experience permanent damage. Children who experience LV dysfunction during or immediately after anthracycline therapy are at the highest risk for chronic, progressive cardiac abnormalities [3].
Early-onset cardiotoxicity is characterized by LV dysfunction, electrophysiologic changes, decreased exercise capacity, and clinical CHF [16, 67, 69–74]. Late-onset LV dysfunction is more common among survivors of childhood cancer [16, 75]. Late LV dysfunction has been found to occur as far as two decades following completion of treatment. This type of cardiac deterioration most likely stems from damage induced by treatment that was not severe enough to cause observable symptoms during therapy. Progressive LV dilation, LV wall thinning, and decreased LV contractility develop from cardiomyocyte death and/or damage [76]. In order to maintain cardiac output and LV systolic function, the LV dilates, leading to thinning of the ventricular walls. These changes in structure place more stress on the walls of the LV, further compromising its systolic performance. Eventually, dilation of the LV is unable to meet the additional stress and metabolic demands placed on the heart, leading to CHF. Additional stressors on the heart that can exacerbate underlying dysfunction include acute viral infection [77], growth hormone therapy [78], pregnancy, and physical strain such as lifting weights [77, 79, 80].
Abnormalities have been found to increase over time and are often monitored in echocardiographic measurements, such as LV fractional shortening (LVFS), LV end diastolic dimension, LV afterload, LV contractility, and LV diastolic filling phase indices including strain [21, 80, 81]. Electrocardiograms (ECG) and exercise stress tests can also detect cardiac abnormalities [21]. Lipshultz et al. found that a majority of survivors of ALL had abnormal LV afterload indicative of reduced LV contractility after a median of 6 years of follow-up [21]. The findings are suggestive of progressive rather than static cardiotoxicity because 24 of 34 patients had increased LV afterload values on their last study when compared to earlier measurements.
10.3.1.1 Drug-Related Risk Factors
Higher cumulative dose of anthracyclines is a well-known risk factor for LV dysfunction (Table 10.3) [21, 81]. In a study of 6,493 patients who received varying cumulative doses of anthracyclines, the prevalence of CHF was 0.15 % with doses of 400 mg/m2 or less, while it was 7 % among those who had cumulative doses of 550 mg/m2 or more [82]. Also, those who received the higher cumulative dose of anthracycline had a relative risk greater than five of clinically apparent cardiotoxicity [83]. In 1991, Lipshultz et al. found that high cumulative dose of anthracycline was the strongest predictor of cardiotoxicity in a cohort of 115 ALL survivors who received doxorubicin [21]. Another cohort was constructed by Steinherz et al. who demonstrated decreased cardiac function using echocardiography over time in patients who had received anthracyclines 4–20 years prior [80]. Similarly, data from the National Wilms’ Tumor Study Group found that cumulative dose of doxorubicin increased the patient’s relative risk of CHF by a factor of 3.3 for every 100 mg/m2 of doxorubicin [84]. Though increases in dose of doxorubicin lead to increased cardiotoxicity, the relationship is not linear (Fig. 10.1). Notably, LV dysfunction is still reported in patients with total dose less than 300 mg/m2 [85, 86]. As alluded to above, the dose of a cardiotoxic agent is only one determinant in overall cardiac risk assessment, and no dose of anthracycline is absolutely safe [87].
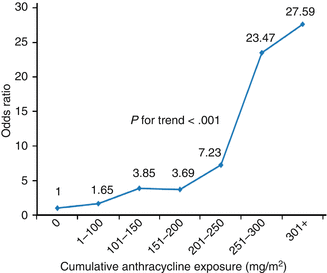
Table 10.3
Risk factors for cardiotoxicity
Risk factor | Comment | References |
---|---|---|
Cumulative anthracycline dose | Cumulative doses >500 mg/m2 are associated with significantly elevated long-term risk | |
Time after therapy | Incidence of clinically important cardiotoxicity increases progressively after therapy | |
Rate of anthracycline administration | Prolonged administration to minimize circulating dose may decrease toxicity; results are mixed | |
Individual anthracycline dose | Higher individual doses are associated with increased late cardiotoxicity, even when cumulative doses are limited; no dose is risk-free | |
Type of anthracycline | Liposomal encapsulated preparations may reduce cardiotoxicity. Data on anthracycline analogues and differences in cardiotoxicity are conflicting | |
Radiation therapy | Cumulative radiation dose >30 Gy; as little as 5 Gy increases the risk; before, after, or concomitant with anthracycline treatment | |
Concomitant therapy | Trastuzumab, cyclophosphamide, bleomycin, vincristine, amsacrine, and mitoxantrone, among others, may increase susceptibility or toxicity | |
Preexisting cardiac risk factors | Hypertension; ischemic, myocardial, and valvular heart disease; prior cardiotoxic treatment | [71] |
Personal health habits | Smoking, alcohol consumption, energy drinks, stimulants, prescription and illicit drugs | [90] |
Comorbidities | Diabetes, obesity, renal dysfunction, pulmonary disease, endocrinopathies, electrolyte and metabolic abnormalities, sepsis, infection, pregnancy, viruses, elite athletic participation, low vitamin D levels | |
Age | Both young and advanced age at treatment are associated with elevated risk | |
Sex | Females are at greater risk than males | [81] |
Complementary therapies | More information needs to be collected to assess risk | [90] |
Additional factors | Trisomy 21; African-American ancestry | [70] |
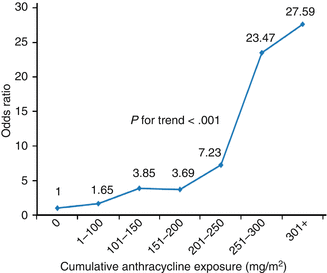
Fig. 10.1
Dose-response relationship between cumulative anthracycline exposure and risk of cardiomyopathy. Patients with no exposure to anthracyclines served as the reference group. Magnitude of risk is expressed as odds ratio, which was obtained using conditional logistic regression adjusting for age at diagnosis, sex, and chest radiation (Reprinted with permission from the publisher Blanco et al. [247]
Echocardiographic measures of cardiac strain were measured in a cohort of pediatric oncology patients treated with a single dose of anthracycline as compared to another that received at least 228 mg/m2 or more [21]. Children who received a single dose had less impaired LV function than those who received more. In this population, LVFS and LV contractility were significantly reduced, reflecting cardiac dysfunction. Left ventricular afterload, which is a direct function of blood pressure, LV dimension, and inversely related to LV wall thickness, was greatly elevated in those who received more anthracycline. This was attributed to a reduced LV wall thickness. LV contractility was also decreased.
10.3.1.2 Drug Delivery
Rate of administration has been suggested as another possible segregating factor in determining anthracycline toxicity (Table 10.3). Some studies found that children who received higher anthracycline dose rates experienced cardiotoxicity independent of cumulative dose [88]. Additionally, in adult protocols, continuous infusion of anthracyclines was associated with reduced cardiotoxicity [89]. Despite the lack of evidence on efficacy in the pediatric population, many pediatric protocols incorporated continuous infusion based on adult study findings [90–92]. However, evidence to date does not support a cardioprotective difference between continuous infusions as compared to bolus doses in children. While it was thought that prolonged exposure might reduce the peak dose and help prevent anthracycline-induced cardiomyopathy, this appears not to be so according to echocardiographic measures published in 2002 [91]. Left ventricular structure and function were not significantly different between the two groups who received doxorubicin either as a bolus or as a continuous infusion. Multiple echocardiographic measurements were used in this study to evaluate LV function, and in both cohorts equivalent levels of LV dysfunction were observed. Continued follow-up of this cohort had similar findings after 8 years [92]. Subclinical cardiotoxicity after moderate doses of anthracycline was not mitigated by 6-hour infusions in another study [93], which also suggests that rate of infusion is not an important parameter in determining risk.
10.3.1.3 Liposomal Anthracyclines
Clinical trials with doxorubicin began in the 1960s but not until the 1990s was its liposomal form used [94]. By encapsulating doxorubicin within a liposome, the volume of distribution is decreased, thus decreasing toxicity while preserving efficacy (Table 10.3) [95]. Liposomes also appear to localize their encapsulated drug directly at tumor sites by having an affinity for leaky tumor vasculature [95].
No difference in efficacy was noted in patients with metastatic breast cancer when comparing pegylated liposomal to conventional doxorubicin, but the incidence of cardiotoxicity decreased from 19 to 4 % (p < 0.001) [96]. The process of pegylating involves attaching polyethylene glycol to the molecule to further decrease immunogenicity and clearance while enhancing therapeutic effects. Additionally, two studies provided evidence that pegylated doxorubicin appears to accumulate in myocardial cells in a reduced amount when compared to doxorubicin [97, 98]. Further evidence of decreased cardiotoxicity is provided when comparing serial left ventricular ejection fraction (LVEF) measurements as cardiomyopathy is decreased at cumulative doses above 500 mg/m2 [98]. There are no randomized studies of pegylated doxorubicin in children.
10.3.1.4 Novel Anthracycline Analogues
Epirubicin, an epimer of doxorubicin, exhibits less cumulative dose cardiotoxicity than doxorubicin. Epirubicin was associated with cardiotoxicity in 3 % of patients at a cumulative dose of 900 mg/m2 and 10 % at 1,000 mg/m2. Doxorubicin had a 5 % incidence at 500 mg/m2 and 15 % at greater than 600 mg/m2, almost double that of epirubicin [99]. One study found similar efficacy rates when comparing non-pegylated liposomal doxorubicin and epirubicin in 160 patients [100].
However, several studies suggest epirubicin may cause subclinical cardiac injury [71]. While no CHF was noted for patients receiving epirubicin at a cumulative dose of 400–500 mg/m2, the LVEF was decreased significantly in one third of patients at a dose of 450 mg/m2 [101]. While clinical cardiotoxicity is decreased in the short term, long-term follow-up is necessary to adequately assess epirubicin toxicity.
Idarubicin is an analogue of doxorubicin that has been shown to exhibit decreased cardiotoxicity when compared to doxorubicin. While providing a decrease in cardiomyopathy (5 % at its target dose of 150–290 mg/m2) [102], subclinical cardiac effects were observed at lower cumulative doses (150 mg/m2) [102]. Similar to epirubicin, idarubicin appears to provide less clinical cardiotoxicity but subclinical effects remain.
Mitoxantrone is part of the anthracenedione family but is structurally similar to anthracycline. This class has antiviral, antibacterial, immunomodulatory, and antitumor properties. While mitoxantrone has been shown to be effective in treating various malignancies, the risk of CHF remains elevated compared to other antineoplastic therapies. The risk of CHF at the upper limit of dosing (140 mg/m2) is 2.6 % (Table 10.1) [103]. However, in evaluating subclinical cardiac function, a decrease in LVEF to less than 50 % was seen in 2 % of patients [103].
Monoclonal antibodies against vascular endothelial growth factor, such as bevacizumab and trastuzumab, are used to treat a variety of solid tumors. These antibodies affect myocardial homeostasis and can lead to changes in cardiomyocytes function and metabolism [104]. One randomized controlled study found that when combined with anthracycline treatment, bevacizumab was associated with heart failure in 3.8 % of these adult patients [105]. It is unknown whether bevacizumab enhances cardiomyopathy in children.
10.3.2 Radiation Exposure and Cardiac Toxicity
Radiation-induced CVD is a major cause of morbidity and mortality in pediatric patients receiving mediastinal radiation. Complications can be both acute and chronic and include pericarditis, CAD, cardiomyopathy, valvular defects, veno-occlusive disease, and conduction abnormalities. Methods to prevent these complications include assessing patient risk factors and adjusting dose and size of the radiation portal. Doses greater than 40 Gy have been associated with increased cardiac mortality [106].
The onset of radiation-induced cardiotoxicity typically is 10 or more years following completion of therapy. Thickening of the pericardium due to high doses of mediastinal radiation has become rare with modern day practices. Complications such as restrictive cardiomyopathy and myocardial infarction are more prevalent in children treated with radiation. Nonetheless, the cohort of survivors who received high-dose mediastinal radiation is aging, and data suggest that they will be experiencing the complications outlined herein. Unlike others who have cardiac disease, the clinical symptoms of myocardial infarctions can be harder to detect or even nonexistent in this population [107].
Also, radiotherapy is associated with diastolic dysfunction and anthracyclines with systolic dysfunction. One study evaluated cardiac status of 48 long-term survivors of Hodgkin lymphoma treated with mantle irradiation [108]. At a median follow-up of 14 years, LV systolic dysfunction was found in five (10 %) of the survivors, although three of these five had also been treated with anthracyclines. On the other hand, 18 (37 %) had LV measurements indicating diastolic dysfunction.
Valvular dysfunction is an increasingly common finding in these patients. In the same study previously mentioned, Adams et al. found at least one heart valve abnormality in 20 (43 %) of the survivors [108]. Other studies have found similar echocardiographic findings [109–112]. Valvular heart disease may be progressive and can contribute to CHF [62].
10.3.2.1 Dose Reduction
Historically, radiation doses of 30–40 Gy were used in pediatric Hodgkin lymphoma and were associated with subsequent cardiotoxicity. Current radiation exposure is at or below doses of 25 Gy [113], much less than the aforementioned 40 Gy causing clinical cardiotoxicity. Further studies of cardiotoxic events at this level are necessary, and follow-up of the patients who received the much higher doses of radiotherapy should be closely pursued.
Reduction of the volume of radiation received by the patient has been achieved through the utilization of involved-field radiotherapy as opposed to mantle radiotherapy. This technique reduced the relative risk of cardiac-related death from 5.3 to 1.4 in patients treated for Hodgkin lymphoma [114]. Similar reductions of predicted risk have been reported by others [115, 116]. Subcarinal blocking of the LV has also been found to reduce the incidence of pericarditis [117]. Further reductions in radiation volume are now being tested and include involved site and involved node radiation therapy; both approaches will decrease the dose to the heart [118].
10.3.2.2 Targeted Therapy
Three-dimensional conformal radiation therapy and proton therapy are currently being studied for their ability to reduce dose of radiation received by cardiac structures. Three-dimensional conformal radiation therapy uses CT imaging to gather tissue density and depth information which assists in the calculation of dose distribution, with the radiation fields delivering this dose from multiple angles. Proton therapy uses charged particles as opposed to high-energy photons to deliver therapeutic radiation. These charged particles have reduced exit dose as they leave normal tissue, which may confer reduced toxicity. Further research is required to determine the capability of these contemporary techniques in reducing radiation exposure to cardiac structures.
Respiratory maneuvers during radiation, such as quiet breathing or breath holding, may decrease radiation to the heart and, thus, cardiotoxicity. In one study of breast cancer patients, deep inspiration was performed, which displaces the heart caudally. In 21 % of patients, the heart was displaced out of the radiation field [119].
10.4 Detection and Screening
10.4.1 Cardiac Considerations
Assessment of multiple studies has shown that childhood cancer survivors are at increased risk of CVD compared to the general population as early as 1 year after treatment [120] and for at least 45 years after initial diagnosis [121, 122]. Studies comparing long-term survivors to their siblings have shown higher rates of CHF, myocardial infarction, valvular disease [123], and subclinical dysfunction [124]. Death from cardiac disease is the third leading cause of death in long-term survivors [120, 121, 125]. Long-term survivors of childhood cancer who received potentially cardiotoxic therapies should undergo regular, repeated evaluations of cardiac status, even if the patient is asymptomatic. Although screening regimens have been suggested for patients treated with doxorubicin and/or irradiation [49, 126–129], no specific regimen for childhood cancer survivors has ever been tested for efficacy and cost-effectiveness. Nevertheless, serial evaluation recognizes the following: (1) as the survivor grows and matures, demands on the heart increase, which a damaged heart at some point may no longer be able to meet, (2) lifestyle changes may further stress the heart, and (3) cancer therapy-related heart disease may itself be progressive.
Furthermore, survivors need to be screened as they undertake or contemplate changes in their life that increase cardiac stress such as beginning a new exercise program, starting growth hormone therapy, becoming pregnant, or undergoing anesthesia. It has been shown that growth hormone deficiency is seen after radiation to the hypothalamic-pituitary axis, which many long-term survivors have received. The LV wall, which has been shown to be thinned after anthracycline and radiation treatment (Fig. 10.2), is increased in diameter after growth hormone therapy is started. However, this change only lasts as long as growth hormone replacement therapy is continued [130]. Clearly, those patients who have had an abnormal finding or study, let alone a symptomatic cardiac event at any time, should have more frequent cardiac screening. The importance of screening all survivors treated with potentially cardiotoxic therapy is underscored by the fact that Lipshultz and colleagues could not find a correlation between patient- or parent-reported symptoms and measures of LV function or exercise tolerance in those treated for ALL with doxorubicin with or without chest irradiation [21].
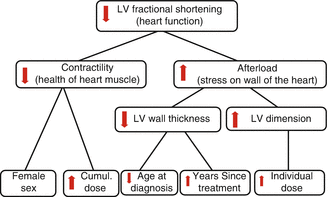
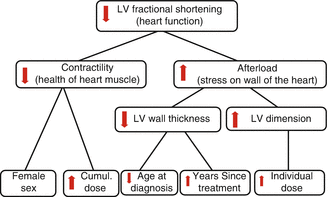
Fig. 10.2
Marginal (Kaplan-Meier) and (C-E) cause-specific (competing risk) cumulative incidence of cardiac events (CEs) in childhood cancer survivors. Marginal cumulative incidence for all CEs, stratified according to potential cardiotoxic (CTX) therapy or no CTX therapy, log-rank p < 0.001 (Reprinted with permission from the publisher Wouters et al. [89])
Longer follow-up is important since it is known that both the prevalence and severity of LV abnormalities increase over time (Fig. 10.3) [81, 131]. Lipshultz et al. demonstrated an increased incidence of LV dysfunction when increasing the length of follow-up of 120 patients [81]. Acute LV dysfunction can be treated with anticongestive therapy and usually shows an initial improvement, but a progressive decline is expected even with appropriate long-term medications. In following pediatric cancer survivors at 6 years after anthracycline administration, it was found that 65 % had silent cardiac dysfunction [21, 69, 81]. Pregnancy, infection, cocaine use, and demanding exercise regimens may not be the only stressors that precipitate CHF. Although one study found no cases of peripartum cardiomyopathy in following 53 survivors at a mean follow-up of 20 years [132], the study is not adequately powered. Further research on a larger scale should be conducted to investigate stressors and their effects on the heart. Clinically significant cardiotoxicity such as CHF or cardiac rhythm-related symptoms can initially appear either during the third trimester of pregnancy or during the first few months postpartum and may be attributable to intravascular volume increases during pregnancy or to other pregnancy-associated changes. Pregnancy should be considered to be a cardiac stressor for survivors and follow-up as high-risk patients is recommended. Screening protocols should include close serial monitoring with involvement of a multidisciplinary team approach. There is need for continued cardiac monitoring, even in asymptomatic patients, particularly during stressful circumstances [133].
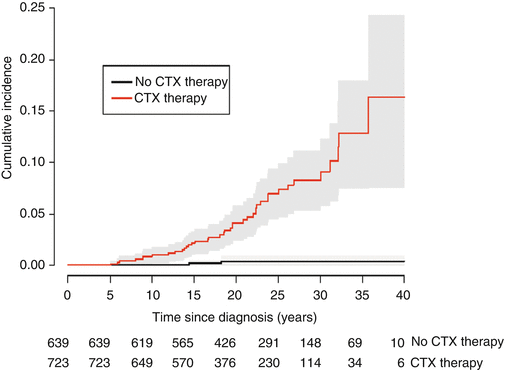
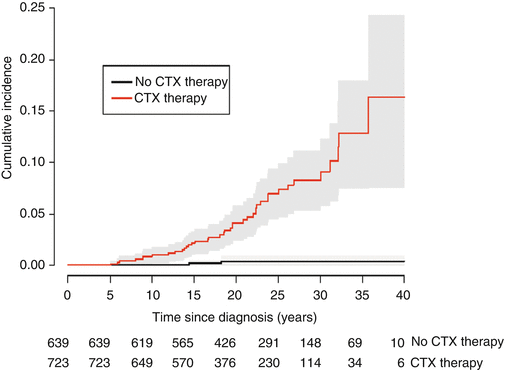
Fig. 10.3
The relationships between cardiac and noncardiac findings in long-term survivors of childhood malignancy treated with anthracycline therapy (Reprinted with permission from the publisher Van der Pal et al. [248]
Cardiac evaluation of the survivor should first begin with a thorough annual history and physical examination [126]. Self-reported symptoms without specific questioning do not necessarily correlate well with cardiovascular abnormalities detected by specialized testing; it is essential that information be determined by specific, quantitative parameters (e.g., “Can you walk up two flights of stairs without becoming short of breath?”). Changes in exercise tolerance, dyspnea on exertion, palpitations, and syncope should all be evaluated by specific screening modalities because they may be the manifestations of the late anthracycline-related cardiotoxicity (CHF and arrhythmias). Worrisome findings on physical examination include tachypnea, tachycardia, gallops, rales, hepatomegaly, peripheral edema, and diminished peripheral pulse volume and perfusion, any of which is suggestive of cardiac abnormalities.
The history should not only evaluate risk from therapy but also traditional CVD risk factors such as hypertension, tobacco abuse, diabetes, family history of early CVD, gender, and age. Landy et al. using the traditional scoring systems for CVD risk (the Pathobiological Determinants of Atherosclerosis in Youth (PDAY) and the Framingham Risk Calculator (FRC)) found that long-term survivors had a similar risk profile to their siblings. While a higher proportion of survivors had PDAY odds ratios and FRC risk ratio(s) greater than 4, this was not statistically significant possibly suggesting that the increased risk of CVD is truly due to therapeutic cardiotoxicity [128].
10.4.1.1 Assessment of Coronary Artery Disease
All childhood cancer survivors should be screened regularly for CAD risk factors. While those treated with mantle radiation are probably most at risk for CAD, survivors treated with anthracyclines and high-dose cyclophosphamide may also have damaged hearts that can ill afford further compromise from myocardial ischemia or infarction. Patients who received brain irradiation, especially those with proven growth hormone deficiency or other hypothalamic-pituitary axis dysfunction, may also be at higher risk, compared with other survivors. Risk factor for CAD, such as family history, hypertension, tobacco abuse, hyperlipidemia [134], obesity, diabetes mellitus, and a sedentary lifestyle, should be evaluated at each long-term visit. Counseling to reduce such risk factors is extremely important. Signs and symptoms of pericarditis, cardiomyopathy, valvular disease, arrhythmia, and CAD should all raise concern.
As in other children, chest pain is usually due to costochondritis (Tietze’s syndrome) in young survivors and is characterized by point tenderness, discomfort with inspiration, and response to nonsteroidal analgesics. However, because of the sharply increased risk of myocardial infarction in survivors treated with mediastinal irradiation, myocardial infarction should be ruled out in any such patient who presents acutely. In a study of 294 survivors of Hodgkin disease who received mediastinal radiation of 35 Gy, 18.4 % (54 of 294) had abnormal stress testing, and on invasive coronary angiography, 22 had coronary stenoses of more than 50 % of the luminal diameter [135]. Although the possibility of myocardial ischemia is strongly suggested by crushing pain (especially with exercise), diaphoresis, dyspnea, and nausea, the absence of these symptoms does not necessarily exclude ischemia because chest irradiation may cause individuals to feel cardiac pain abnormally [136]. Complaints of chest pain, even in young survivors treated with mediastinal irradiation, require additional evaluation.
Noninvasive Coronary Artery Imaging
In 2008, the American Heart Association published a scientific statement regarding noninvasive coronary artery imaging comparing magnetic resonance angiography and multidetector CT angiography [137]. With regard to detection of coronary atherosclerosis, studies of magnetic resonance angiography at multiple centers show a sensitivity ranging from 65 to 93 % and specificity of 42–100 %. A meta-analysis of 64-slice CT angiography found 6 studies with sensitivity of 93 % and specificity of 96 % and found 19 studies with a sensitivity of 86 % and specificity of 96 %. Each of these two modalities has its benefits and limitations. CT angiography has higher spatial resolution with a shorter examination time and wider availability when compared to magnetic resonance angiography, while magnetic resonance angiography has no exposure to ionizing radiation or iodinated contrast. The summary recommendations state that neither modality should be used for screening asymptomatic patients, but both have potential benefit for symptomatic patients with intermediate coronary risk by standard criteria. Patients with high pretest likelihood for stenosis should undergo invasive angiography as opposed to CT angiography due to likelihood of need for intervention [137].
Invasive Procedures
In all survivors treated with potentially cardiotoxic therapies, angiography and cardiac catheterization are appropriate for evaluating symptomatic disease (angina and CHF, respectively). They are not appropriate for routine serial screening in the asymptomatic survivor. According to some experts, however, these procedures should be performed if any clinically significant cardiac lesion is found due to the fact that CAD can be asymptomatic and is often present in those who received mediastinal irradiation [138].
10.4.1.2 Assessment of Cardiomyopathy and Congestive Heart Failure
Imaging
The most common screening methods for anthracycline-associated cardiomyopathy are echocardiograms, radionuclide ventriculography – also commonly referred to as radionuclide angiography (RNA) – and ECGs. These methods are also useful for screening for radiation-associated cardiotoxicity with subtle differences. Echocardiography and RNA are both excellent methods of measuring LV systolic function, but echocardiography has the advantage over RNA of being noninvasive. Echocardiography also has the advantage of being able to evaluate heart structures such as the pericardium and valves. Unfortunately, it can be technically difficult in adults due to body habitus and bone density.
Fractional shortening and ejection fraction are reliable echocardiographic measures of LV systolic function. Fractional shortening is the percentage of change in LV dimension between systole and diastole. Both measurements, however, are dependent on loading conditions, which may vary considerably, particularly during chemotherapy [139, 140]. Load-independent measures of cardiomyocyte health and myocardial function can be provided by measurements of wall stress and contractility, i.e., the relationship between wall stress and the heart rate corrected velocity of circumferential fiber shortening [140]. Additionally, anatomic measurements such as posterior wall thickness and LV dimension are useful. Current monitoring recommendations include obtaining echocardiograms of these measurements at regular intervals after therapy [126, 129]. Several studies show abnormal LVFS in long-term survivors versus controls [24, 141, 142]. The recommended monitoring intervals depend on cumulative anthracyclines dose, other therapies received, the detection of abnormalities, any symptoms the patient may experience, and coexistent stressors such as growth or participation in competitive athletics.
Echocardiographic modalities such as strain and strain rate are used to measure regional myocardial wall motion as well as LV systolic and diastolic function. Adult studies on breast cancer patients have found that strain rate imaging allowed for earlier and better detection of subtle changes in preclinical cardiac function such as longitudinal LV function [74, 143–145]. Although studies have found high positive predictive value for longitudinal strain, this measure depends partially on the prevalence of the condition being studied. Although positive predictive value gives the probability that a positive screening test will correctly identify an outcome of interest, it does not validate a test as cost-effective or practical. Further research is required to elucidate the validity of strain and strain rate as surrogate measures of LV function in childhood cancer survivors [146].
Radionuclide ventriculography determines an ejection fraction that is arguably more accurate than that derived from echocardiography by calculating the change in radioactivity at end diastole to that at end systole. However, this measure is load-dependent, and no load-independent measure of cardiomyocyte health exists for this modality. Although it has been recommended as a baseline study to be repeated every 5 years in anthracycline-treated children (in addition to more frequent echocardiography [109, 126, 127]), further studies are necessary to determine whether RNA is of clinical value for the entire cohort of asymptomatic patients. It is clearly useful for those in whom a good echocardiogram cannot be obtained, which is frequently the case in adults. However, it should be noted that the LVFS on echocardiography and the ejection fraction on RNA are not directly convertible.
Myocardial performance index is a ratio of isovolumic contraction and relaxation times to ejection time and is a newer form of evaluation of cardiac dysfunction using Doppler echocardiography. Changes in myocardial performance index can occur before changes in the echocardiographic measures of LV function suggesting that myocardial performance index may also be a useful tool in evaluation of early asymptomatic cardiac dysfunction [147, 148].
A significant difference in anthracyclines and radiation-associated cardiomyopathy is the type of cardiac dysfunction caused. Although otherwise quite different from other types of dilated cardiomyopathy [13], anthracycline-associated cardiomyopathy resembles dilated cardiomyopathy because it primarily causes diastolic dysfunction. Lipshultz et al. found that survivors of childhood ALL treated with anthracyclines initially developed dilated cardiomyopathy as evidenced by reduced shortening fractions and contractility with LV dilation [3]. As time progressed, they developed signs of restrictive cardiomyopathy such as smaller LV dimensions and decreased wall thickness [73] (Fig. 10.4). Radiation damage differs from that of anthracyclines as it causes restrictive cardiomyopathy, which primarily leads to diastolic dysfunction (i.e., problems with filling the heart). Both conditions, however, can cause CHF in the long term. Therefore, it is important to screen for both with the hope that early intervention can delay or prevent CHF. The problem is that measuring diastolic function noninvasively can only be done through imprecise echocardiographic techniques. In addition to measuring LV wall stress, LVFS, and LV wall thickness, measuring LV mass and LV chamber size is recommended, which, if abnormal, may suggest restrictive cardiomyopathy. Measuring the ratio of peak early filling to peak late filling of the LV (E/A ratio) and other indirect echocardiographic indicators of diastolic function is also recommended. Brouwer et al. showed increasing rates of diastolic dysfunction in a cohort of long-term survivors of bone tumors treated with doxorubicin when evaluated at 9 and 14 years posttreatment [149]. Echocardiography is also useful for following valvular and pericardial status. Recommendations for frequency of monitoring of cardiac function are based on age at time of treatment, anthracycline dosage, and whether combined modalities of therapy are used [126, 129]. Lifetime health care cost of echocardiographic screening using Children’s Oncology Group guidelines is approximately $500 million for 100,000 anthracycline-exposed survivors (2010 US dollars) or $5,000 per patient and was predicted to extend quality-adjusted life years by 1.8 months and to delay CHF by 8.4 months [150].
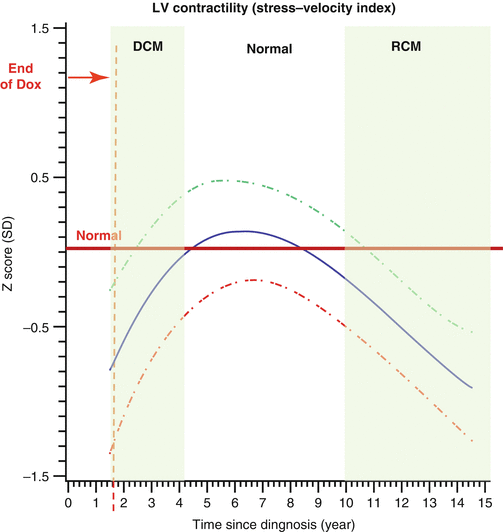
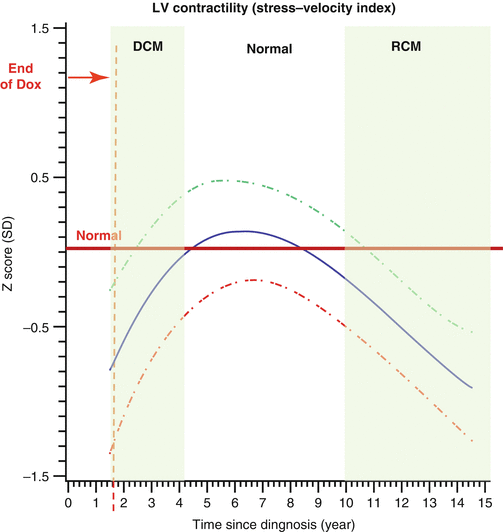
Fig. 10.4
Z-scores of cardiac measurements from 115 long-term survivors of acute lymphoblastic leukemia treated with doxorubicin, by time since diagnosis (years). The solid line is the overall group mean in this model. The dashed lines are the upper and lower 95 % CIs from the predicted mean 2 SEs of the mean. Left ventricular (LV) contractility (stress-velocity index) (Reprinted with permission from the publisher Lipshultz [3])
Biomarkers
Blood cardiac biomarkers are garnering interest for the detection of cardiac dysfunction during and after therapy, including in long-term survivors. These blood cardiac biomarkers include cardiac troponin T (cTnT) and N-terminal pro-B-type natriuretic peptide (NT-pro-BNP). Lipshultz et al. demonstrated that during and immediately after therapy with doxorubicin with or without dexrazoxane, both cTnT and NT-pro-BNP are elevated in some patients. Dexrazoxane seems to offer protection as a smaller percentage of patients had elevations of either biomarker at all points in the study (Fig. 10.5a, b) [17]. A study of 122 survivors showed no elevations in cTnT. However, 13 % of these patients had abnormal NT-pro-BNP, which correlated with cumulative anthracycline doses. Only 3 % and 7 %, respectively, had a LVFS less than 29 % and LVEF less than 55 % suggesting that NT-pro-BNP may provide earlier detection of cardiac dysfunction, but at this time study size is a significant limitation to development of recommendations [151].
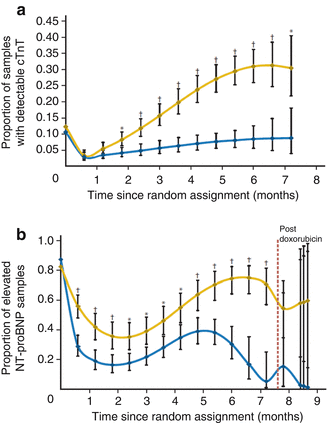
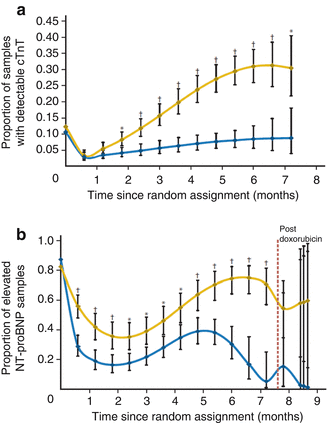
Fig. 10.5
(a) Model-based estimated probability of having an increased cardiac troponin T (cTnT) level at each depicted time point in patients treated with doxorubicin, with or without dexrazoxane (Reprinted with permission from the publisher Lipshultz et al. [12]). (b) Model-based estimated probability of having an increased N-terminal pro-brain natriuretic peptide (NT-proBNP) level at each depicted time point in patients treated with doxorubicin with or without dexrazoxane (Reprinted with permission from the publisher Lipshultz [12])
10.4.1.3 Assessment of Dysrhythmias/Conduction Abnormalities
Electrical conduction abnormalities and rhythm disturbances may remain silent until fatal. Serial screening ECGs may identify potentially fatal arrhythmias such as prolonged corrected QT interval, atrioventricular conduction delay, ventricular ectopy, low voltage, second-degree AV block, complete heart block, ST elevation (or depression), and T wave changes. Recommendations from the CVD Task Force of the Children’s Oncology Group include a baseline ECG at the completion of therapy and, for those with prolonged corrected QT interval, counseling on avoidance of potentially dangerous medications (i.e., tricyclic antidepressants, antifungals, and macrolides) [126].
No study conclusively establishes what screening tool and with what frequency it should be used. Steinherz et al. recommended performing 24-h ECG every 5 years in long-term survivors who had received anthracyclines, and this proposal is commonly followed. No large study has been done that conclusively demonstrates with what frequency screening should be undertaken, although the importance in doing so is clear.
10.4.1.4 Cardiometabolic Screening
Heart-healthy lifestyles should be encouraged in long-term survivors of cancers treated with cardiotoxic therapies even if the patient is asymptomatic [152]. Those who have received anthracyclines, high-dose cyclophosphamide and cardiac radiation are at highest risk of CHF, and cardiac mortality occurs at increased rates within the first decades after cardiotoxic treatments [153]. Protocols for screening those who have survived cardiotoxic therapy have not been tested for efficacy, but a wide scope of monitoring should be considered, including assessment of both modifiable and non-modifiable risk factors. Relevant screening methods include detailed treatment history, assessment of independent cardiovascular risk factors, and detailed review of systems [126]. As the heart endures continued stress, it may not be able to meet the demand. Moreover, cardiac disease may itself be progressive in the setting of cardiotoxic therapies [154]. The Children’s Oncology Group consensus statement of 2008 recommends that risk factors should be identified and therapies tailored to the details of the cardiotoxic regimen [126]. When lifestyle changes require an increase in cardiac demand, whether in the form of a physically demanding job or new exercise regimen, screening tests may need to be performed. Moreover, screening is important because symptoms do not consistently correlate with cardiac performance as measured by echocardiographic variables such as LV function [133]. Screening to assess for cardiac dysfunction should be undertaken regularly and aimed at addressing problems earlier rather than later.
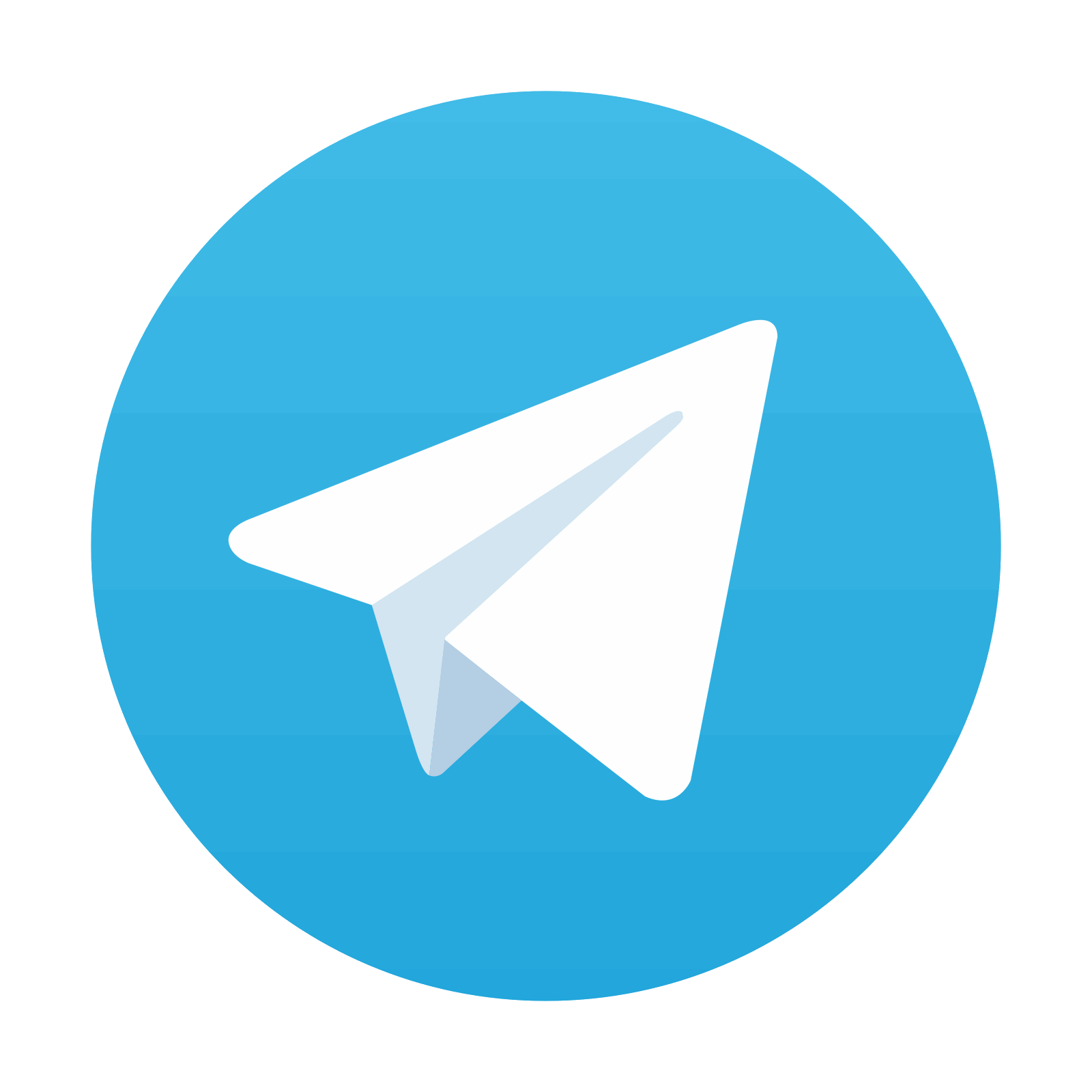
Stay updated, free articles. Join our Telegram channel

Full access? Get Clinical Tree
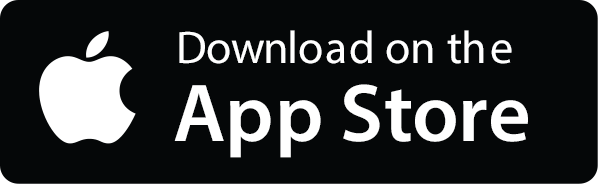
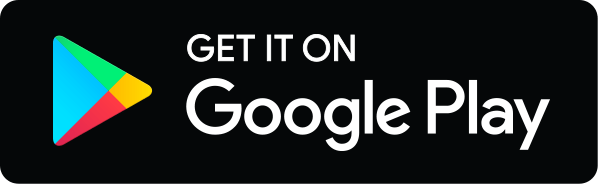
