(1)
Cancer Survivorship, Childern’s Hospital of Philadelphia, Perelman School of Medicine at the University of Pennsylania, Philadelphia, USA
14.1 Pathophysiology
14.1.2 Anatomy of Normal Testis
14.1.4 Normal Developmental Stages
14.3.1 Effects of Chemotherapy
14.3.2 Effects of Radiation
14.3.2.1 Leydig Cell Function Following RT
14.3.2.3 Summary
The testes are extremely sensitive to chemotherapy, radiation, and surgical interventions. Cancer therapy can interfere with reproductive ability and libido in men. The differential sensitivity of spermatozoa-producing Sertoli cells, however, compared to the testosterone-producing Leydig cells allows for greater effects on the reproductive capacity of men than effects on their sexual function. In this chapter, we review the pathophysiology of the testes and clinical manifestations of the testicular injury in response to gonadotoxic therapies. Furthermore, we will outline methods for screening males for gonadotoxicity and suggest potential fertility preservation methods that can be used in certain instances.
14.1 Pathophysiology
14.1.1 Overview of Normal Gonadal Development
Although the chromosomal and genetic sex of an embryo is determined at fertilization, male and female morphological sexual characteristics do not differ until the seventh week of gestation [49]. This initial period of early genital development is referred to as the indifferent stage of sexual development. During the fifth week, proliferation of the mesothelial cells and of the underlying mesenchyme produces a bulge on the medial side of the mesonephros, known as the gonadal ridge. Fingerlike epithelial cords then grow into the underlying mesenchyme. The indifferent gonad now consists of an outer cortex and inner medulla [49]. During the sixth week, the primordial germ cells enter the underlying mesenchyme and incorporate into the primary sex cords. In embryos with an XY sex chromosome complex, testis-organizing factor (H-Y antigen) regulated by the Y chromosome determines testicular differentiation; the medulla differentiates into a testis and the cortex regresses. The gonads can be recognized as testes 7–9 weeks postfertilization [49].
The first stage of testis differentiation is the formation of testicular cords consisting of Sertoli precursor cells packed tightly around germ cells. The diploid germ cells undergo meiosis in the fetal testis and remain in meiotic arrest until puberty. Sertoli cells, which provide a location for support and proliferation of spermatogonia are derived from the mesonephros and proliferate only during fetal life and in the neonatal period [77]. After the eighth week of fetal life, the Leydig cell of the fetal testis secretes testosterone. Luteinizing hormone (LH) release is suppressed, and masculinization of the external genitalia and urogenital sinus of the fetus results. By the third month, the penis and prostate form [14]. Normal testes descend by the seventh month of gestation.
14.1.2 Anatomy of Normal Testis
The adult testis is an oblong, approximately 4.5 cm long organ weighing 34–45 g [76]. The testis is composed of three principal cell types: germ cells that develop into sperm, Sertoli cells that support and nurture developing germ cells and are also the site of production of the glycoprotein hormone inhibin, and Leydig cells that are responsible for testosterone synthesis [70]. Seminiferous tubules, the sites of spermatogenesis, are formed by germ cells and Sertoli cells. The Leydig cells that are responsible for the production of testosterone lie near the basal compartment of the seminiferous tubules, enabling them to deliver high concentrations of testosterone necessary for normal spermatogenesis and male secondary sexual characteristics [70].
The seminiferous tubules are embedded in a connective tissue matrix containing interspersed Leydig cells, blood vessels, and lymphatics and are surrounded by a basement membrane (tunica propria) upon which the seminiferous epithelium rests. Spermatogenesis takes place in the seminiferous epithelium. The least differentiated germ cells, the spermatogonia, divide to form spermatocytes that immediately undergo meiosis. The haploid spermatids that are formed develop into flagellate motile spermatozoa. This process requires up to 74 days [73]. Since spermatozoa are continuously produced in adult men, a constant supply of germ cell precursors is necessary. The newly formed spermatozoa are transported through the lumen of the seminiferous tubules into the epididymis where they are stored.
14.1.3 Hypothalamic-Pituitary-Testicular Axis
The primary regulators of testicular function are the anterior pituitary hormones, luteinizing hormone (LH) and follicle-stimulating hormone (FSH), both of which are released in response to the hypothalamic releasing factor, GnRH. GnRH is secreted from the median eminence into the hypophyseal portal system in a pulsatile manner and acts on the gonadotropes of the pituitary gland to stimulate secretion of LH and FSH [49]. LH regulates Leydig cell function by binding to specific LH receptors on the plasma membrane of Leydig cells. This leads to the formation of the cAMP that drives testosterone biosynthesis via a complex cascade starting with cholesterol [49]. Testosterone is transported from Leydig cells to the seminiferous tubules, where it acts to enhance spermatogenesis. Testosterone is also a prohormone for two different and metabolically active hormones, dihydrotestosterone (DHT) and estradiol. DHT mediates male sexual differentiation and virilization, whereas estradiol mediates bone maturation, mineralization, and epiphyseal fusion [72]. Testosterone controls pituitary LH secretion by a negative feedback mechanism; LH levels will rise when the Leydig cells are unable to produce testosterone.
Sertoli cells are the only cell type of the testis that possess FSH receptors [70]. FSH is delivered to the interstitial area of the testis by way of the arterial system. It then passes through the basement membrane of the seminiferous tubule to reach the Sertoli cells that line this membrane. FSH binds to the FSH receptors of the Sertoli cell and then mediates the synthesis of a variety of proteins and enzymes including the inhibins. Inhibin is a major feedback regulator of FSH and may thus be useful as a marker of spermatogenesis [66, 80]. The production of androgen transport protein by Sertoli cells can be induced and maintained by FSH.
FSH triggers an event in the immature testis that is essential for the completion of spermiogenesis. Thereafter, spermatogenesis will proceed continuously as long as an adequate and uninterrupted supply of testosterone is available. The lack of negative feedback from germinal epithelium results in an elevated FSH level.
14.1.4 Normal Developmental Stages
A neonatal surge in testosterone secretion is caused by high LH and FSH concentrations that occur during the first few months of life. From the age of about 3 months until the onset of puberty, plasma concentrations of LH and FSH are quite low and the testes are relatively quiescent [70]. At the onset of puberty, pulsatile secretion of LH (and to a lesser extent, FSH) occurs during sleep that is associated with increased nighttime plasma concentrations of testosterone. As puberty progresses, the increased pulsatile release of gonadotropins and the high concentrations of testosterone are maintained throughout the day and night [70].
In a normal male, the first sign of puberty is enlargement of the testis to larger than 2.5 cm [77]. This is due to seminiferous tubule growth, although Leydig cell enlargement contributes as well. Androgens synthesized in the testes are the driving force behind secondary sexual development, although adrenal androgens also play a role in normal puberty. The range of onset of normal male puberty extends from 9 to 14 years of age. Boys complete pubertal development in 2–4.5 years (mean 3.2 years) [77]. The development of the external genitalia and pubic hair has been described in stages by Marshall and Tanner (Table 14.1) [47]. The first appearance of spermatozoa in early morning urinary specimens (spermarche) occurs at a mean age of 13.4 years, at gonadal stages 3 and 4 simultaneous with the pubertal growth spurt.
Stage | Description | Mean age at onset (year) range 95 % |
---|---|---|
1 | Preadolescent. Testes, scrotum, and penis are about same size and proportion as in early childhood. No pubic hair | |
2 | Scrotum and testes have enlarged; change in texture and some reddening of the scrotal skin. Testicular length >2 cm <3.2 cm. Sparse growth of long, slightly pigmented downy pubic hair, straight or only slightly curled appearing chiefly at base of penis | 11.6 9.5–13.8 |
3 | Growth of penis has occurred, at first mainly in length but some increase in breadth; further growth of testes and scrotum. Testicular length >3.3 cm <4.0 cm. Pubic hair is considerably darker, coarser, and curlier and spreads sparsely | 12.9 10.8–14.9 |
4 | Penis further enlarged in length and breadth with development of glans. Testes and scrotum are further enlarged. Scrotal skin has darkened. Testicular length >4.1 cm <4.9 cm. Pubic hair is now adult in type, but the area it covers is still smaller than most adults. There is no spread to medial surface of the thighs | 13.8 11.7–15.8 |
5 | Genitalia are adult in size and shape. No further enlargement takes place after stage 5 is reached. Testicular length >5 cm. Pubic hair is adult in quantity and type, distributed as an inverse triangle. The spread is to the medial surface of the thighs | 14.9 12.7–17.1 |
14.2 Cytotoxic Effects of Therapy
14.2.1 Cytotoxic Effects of Chemotherapy
Testicular dysfunction is among the most common long-term side effects of chemotherapy in men. The germinal epithelium is particularly susceptible to injury by cytotoxic drugs secondary to a high mitotic rate [30]. Leydig cells in contrast appear relatively resistant to the effects of chemotherapy [79]. In 1948, azoospermia after an alkylating agent (nitrogen mustard) was described in 27 of 30 men treated for lymphoma [75]. Subsequently, it has become apparent that all alkylating agents are gonadotoxic [26, 46, 55]. Antimetabolite therapy in general (e.g., methotrexate, mercaptopurine) does not have an adverse impact on male fertility. Cisplatin-based regimens result in temporary impairment of spermatogenesis in all patients but with recovery in a significant percentage [23]. The agents most commonly gonadotoxic in males are listed in Table 14.2.
Table 14.2
Gonadotoxic chemotherapeutic agents and associated cumulative doses that impact male fertility
Gonadotoxic agents | Cumulative doses |
---|---|
Cyclophosphamide | 7 g/m2 |
Ifosfamide | 42–60 g/m2 |
Nitrosoureas, e.g., BCNU and CCNU | 1 g/m2 and 500 mg/m2 |
Chlorambucil | 1.4 g/m2 |
Melphalan | 140 mg/m2 |
Busulfan | 600 mg/m2 |
Procarbazine | 4 g/m2 |
Cisplatin | 500 mg/m2 |
Initial reports, based upon histological studies and normal basal FSH levels from small numbers of patients, suggested that the immature testis was relatively resistant to chemotherapy. More recently, however, it has become apparent that both the prepubertal and pubertal testes are vulnerable to cytotoxic drugs [6, 30]. Impairment of spermatogenesis may be irreversible in the months to years following chemotherapy. However, late recovery of spermatogenesis up to 14 years following chemotherapy has been reported [30]. In summary, testicular damage is agent-specific and dose-related. The chance of recovery of spermatogenesis following cytotoxic chemotherapy and the extent and speed of recovery are related to the agent used and the dose received [48]. In contrast to the germinal epithelium, Leydig cells appear relatively resistant to the effects of chemotherapy [68]. However, a few studies have demonstrated a reduction in testosterone concentrations following treatment with gonadotoxic agents, and there is evidence to suggest that the Leydig cell impairment following chemotherapy may be relevant clinically.
14.2.2 Cytotoxic Effects of Testicular Irradiation
Soon after the discovery of X-rays by Roentgen, investigators noted that spermatogenesis is exquisitely sensitive to radiation [60]. The testes are directly irradiated in rare situations such as testicular involvement of acute lymphoblastic leukemia (ALL). Although the testes are usually not directly in the radiation field, they can still receive irradiation via body scatter. Scatter occurs when X-rays interact with tissues near the target of interest, resulting in secondary X-rays that then hit the target [37]. The amount of scattered radiation is a function of the proximity of the radiation field to the target, the field size and shape, the X-ray energy, and the depth of the target. Of these, distance from the field edge is the most important factor. Scatter dose to the testes becomes a real issue when treating a field that extends into the pelvis as in some cases of Hodgkin’s disease or soft tissue sarcoma of the thigh. Small children, because of their short trunk length, can be at greater risk from scattered radiation than larger individuals.
The germinal epithelium is most sensitive to radiation effects and some effect on spermatogenesis will be seen at doses of 10 cGy (Table 14.3) [3]. Permanent sterilization may be seen with doses as low as 100 cGy [3]. Speiser et al. reviewed experimental data in mammals that indicated that the total radiation dose required to induce permanent azoospermia was lower if a fractionated regimen was used than if a single dose was given [74]. The details of these experiments cannot be extrapolated to humans because there are significant differences in germ cell radiosensitivity between different species. However, the general conclusion that fractionated radiation is more effective than single doses in destroying germ cells appears to be true in humans as well. The explanation given for this is that at any given time, some percentage of the spermatogonia are relatively radioresistant because they are not proliferating. A single large dose of radiation may not kill these cells. However, when fractionated radiation is delivered, there is a chance that these cells will enter the proliferating compartment and be susceptible to radiation-induced killing. Therefore, the most efficient regimen for spermatogonial killing would be one in which each fraction is maximally efficient in causing death in proliferating spermatogonia but in which there are a sufficient number of fractions such that all the spermatogonia are eventually irradiated when they are in a proliferative, radiosensitive state.
Table 14.3
Impairment of spermatogenesis and Leydig cell function after fractionated radiotherapy [3]
Testicular dose (cGy) | Effect on spermatogenesis | Effect on Leydig cell function |
---|---|---|
<10 | No effect | No effect |
10–30 | Temporary oligospermia | No effect |
30–50 | Temporary azoospermia at 4–12 months after radiation. 100 % recovery by 48 months | No effect |
50–100 | 100 % temporary azoospermia for 3–17 months after radiation Recovery begins at 8–26 months | |
100–200 | 100 % azoospermia from 2 months to at least 9 months. Recovery begins at 11–20 months | Transient rise in LH No change in testosterone |
200–300 | 100 % azoospermia beginning at 1–2 months. May lead to permanent azoospermia. If recovery takes place, it may take years | Transient rise in LH No change in testosterone |
1,200 | Permanent azoospermia | Elevated LH. Some patients may have decreased basal testosterone or in response to HCG stimulation. Replacement therapy not needed to ensure pubertal changes in most boys |
2,400 | Permanent azoospermia | Elevated LH. Many patients, but not all, will have decreased testosterone. Replacement therapy needed to ensure pubertal changes in most boys |
More attention has been focused on the effects of radiation on spermatogenesis than on its effects on Leydig cell function [30]. The data available indicates that chemical changes in Leydig cell function are observable following direct testicular irradiation with the effect more pronounced with 2,400 cGy than with 1,200 cGy [71]. The severity of the effect is more marked the younger the patient at the time of radiotherapy [9]. In general, progression through puberty and testosterone production proceeds normally in males subjected to radiation therapy.
14.3 Clinical Manifestations
14.3.1 Effects of Chemotherapy
The extent and reversibility of cytotoxic damage generally depends on the agent and cumulative dose received, although significant individual variation has been observed consistently. The effects of alkylating agents on testicular function have been studied extensively.
Cyclophosphamide, either alone or in combination with other agents, is known to damage the germinal epithelium. Meta-analysis of 30 studies that examined gonadal function following various chemotherapy regimens noted that gonadal dysfunction correlated with total cumulative dose of cyclophosphamide; more than 300 mg/kg was associated with >80 % risk of gonadal dysfunction [61]. A study of men treated for pediatric solid tumors reported permanent azoospermia in 90 % of men treated with cyclophosphamide doses >7.5 g/m2 [78]. Other studies have also found that 7.5–9 g/m2 of cyclophosphamide is associated with a significant risk of testicular injury [4, 36].
Although tumor cytotoxicity data indicates that 1.1 g/m2 cyclophosphamide is approximately equivalent to 3.8 g/m2 ifosfamide [13], the relative gonadotoxic effect is not well known. In a recent series of male childhood survivors of osteosarcoma with a median age of 9 years (range 4–17 years) from completion of therapy, the incidence of azoospermia related to ifosfamide therapy (median 42 g/m2) versus no ifosfamide was statistically significant (P = 0.005). Six patients were normospermic: five received no ifosfamide and one received low-dose ifosfamide (24 g/m2). Fifteen of the 19 azoospermic patients received ifosfamide. Infertility in the others may have been related to cisplatin (560–630 mg/m2). One patient had oligospermia [45]. Recent studies support that doses of ifosfamide in excess of 60 mg/m2 are associated with a significant risk of infertility in male patients [34, 84].
Hodgkin’s disease (HD) patients treated with six or more courses of mechlorethamine, vincristine, procarbazine, and prednisone (MOPP) have also demonstrated permanent azoospermia attributable to both of the alkylating agents: mechlorethamine and procarbazine. Procarbazine appears to play a major role in this process. Hassel et al. studied testicular function after OPA/COMP (vincristine, prednisone, adriamycin/cyclophosphamide, vincristine, methotrexate, prednisone) chemotherapy without procarbazine in boys with HD. These patients showed no major testicular damage compared to boys who had received OPPA/COPP (includes procarbazine), again pointing out that procarbazine is a potent gonadotoxic agent [25]. Treatment of Hodgkin’s disease with combination chemotherapy regimens such as ChlVPP (chlorambucil, vinblastine, procarbazine, and prednisolone) or COPP (cyclophosphamide, vincristine, procarbazine, and prednisolone) have also been reported in a number of studies to result in permanent azoospermia in 99–100 % of patients treated with six to eight courses of these regimens [12, 28, 46]. After ChlVPP, FSH and LH were elevated in 89 % and 24 % respectively, with azoospermia in all seven patients tested. Charak and coworkers found azoospermia in all 92 patients following treatment with six or more cycles of COPP; 17 % of patients had been treated more than 10 years previously, suggesting that germinal epithelial failure is likely to be permanent [12]. CHOP (cyclophosphamide, doxorubicin, vincristine, prednisolone) or CHOP-like regimens such as those which are used for non-Hodgkin’s lymphoma (NHL) are generally less gonadotoxic than those used for HD, presumably due to the absence of procarbazine in the treatment for NHL (e.g., VAPEC-B, VACOP-B, MACOP) [51, 59]. Azoospermia occurring on therapy for NHL is likely to recover within the following year [58]. Efforts to reduce the risk of sterility after Hodgkin disease include the use of ABVD (adriamycin, bleomycin, vinblastine, dacarbazine – an effective combination that does not contain the alkylating agents chlorambucil or procarbazine) [40, 82] and other related regimens. Viviani and coworkers showed that while recovery of spermatogenesis after MOPP was rare, all who experienced oligospermia after ABVD recovered completely by 18 months [82]. Hybrid regimens (i.e., alternating cycles of ABVD with ChlVPP or MOPP) are also less gonadotoxic than MOPP, ChlVPP, or COPP given alone.
Nitrosoureas, used in the treatment of brain tumors in childhood, may also cause gonadal damage in boys [1, 44, 66]. In nine children treated for medulloblastoma with craniospinal radiation and a nitrosourea (carmustine or lomustine plus vincristine in four and procarbazine in three), there was clinical and biochemical evidence of gonadal damage. Specifically, these children presented with elevated serum FSH and small testes for the stage of pubertal development (compared with eight children similarly treated but without chemotherapy). The authors concluded that nitrosoureas were responsible for the gonadal damage, with procarbazine also contributing in the three children who received this drug.
PVB (cisplatin, vinblastine, and bleomycin) is standard chemotherapy with minimal effects on long-term testicular function that is used in patients with germ cell tumors. Such patients, however, can be affected by ejaculatory failure caused by damage to the thoracolumbar sympathetic plexus during retroperitoneal lymph node dissection and by preexisting germ cell defects. Hansen et al. found that for patients treated with orchiectomy or orchiectomy plus PVB, sperm production was similar 1.5 years after treatment. Approximately half in each group had sperm counts below the normal control reference level [24]. Lampe et al. analyzed data on 170 patients with testicular germ cell cancers who underwent treatment with either cisplatin- or carboplatin-based chemotherapy [41]. A median of 30 months after completion of chemotherapy, 54 (32 %) were azoospermic and 43 (25 %) were oligospermic. The probability of recovery to a normal sperm count was higher in men: (a) with a normal pretreatment sperm count, (b) men who received carboplatin rather than cisplatin-based therapy, and (c) men treated with less than five cycles of chemotherapy. Recovery continued for more than 2 years, with the calculated chance of spermatogenesis at 2 years being 48 %, and a calculated chance of spermatogenesis at 5 years being 80 % [31, 41].
Heyn and colleagues described the late effects of therapy on testicular function in patients aged 10 months to 19 years with paratesticular rhabdomyosarcoma as a result of cyclophosphamide, radiation, and retroperitoneal lymph node dissection. Tanner staging was normal in 45 patients for whom it was recorded. However, eight had loss of normal ejaculatory function. Elevated FSH values and/or azoospermia occurred in greater than half the patients where data was available. Testicular size was decreased in those who received cyclophosphamide or testicular irradiation [27].
The importance of alkylating agents in the induction of gonadal toxicity is noted by contrasting the above outcomes to those of children with acute lymphoblastic leukemia (ALL). In general, testicular function is normal in boys after chemotherapy for ALL. All 37 survivors of childhood ALL evaluated at two time points after the completion of treatment (median age 9.7 years and again 18.6 years later) completed pubertal development normally and had a testosterone concentration within the normal adult range [83]. Six men showed evidence of severe damage to the germ epithelium with azoospermia or elevated FSH; all of these patients had received cyclophosphamide as part of their chemotherapy regimen [83]. In contrast to alkylating agents, the classic antimetabolites used in the treatment of childhood ALL are not associated with long-term gonadal toxicity [30]. Both vincristine and corticosteroids can cause immediate inhibition of spermatogenesis; however, following the cessation of these agents, spermatogenesis recovery occurs [39].
14.3.1.1 Treatment-Induced Leydig Cell Failure from Chemotherapy
Leydig cells are much less vulnerable to damage from cancer therapy than germ cells, likely due to their slow rate of turnover [68]. For example, chemotherapy-induced Leydig cell failure resulting in androgen insufficiency and requiring testosterone-replacement therapy is rare. However, studies suggest that Leydig cell dysfunction may be observed following treatment with alkylator-based regiments. Specifically, raised plasma concentrations of LH combined with low levels of testosterone are the hallmarks of Leydig cell dysfunction. When Leydig cell dysfunction occurs prior to or during puberty, affected individuals will experience delayed and/or arrested pubertal maturation and lack of secondary sexual characteristics [70]. If the insult follows completion of normal pubertal development, observed symptoms include loss of libido, erectile dysfunction, decreased bone density, and decreased muscle mass [70]. Measurements of testosterone and gonadotropin concentrations are therefore warranted following chemotherapy treatment. Males with a raised LH concentration in the presence of a low testosterone levels should be considered for androgen replacement therapy.
14.3.2 Effects of Radiation
The data for gonadal function following fractionated radiotherapy in humans comes from patients with cancers who have been treated with either fields near the testes in which there is scatter dose or diseases such as testicular cancers or ALL in which the testes are thought to be at risk of harboring disease and irradiated directly. One of the potentially confounding issues is that some of these cancers may themselves be associated with decreased gonadal function independent of irradiation. For example, Hodgkin’s disease is well documented to cause oligospermia in some patients [29]. Patients with testicular tumors may have preexisting gonadal dysfunction [29]. In ALL, the leukemic cells may infiltrate into the interstitium of the testis and conceivably affect Leydig cell function.
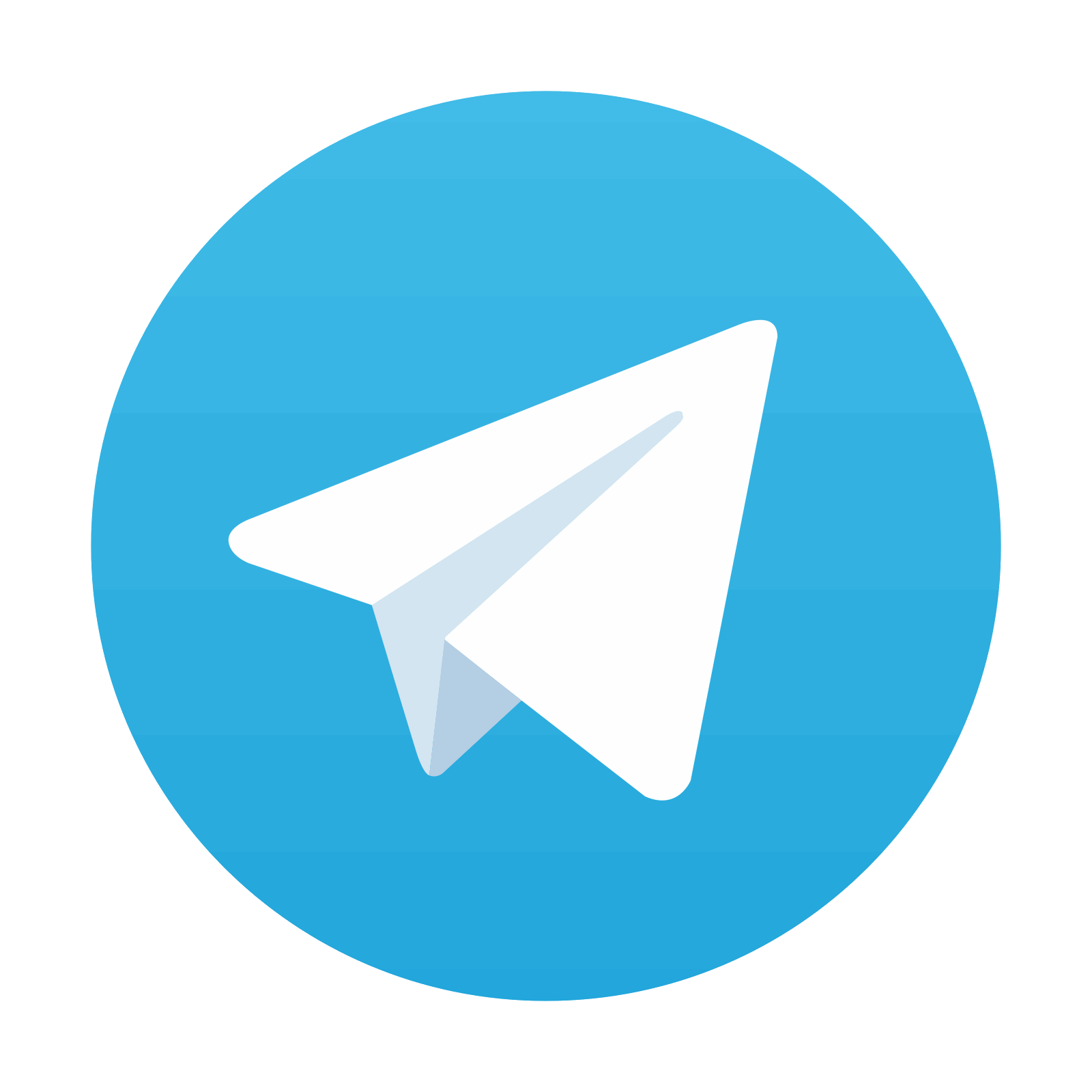
Stay updated, free articles. Join our Telegram channel

Full access? Get Clinical Tree
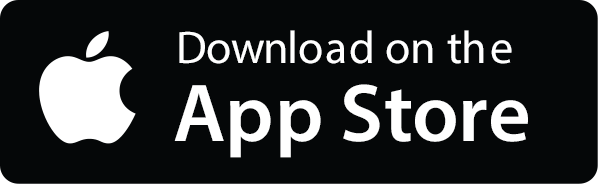
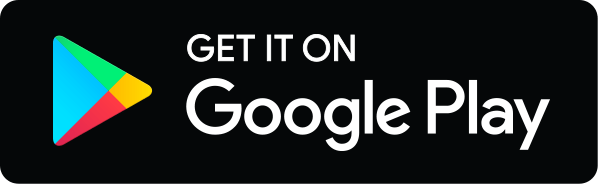