ARTHROPOD-BORNE VIRAL ENCEPHALITIDESM
TOM SOLOMON, ADJANIE PATABENDIGE, AND RICHARD J. WHITLEY
Conventionally, viruses are placed within families and genera according to the taxonomic system, based on morphologic, biochemical, antigenic, and genetic similarities. However, because the taxonomic system tells us nothing about how a virus is transmitted or about the patterns of disease it causes, it is of relatively little help to the practicing clinician. For this reason, alternative groupings that incorporate these clinical and epidemiologic aspects are often used. One such group is the arthropod-borne viral (or arboviral) encephalitides that includes viruses transmitted by arthropods (insect, ticks, sandflies, and biting midges) that are important causes of central nervous system (CNS) disease in humans. Altogether, there are more than 500 arthropod-borne viruses, or “arboviruses,” that come from four viral families (Togaviridae, Flaviviridae, Bunyaviridae, and Reoviridae); only a relatively small number of these viruses are responsible for human disease (Table 15.1). Within the family Togaviridae, the Alphavirus genus contains eastern, western, and Venezuelan equine encephalitis (EEE, WEE, and VEE) viruses—important causes of disease in horses and humans in the Americas—and chikungunya virus (CHIKV), which caused major outbreaks associated with severe morbidity in the Indian Ocean islands and in India during 2005 to 2007 (1). The flaviviruses (genus Flavivirus, family Flaviviridae) include Japanese encephalitis virus (JEV), which occurs across much of southern and eastern Asia and is the most important cause of epidemic encephalitis worldwide; West Nile virus (WNV), which has spread to cause large encephalitis outbreaks in the United States; dengue virus (DENV), which continue to be a global threat with over 100 million infected annually; and tick-borne encephalitis (TBE) viruses—important in Europe and Russia. Within the family Bunyaviridae, the Bunyavirus genus includes La Crosse virus (LACV) and other California serogroup viruses that cause encephalitis, whereas the Phlebovirus genus includes Toscana virus (TOSV), an important emerging cause of CNS disease in southern Europe. The Reoviridae family includes Colorado tick fever virus (CTFV) and similar members of the Coltivirus genus that causes febrile illness and CNS disease.
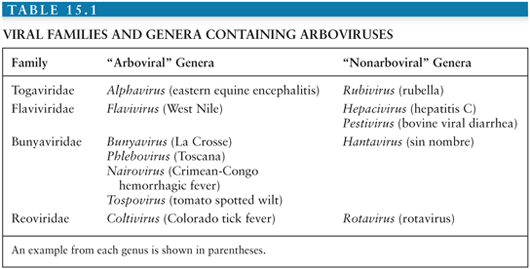
Arboviruses classically cause three disease patterns in humans (Fig. 15.1): fever-arthralgia-rash syndromes, viral hemorrhagic fevers (often associated with hepatitis), and neurologic disease, which are the focus of this chapter. They constitute some of the most important emerging and reemerging infectious diseases. General principles of arboviral ecology, epidemiology, and pathogenesis, as well as diagnosis, management, and prevention of arboviral encephalitis, are discussed before the more important diseases are considered in more detail.
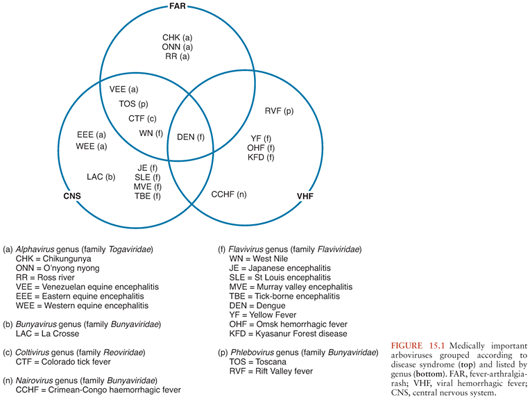
ECOLOGY OF ARBOVIRUSES
A basic understanding of the ecology of arboviruses is helpful in understanding the epidemiology of human disease. For most of the arboviruses that cause neurologic disease in humans, including many of the flaviviruses, alphaviruses, and bunyaviruses, humans are not part of the natural cycle, being incidental hosts (Fig. 15.2). In nature, these viruses are typically transmitted between birds or small rodents in enzootic cycles that use mosquitoes or ticks as vectors. To be evolutionarily successful, arboviruses require immunologically naive hosts, which is why most have evolved to use rapidly reproducing animals. Humans are infected when they encroach upon this natural enzootic cycle and are bitten by the vector. This may be because they live in or have entered areas where the virus circulates naturally or because changes in ecologic or environmental circumstances have caused the virus to move closer to areas inhabited by humans. Sometimes a different mosquito carries the virus to humans (a bridging vector), and sometimes there is an intermediate vertebrate cycle that brings the virus closer to humans (e.g., equines for some of the alphavirus encephalitides and swine for JEV). A large outbreak of disease in animals is an epizootic. Whereas natural hosts have prolonged and high viremia, humans have transient and low viremia, insufficient to transmit the virus further, and are considered “dead-end” hosts. Most human infections are asymptomatic or result in a mild nonspecific febrile illness, but in a small proportion, the virus enters the CNS to cause the syndrome for which the virus is known. The brief and low viremia makes diagnosis of human infection by virus isolation or nucleic acid amplification difficult, and for the most part, serologic tests are used. Although humans are “dead-end” hosts for most arboviruses, some arboviruses are better adapted to replicate in humans, resulting in persistent and high viremias, allowing for further transmission to a biting insect. Examples include VEEV, TOSV, CTFV, and DENV. These high viremias typically cause a characteristic arboviral “fever-arthralgia-rash” syndrome in most patients; CNS manifestations tend to be milder, for example, aseptic meningitis. Because of the high viremias, virus isolation and polymerase chain reaction (PCR) are more successful for these viruses.
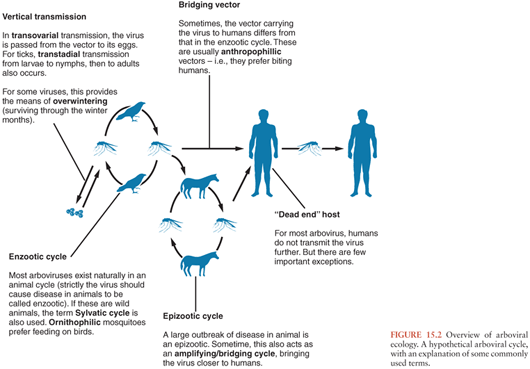
Arboviruses replicate in both the vertebrate host and the vector in an “extrinsic” cycle. Whereas vertebrate hosts typically mount an immune response and clear the virus, the arthropod vectors are usually infected for life. Virus is ingested by the vector with an infected blood meal, replicates in the epithelium of the mesenteron (midgut), then disseminates to the salivary glands, where it replicates further and is excreted with each blood meal. The virus may also be passed on from the adult mosquitoes and ticks to their offspring, via transovarial transmission (Fig. 15.2). For ticks, virus can also be transmitted vertically from the larval to nymph then adult stages (transtadial transmission). Vertical transmission is an important means of overwintering (i.e., surviving the cool winter months) for some viruses, but for others, the means of overwintering is uncertain. An exception to a “typical” arboviral cycle includes mechanical transmission via the vector’s mouth parts. More importantly, some viruses, as well as being arthropod borne, can also be directly transmitted between vertebrate hosts, bypassing the arthropod vector, for example, humans can become infected with Rift Valley fever virus (RVFV) from the body fluids of infected animals (2), TBE virus can be transmitted to humans via infected goat’s milk (3), and WNV can be transmitted in infected blood products or transplanted organs (4).
PATHOGENESIS OF ARBOVIRAL ENCEPHALITIS
Despite the wide range of arboviruses that cause encephalitis, there are many similarities in the pathogenesis and pathology (see Table 15.2 for an overview of the arboviral encephalitides). Much of the data has come from rodent models of alphavirus encephalitis. Following inoculation, usually during the bite of a blood-sucking mosquito or tick, arboviruses typically replicate in the skin and local lymph nodes, causing a viremia, before entering the CNS (5). Langerhans cells in the skin have been implicated as a site of replication for alphaviruses and some flaviviruses (6–8). Keratinocytes have also been shown to be one of the initial targets for WNV replication and could be contributing to the persistence of WNV in the skin (9). Replication in local skeletal muscle may also be important for alphaviruses. Invasion of target organs appears to depend in part on the extent of viremia, but also on other invasive viral characteristics (10). The two peaks of the characteristic saddleback or biphasic fever curve seen in many arboviral infections correspond to production of interferon (IFN) and proinflammatory cytokines during the initial viremia, and then a mononuclear inflammatory response in infected target organs (11). IFN production is induced when viral components are detected by cytoplasmic proteins such as retinoic acid-inducible gene I (RIG-I) and melanoma differentiation-associated gene 5 (MDA5) during the unwrapping of the viral RNA from the nucleocapsid to allow replication. In addition, cells use toll-like receptors (TLRs) to recognize pathogen-associated molecular patterns. TLR3, TLR7, TLR8, and TLR9 are the main viral sensors in humans. TLR7, TLR8, and TLR9 are localized in the endosomal compartments and signal through the MyD88 adapter, leading to type 1 IFNs and inflammatory cytokine production. TLR3 is located both on the cell surface and in endosomal compartments and induces production of type 1 IFNs through toll/interleukin-1 (IL-1) receptor (TIR) domain-containing adapter-inducing IFN-β (TRIF) adapter. Protein kinase R (PKR) and 2′, 5′-oligoadenylate synthetase (OAS) are two other cellular viral sensors. Viral sensors activate IFN regulatory factors and transcriptional induction of IFNs, which then signal through the JAK/STAT pathway leading to transcription of many interferon-stimulated genes (ISGs). The expression of ISGs and induction of their products (e.g., RIG-I, MDA5, TLRs, OAS, PKR) are critical for limiting replication of arboviruses. Many arboviruses encode nonstructural proteins to counter the host immune response. The main function of these nonstructural proteins is to antagonize the initial IFN response and is an important adaptation that has helped arboviruses to establish themselves in numerous vertebrate hosts (12).
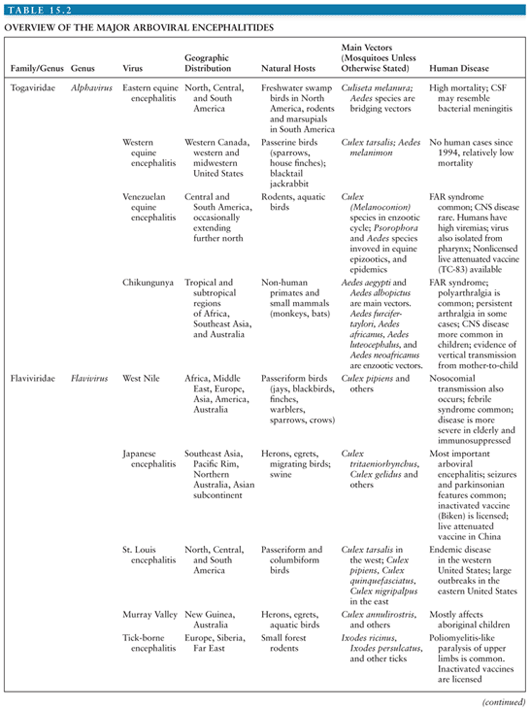
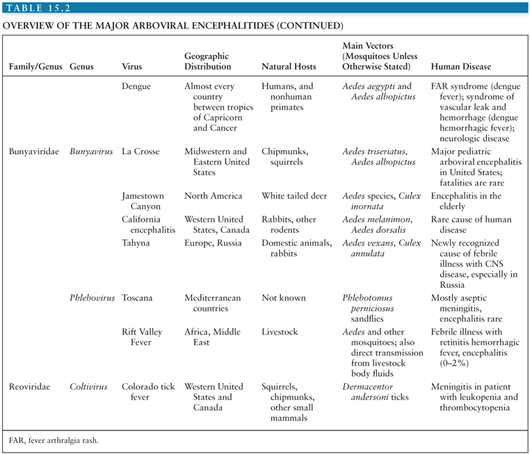
The mechanisms of virus entering the CNS may include infection of or transport across brain microvascular endothelial cells that form the blood–brain barrier (BBB) and infection of olfactory neurons or of choroid plexus epithelial cells (13–16). However, the exact mechanisms of virus entry into the CNS across the BBB and interactions of the virus with the BBB remain incompletely understood, despite the critical role of the BBB in preventing pathogen entry to the CNS (17–20). In animal models, the term neuroinvasiveness is used to describe a virus’ ability to enter the nervous system following peripheral inoculation. “Neurovirulence” describes its ability to cause damage once within the CNS (e.g., following invasion from the periphery or following direct intracranial inoculation). Once inside the nervous system, the pathogenesis may involve a combination of infection and dysfunction of neurons, caused by direct viral or indirect inflammatory damage and other mechanisms such as programmed cell death. The immune response to alphavirus infection has been characterized in experimental animals and includes an early innate response with type I interferon (IFN-α and IFN-β) production, followed by specific humoral and cellular immune responses (21). Experiments with knockout mice have shown type I IFN limits early viral replication, possibly by production of MxA protein, a large cytoplasmic guanosine triphosphatase that prevents accumulation of viral genomic and subgenomic RNA (22). Tumor necrosis factor-α (TNF-α) and other proinflammatory cytokines are produced, contributing to the pathogenesis (23). Production of IFN-α and other cytokines and chemokines has been implicated in JEV in humans (24–26).
The humoral immune response in human alphavirus and flavivirus encephalitis includes early production of immunoglobulin M (IgM), followed by immunoglobulin G (IgG) that persists for years (27–30). The failure of antibody production is associated with a fatal outcome in human disease (30–32). Antibody appears to be protective by neutralization, nonneutralizing binding, and complement-mediated lysis of infected cells (33). In animal models of alphavirus encephalitis, antibody assists with nonlytic clearance of virally infected cells by a mechanism that appears to be synergistic with the effects of IFN-α (34). This may be especially important for clearing virus from the CNS, where limited expression of major histocompatibility class (MHC) antigens in mice (or human leukocyte antigen [HLA] antigens in humans) may restrict the role of T lymphocytes. Infiltration into the CNS of antibody-producing B cells has been demonstrated in animal models (35). Cellular immune responses to alphavirus infection include lymphoproliferative, cytokine, and cytotoxic. In alphavirus encephalitis, there is infiltration of natural killer cells, B cells, CD4, CD8 T cells, and macrophages. CD8 T cells may be important in virus clearance from infected macrophages (36). Studies on humoral responses to flavivirus infection have shown protection of mice by passive transfer of antibodies against lethal challenge by several flaviviruses (37–39). Furthermore, B cell–deficient mice were shown to be highly susceptible to flavivirus infection (37,40–42). In contrast, the role of T cells during flavivirus infection is less well characterized. A few studies have shown a protective role for CD4+ T cells by enhancing antibody production and sustaining flavivirus-specific CD8+ T-cell responses (43,44). CD8+ T cells have been shown to be important for clearance of virus and survival of mouse models of WNV (45–48), whereas cytotoxic T cells caused accelerated and more severe pathogenesis in mice infected with MVEV (49). In mice infected with JEV, CD8+ T cells did not affect the outcome of the infection. Therefore, a conflicting role for CD8+ T cells in flavivirus encephalitis is suggested (40).
PATHOLOGY OF ARBOVIRAL ENCEPHALITIS
Few data are available for CNS infections caused by coltiviruses and phleboviruses because they are rarely fatal. Pathologic studies of fatal human encephalitis caused by alphavirus, flavivirus, and bunyaviruses show many common findings (50–56). The leptomeninges are normal or slightly hazy, and histologic examination shows an inflammatory infiltrate. The brain parenchyma is congested with focal petechiae or hemorrhage. Lesions are distributed through the gray matter of the cerebral cortex, midbrain, basal ganglia, cerebellum, brainstem, and spinal cord, providing the anatomic correlates for many of the clinical features seen (see later discussion). Microscopically, there is perivascular cuffing, with infiltration of mononuclear and polymorphonuclear cells into the parenchyma, as well as neuronophagia of infected cells by glial cells. Immunohistochemical analysis of the lymphocyte populations typically shows CD4+ and CD8+ cells, which are presumed to be cytotoxic T cells. Similar changes are seen in animal models. Initially, there is perivascular infiltration of mononuclear cells, with a few polymorphonuclear cells, which is accompanied by perivascular extravasation of red blood cells (RBCs), endothelial cell swelling, and hyperplasia (57,58). This is followed by migration of lymphocytes and monocytes toward virus-infected neurons. The inflammation is accompanied by gliosis and by inflammatory and glial cell apoptosis (59). Demyelination is seen in encephalitis caused by some alphaviruses but does not seem to be important in the flaviviruses (60). Although the cellular immunity and the inflammatory response are important in most infections, some patients die from virally induced neuronal cell death before there is evidence of a cellular immune response and inflammation.
Various processes contribute to neuronal cell death including apoptosis, cytoplasmic swelling, vacuolation, and membrane breakdown (61). In some instances, alphaviruses are inadequately cleared from the CNS, as illustrated by detection of viral RNA in the brains of mice long after recovery (62,63). Chronic progressive human disease with continuing brain inflammation long after the acute disease has been reported in some alphavirus encephalitides (64). For example, persistent symptoms such as joint and muscle pains can last for several years post CHIKV infection (1). Chronic infection does not appear to be important for most of the mosquito-borne flaviviruses, but there is evidence of persistent infection and chronic inflammation for humans infected with TBEV and in animal models (65,66).
CLINICAL FEATURES OF ARBOVIRAL ENCEPHALITIS
The clinical syndrome following neurologic infection with an arbovirus depends on the site of attack. If the meninges are involved, meningitis results; inflammation of the brain parenchyma causes encephalitis; and damage in the spinal cord causes myelitis. The term meningoencephalomyelitis encompasses the concept that all three components may be affected. Despite the wide range of etiologic agents, there are many similarities in the clinical presentations of arboviral encephalitis. After a brief incubation period, which typically is 4 to 8 days but may range from 1 to 28 days, patients develop a nonspecific flulike illness with fever, headache, nausea, and vomiting. Often, there are upper respiratory tract symptoms, such as cough and sore throat, or abdominal pain and diarrhea. Sometimes, a rash, conjunctival or pharyngeal injection, or lymphadenopathy may give a clue to the diagnosis. This may be seen for the “fever-arthralgia-rash” viruses, for example, WNV, DENV, CTF, TOSV, or VEEV. The febrile phase usually lasts a few days and may immediately be followed by continued fever with the development of neurologic symptoms and signs, or there may be an intervening asymptomatic period, thus giving a “saddleback” or biphasic fever curve characteristic of some arboviral infections, such as TBE or CTF. Neurologic disease may manifest as subtle changes in behavior, or mutism, resulting in an initial misdiagnosis of psychiatric illness. More often though, there is a profound reduction in consciousness level, which in children may be heralded by seizures. Seizures and status epilepticus are especially common in children with JEV and LACV encephalitis (67,68). As well as obvious generalized tonic-clonic seizures, subtle motor status epilepticus is being increasingly recognized. A great range of focal neurologic signs may be seen in arboviral encephalitis, including those of upper motor neuron damage, such as a hemiparesis, and cranial nerve signs indicative of brainstem damage. Flaccid limb weakness due to destruction of the anterior horn cells in the spinal cord is seen in flavivirus encephalitis and EEEV. Most flaviviruses can also cause a poliomyelitis-like flaccid paralysis that typically affects the lower limbs for mosquito-borne flaviviruses such as JEV, WNV, and Murray Valley encephalitis (MVE), and the upper limbs for TBE (69–71). Extrapyramidal signs are common in both alphavirus and flavivirus encephalitis and are thought to be the clinical correlates of the basal ganglia damage evident pathologically and radiologically, including increased tone causing generalized axial and limb rigidity, or rigidity/spasm such as opisthotonus, and tremors of the limbs, eyelids, and tongue.
DIFFERENTIAL DIAGNOSIS OF ARBOVIRAL ENCEPHALITIS
The differential diagnosis of arboviral encephalitis is broad and includes other causes of viral encephalitis (Table 15.3), as well as diseases that mimic viral encephalitis (Table 15.4). Certain epidemiologic features may give a clue that a patient has an arboviral infection. For example, in parts of Asia, epidemics of JEV occur with great predictability after the start of the rainy season. In the Americas, the alphavirus equine encephalitides are typically associated with illness in horses. More recently, birds falling from the sky have heralded the arrival of WNV. The age and occupation of the patient can also point to epidemiologic risk factors. JEV tends to affect children in rural parts of Asia, recent WNV encephalitis outbreaks have affected the elderly or patients on immunosuppressive drugs, and LACV encephalitis tends to affect children. A recent tick bite may suggest TBE in Europe, Russia, and the Far East, or CTF in parts of North America, though other tick-borne diseases, such as borreliosis, ehrlichiosis, and rickettsial disease must be considered. A biphasic illness is characteristic of some arboviral encephalitides. On examination, certain neurologic signs may suggest a patient has arboviral encephalitis rather than encephalitis caused by herpes or other viruses. For example, in an unconscious patient, the presence of flaccid limbs consistent with anterior horn cell damage, or the tremors, increased tone, and rigidity spasms suggestive of basal ganglia damage may suggest an arboviral cause.
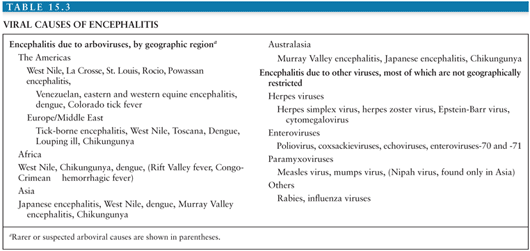
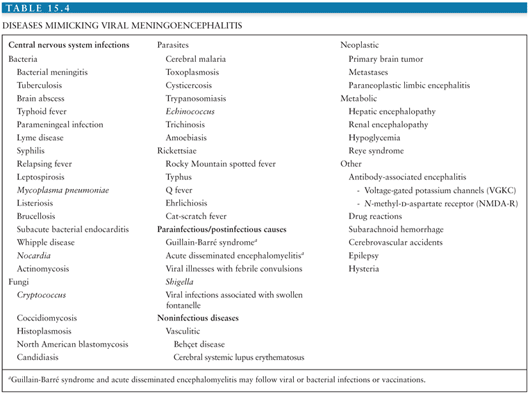
INVESTIGATION AND DIAGNOSIS OF ARBOVIRAL ENCEPHALITIS
A mild leukocytosis is common in many arboviral encephalitides, but leukopenia with thrombocytopenia is sometimes seen in TBE and CTF. Hyponatremia due to a syndrome of inappropriate antidiuretic hormone (SIADH) secretion is common, and in some infections, alanine transaminase may be mildly elevated. In Western settings, lumbar puncture is often delayed in severely ill patients until imaging has ruled out a space-occupying lesion, or incipient brainstem herniation. In many tropical settings where imaging is not available, lumbar punctures are performed on most encephalopathic patients who do not have signs of brainstem damage because of the importance of excluding other treatable causes, particularly bacterial infections (72,73). Lumbar puncture will often reveal mildly elevated cerebrospinal fluid (CSF) opening pressures (up to 250 mm of CSF), a lymphocytic pleocytosis with a normal CSF-to-plasma glucose ratio, and slightly elevated protein level. However, an early lumbar puncture may reveal no cells, or a neutrophil predominance, rather than a lymphocytic CSF. A low glucose ratio has been reported for EEEV and CTF, whereas an elevated ratio has been reported for VEEVs. Radiologic investigations may be helpful in excluding other conditions and in highlighting changes such as the high signal intensity seen on magnetic resonance imaging (MRI) in the thalamus and basal ganglia that characterizes both alphavirus and flavivirus encephalitis (74). Computed tomography (CT) scans may show low signal in the same areas or just edema. Although they do not reveal diagnostic information, electroencephalograms (EEGs) can help identify seizures (particularly the subtle motor seizures described earlier) and distinguish focal seizures from tremors.
For most arboviral infections of humans, viremias are so low and brief that they are undetectable by the time a patient comes to the hospital (75) (Fig. 15.3); exceptions are the viruses for which humans are important natural or amplifying hosts, such as DENV, VEEV, and TOSV. Virus may also sometimes be isolated from the CSF, particularly for infections that prove fatal, or from brain tissue at either biopsy or autopsy. The traditional method for virus isolation was inoculation into suckling mice, but this has largely been replaced by continuous cell lines (e.g., C6/36 cells derived from Aedes albopictus mosquitoes, and Vero cells derived from African green monkey kidney). Amplification by PCR of viral nucleic acid in the CSF or serum tends to be more sensitive than virus isolation, particularly if real-time Taq-Man PCR is used and has helped with diagnosis of WNV and TOSV infections, although false-positive results do occur. Immunohistochemistry of brain tissue may demonstrate viral antigen in fatal cases, and antigen-capture enzyme-linked immunosorbent assays (ELISAs) have been developed, but they have limited utility.
Serologic methods to detect antiviral antibody are the mainstay of the diagnosis of arboviral infections. The traditional techniques such as hemagglutination inhibition, complement fixation, indirect immunofluorescence, and neutralization tests require the demonstration of a greater than fourfold rise in antibody titer in samples taken several weeks apart and thus have practical limitations; for example, they do not give a diagnosis acutely and are not helpful in patients who die or are lost to follow-up. However, they do have specific research uses. For example, hemagglutinating antibodies are often broadly cross reactive, so in a patient with an undiagnosed encephalitis, a panel of antigens can be used to indicate whether a patient was infected with a flavivirus, an alphavirus, a bunyavirus, or other virus; this can then be followed by more specific tests within that group. Neutralizing antibodies persist for life, so they can be used for serologic surveys to indicate whether individuals have ever been infected with a virus. In recent years, IgM and IgG capture ELISAs have become the most useful and widely deployed tests for diagnosing arboviral encephalitis. Depending on the virus, typically 50% of patients have antibody on admission to hospital, and more than 90% have antibody 1 week later, so in patients whose initial test results are negative, the test should be repeated after 1 week (Fig. 15.3). Although they are the most useful tests, ELISAs too have their limitations. For example, because closely related viruses have many shared epitopes, there may be cross reactivity in IgM and IgG capture ELISAs between the viruses. This problem can usually be overcome by testing for the related viruses in parallel, with the strongest reaction indicating the infecting agent. However, sometimes a neutralization test, which is more specific, may be needed. Importantly, antibody will be detected in the serum of individuals who have had recent asymptomatic or febrile infection but no neurologic disease. Its detection in the CSF is usually taken as a better indicator of viral replication in the CNS. Finally, some patients may make antibody late or not at all. These patients are more likely to die and to have virus detected by another means such as isolation or PCR.
Newer approaches for detection of flaviviruses antibody include the lateral flow assay and the microsphere immunoassay (MIA). The lateral flow device was recently approved by the U.S Food and Drug Administration (FDA) for the diagnosis of WNV infection in humans, which can give a result in 15 minutes (76). In MIA, an antigen is attached to encoded microbeads that will bind the target analytes during the assay procedure. These can then be identified using a fluorescence-activated cell sorting system (FACS). MIA is used by many laboratories in the United States for WNV diagnosis as it is highly sensitive compared to a standard ELISA. Furthermore, one serum sample can be analyzed for reactivity to several antigens in a single assay (77). Other approaches include high-throughput rapid microneutralization assays, biosensor-based assays, microfluidic systems based on virus-coated magnetic beads, and multiplex real-time RT-PCR assays (78–81).
TREATMENT OF ARBOVIRAL ENCEPHALITIS
There are no established antiviral drugs for any arboviral encephalitides. IFN-α, ribavirin, and intravenous immunoglobulins have been given to patients with flavivirus encephalitis, based on in vitro and animal data. However, the few clinical data available suggest that IFN-α is not effective in JEV (82). In the West, most patients with encephalitis will be given acyclovir until herpes simplex virus type 1 (HSV-1) encephalitis has been ruled out. The management of arboviral encephalitis focuses on treating the complications of disease, in particular hyponatremia, seizures, and increased intracranial pressure (ICP). In most Western settings, severely ill patients will be electively ventilated and managed in intensive care settings, allowing airway protection, seizure control, optimal assessment of fluid balance, and hyperventilation to reduce increased ICP. However, in many of the settings in which arboviral encephalitis occurs, this is not possible. At a minimum, patients at risk of raised ICP should be nursed at 30°C, with the neck held straight to ensure the jugular venous outflow is not impaired and with urine output monitored and oxygen given.
Seizures are common, particularly in children with flavivirus and bunyavirus infections. In addition to obvious tonic-clonic seizures, subtle motor seizures may be indicative of subtle motor status epilepticus (68). An EEG is advisable, particularly in patients with twitching of a finger, lip, or eyelid. Simple or complicated seizures should be managed according to local standard of care (83–85) (see Chapter 1 where the management of the critically ill patient with CNS infections is discussed). A few specific notes relating to arboviral encephalitis are indicated. Attention to the etiology of increased ICP is of the greatest importance (86,87). In many encephalopathies, if the blood pressure is well maintained, but increased ICP is suspected, 20% mannitol (0.5 to 1.0 g/kg) is given, although the little evidence available suggests the benefits, if any, are only short term (68,88). Steroids are sometimes given, although they were not shown to be beneficial in JEV (89). Hypoglycemia is common in many tropical pediatric conditions (90) and must be looked for and corrected.
PREVENTION OF ARBOVIRAL ENCEPHALITIS
A consideration of the typical arboviral ecologic cycle (Fig. 15.1) reveals points at which intervention might be effective. These can be divided into measures to interrupt the natural cycle, measures to stop humans being bitten by infected vectors, and measures to stop infection resulting in disease (i.e., vaccination). For most of the arboviruses that cause encephalitis, because the natural cycle involves small wild birds and rodents, interrupting this cycle is not possible. However, when an intermediate or amplifying vertebrate host brings the virus closer to humans, for example, equines for VEEV and swine for JEV, measures can be taken. For example, vaccines that protect horses against VEE probably also reduce the number of human cases. Immunization of swine against JEV has been practiced in Asia, though with limited success. However, relocation of swine away from homes is probably a more effective way of reducing transmission of JEV to humans. Surveillance of mosquitoes, dead birds, and sentinel chickens (i.e., chickens deliberately exposed to mosquito bites) for viral infection is used in many Western settings as an indicator of viral activity and possible impending human disease, and antimosquito measures are then implemented. Interventions to reduce the number of circulating mosquitoes depend on the species and the setting. Measures include removing stagnant water from swampy areas, ditches, and drains favored by many Culex species and removing the freshwater containers (car tires, tree holes, litter) favored by Aedes species. Larvicides can also be applied to potential breeding sites (either biologic larvicides such as Bacillus thuringiensis variance israelensis or the chemical larvicide, metoprine). With continuing human cases, public health authorities have practiced ground or aerosol spraying with pyrethroid formulations that kill adult mosquitoes. Newer interventions are under development including the sterile insect technique and the Wolbachia-based strategies to control Aedes species (91–94).
For many of these measures, evidence of beneficial impact is limited, but they demonstrate that public health authorities are taking the situation seriously. Personal measures to reduce the chance of being bitten by infected vectors are important. These include using repellents that contain 10% to 30% DEET (N, N-diethyl-3-methyl benzamide) on skin and clothing, applying permethrin insecticides on clothes, and wearing long-sleeved shirts and trouser. In areas where tick-borne viruses circulate, regular inspection of the limbs ensures no ticks are attached. Many of the Culex vectors bite at night, and the use of impregnated bed nets is recommended. Public education is an important part of any of these measures. For some flaviviruses (e.g., JEV and TBEV), inactivated or live attenuated vaccines are available, although their use is limited by cost and availability. The dramatic reduction in TBE in Austria following widespread vaccination demonstrates feasibility. However, because most of these viruses are enzootic, eradication by vaccinating humans is not possible. For other arboviral diseases, the sporadic nature and low incidence mean that the vaccines would not be economically viable, even if they existed.
ALPHAVIRUSES
The alphavirus genus is one of two important genera in the family Togaviridae (named after the cloak, or toga that envelops the viruses) (11). Whereas alphaviruses are arthropod-borne members of the other genus, the Rubivirus genus organisms (e.g., rubella virus) are not arthropod borne and so are not considered further here (Table 15.1). Alphaviruses cause two clinical syndromes. In Africa, Old World alphaviruses CHIKV and o’nyong-nyong virus cause large outbreaks of fever arthralgia and rash; a similar syndrome in which arthritis is predominant is caused by Ross River virus in Australia. In the Americas, New World alphaviruses EEE, WEE, and VEE viruses cause outbreaks of encephalitis in horses and humans and are the focus of this section (Table 15.2). EEE is notable for having a higher case-fatality rate than other viruses in the group, whereas WEE is remarkable because although it has caused large outbreaks in the past, it has now virtually disappeared as a disease of humans. Although VEEV causes encephalitis in equines, in humans it causes a febrile disease, with only a small proportion developing usually mild CNS disease. Two other alphaviruses, Sindbis virus and Semliki Forest virus, have animal models of the pathogenesis of alphavirus encephalitis. Phylogenetic analyses suggest that the alphaviruses arose in the New World with subsequent introductions into the Old World. The WEE lineage appears to have derived as a recombinant of an ancestral EEE-like and Sindbis-like viruses (95).
Infectious Agent
Alphaviruses consist of a single strand of positive-sense RNA wrapped in a nucleocapsid and surrounded by a glycoprotein-containing lipid membrane that is derived from host cell plasma membrane (11). The virion is 60 to 70 nm in diameter. The 11to 12-kb RNA has a 5′ cap and a 3′ polyadenylated tail. It consists of message-sense RNA that is directly infectious. It codes from the 5′ to the 3′ end for four nonstructural proteins, nsP1, nsP2, nsP3, and nsP4, and five structural proteins—capsid (C), envelope 3 (E3), E2, 6K (a 6-kd protein), and E1. The nonstructural proteins are involved in replication of the viral RNA and production of subgenomic RNA. The C protein has a conserved end with many basic amino acids and is presumed to bind the viral genomic RNA to form the nucleocapsid. This nucleocapsid is an icosahedron with T = 4 symmetry. The E1 and E2 glycoproteins project from the lipid membrane, forming heterodimers that group as trimers to form 80 knobs on the virion surface (96). Alphaviruses gain entry into cells by attaching to the cell surface and entering via the endocytic pathway to form vesicles. As the pH level of the vesicles decreases, the E1/R2 heterodimer dissociates, revealing a highly conserved hydrophobic sequence in E1. This is thought to act as a fusion peptide, causing viral membrane and host cell membrane to fuse, and thus releasing the viral nucleocapsid into the host cytoplasm. Here the nucleocapsid is uncoated of its capsid protein so that naked RNA is exposed to ribosomes for initiation of translation as the first step of viral replication. Virus is replicated in association with the endoplasmic reticulum and Golgi apparatus, and new virions are released by budding at the host cell surface.
Eastern Equine Encephalitis
Epidemiology
EEE virus was first isolated from the brain of a horse that died during an epizootic of equine encephalitis in New Jersey and Virginia in 1933 (97). The first human isolate was made 5 years later. The geographical area affected by EEE extends from Ontario and Quebec provinces in Canada, down the eastern seaboard of the United States, and into South America as far as Argentina. To the West, the area extends as far as Wisconsin and eastern Texas. The virus is transmitted between wading birds, passerines, and other swamp birds by Culiseta melanura and other mosquitoes. It has also been isolated from crows (98). These mosquitoes are strictly ornithophilic (bird biting). However, other mosquitoes that feed on both birds and mammals serve as bridging vectors carrying the virus to horses, humans, and other mammals (Fig. 15.2, Table 15.2). In South America, rodents and marsupials may be more important natural hosts than birds. Outbreaks of disease have also occurred in commercial flocks of pheasants, partridges, turkeys, and emus (99). In these circumstances, direct transmission between birds because of preening and pecking and transmission via the fecal-oral route have also occurred. The means by which the virus persists during the winter is not known, but persisting viremia in birds or vertical transmission into mosquito eggs has been postulated. Cases occur year round but are more likely in the summer, especially when associated with increased rainfall, which by creating a high water table augments the breeding habitat of C. melanura.
EEE usually occurs as single cases and rarely as clusters that may be preceded by disease in horses. The median annual number of cases in the United States is three (range, 1 to 14), and the reported incidence is less than 0.1 to 0.4 per 107. Seroepidemiologic studies show that humans are only rarely infected with the virus. Fewer than 7% of residents older than 45 years had antibody in one study (100). Most infected patients are asymptomatic with a ratio of symptomatic to asymptomatic infections of about 1 in 30. However, the elderly and young children are more likely to develop disease.
Clinical Features
After an incubation period, which is thought to range from 3 to 10 days, patients present with a few days of febrile prodrome and then neurologic disease. Early symptoms include headache, myalgia, photophobia, abnormal sensations, vomiting, dizziness, and lethargy, followed by neck stiffness, a reduced level of consciousness, and seizures. In infants, progression to coma may be more rapid, occurring over 48 hours. On examination, a high pyrexia (>39°C) is common and one third of patients have signs of meningismus. Clinical signs suggestive of brainstem involvement include gaze deviation, nystagmus, and papillary abnormalities. This may be because of inflammatory lesions in the brainstem, but uncal and subtentorial herniation have been seen at autopsy. Hemiparesis and limb spasticity suggest upper motor neuron involvement, whereas flaccid limbs suggest involvement of lower motor neurons in the spinal cord. Seizures may be generalized or focal. In patients who remain conscious, aphasia and emotional lability may occur. Limb dysesthesia and flaccid paralysis have also been reported in a fully conscious patient, suggesting myelitis may occur without brain involvement (101). Approximately one third of patients die, but for those older than 60 years, the proportion is 50%. One third of survivors have moderate or severe sequelae (102).
Diagnosis
Peripheral leukocytosis, with neutrophil predominance can occur. The CSF resembles that of acute bacterial meningitis with a neutrophil pleocytosis (as high as 5,000 cells/mm3); the RBC count and protein level are often elevated, and the glucose ratio is less than 50% in half of the patients (102,103). CT scans show diffuse cerebral edema in most patients. On MRI, lesions that do not enhance with gadolinium are seen in the basal ganglia, thalamus, and brainstem (102). EEGs show background and focal slowing, as well as burst suppression. High-voltage delta waves carry a poor prognosis (103).
Virus isolation from the blood is unusual, although it has been reported for EEE early in the illness (101). In fatal cases, virus may be isolated from brain tissue or detected by PCR or immunohistochemical staining. In most cases, the diagnosis is made serologically by detecting antibody in the blood. Older serologic tests such as hemagglutination inhibition, complement fixation, and neutralization tests are being replaced by IgM capture ELISAs, which detect antibody in a single blood or CSF sample, thus providing an earlier diagnosis.
Treatment and Prevention
Because there are no antiviral drugs, treatment is focused on the complications of infection, particularly seizures and raised ICP and pneumonia. No vaccine against EEE is commercially available, although an inactivated vaccine is used by laboratory workers and others at high risk (104). Recently, a chimeric Sindbis (SINV)/EEEV candidates have been shown to be safe and effective against protecting mice against EEEV (105). A similar vaccine is used to protect horses. Personal protective measures against mosquito bites are described earlier in this chapter.
Western Equine Encephalitis
Epidemiology
The isolation of WEE virus from the brain of horses during an epizootic episode of unknown etiology in 1930 in the San Joaquin Valley of California marked the first time an arbovirus was isolated in the territorial United States (106). Subsequently, in 1938, WEE virus was obtained from a child who succumbed to encephalitis (107). Virus activity has been reported from western Canada, the western and midwestern United States, and as far south as Argentina. In the western United States, WEE virus is transmitted between passerine birds (sparrows and house finches) by Culex tarsalis mosquitoes. Epidemic and epizootic activity occurs mainly in the summer months. Highlands J virus, which is found in Florida, is one of several related viruses in the WEE complex that do not appear to cause disease in humans (108).
Clinical Epidemiology
Until recently, exposure to WEE virus was common, with up to 20% of humans having antibodies in some areas. However, a decline in the rural population and changes in land use have meant that even in areas where the virus circulates, this figure is now less than 1% (109). The largest outbreak was in 1941, with more than 3,000 human cases and hundreds of thousands of equine cases (110) and an estimated incidence of 167 per 100,000 in North Dakota, but since the 1990s, there have been only a handful of reported cases each year. The ratio of apparent to inapparent infections is estimated to be fewer than 1 in 1,000 in adults and 1 in 60 in children.
Clinical Features
The clinical features of WEE tend to be milder than those of EEE. After an incubation period, which ranges from 2 to 10 days, there is a nonspecific febrile prodrome before neurologic disease. Meningismus is seen in half the patients, and although weakness and tremors are common, fewer than 10% of patients develop coma (111). Focal signs include cranial nerve palsies, hemipareses, hyporeflexia, spasticity, generalized rigidity, and occasionally opisthotonus. The overall case-fatality rate is approximately 4% to 10% (112), being higher among the elderly. WEE virus is more severe in infants, with rapid progression from nonspecific illness to convulsions and coma. Transplacental infection has been reported (113). The overall case-fatality rate is 3%, increasing to 8% for those older than 50 years (114). Neurologic sequelae are common in young children. Parkinsonian features including cogwheel rigidity and tremors have also been reported (115).
Diagnosis
The peripheral white blood cell count is usually normal or mildly elevated. Hyponatremia due to SIADH has been reported. CSF opening pressures of more than 200 mm occur in two thirds of patients. CSF white blood cell counts are usually less than 100 cells/mm3 but may range from less than 10 to 500 cells/mm3 with lymphocyte predominance, a slightly elevated protein level, and a normal glucose ratio. CT scans have been reported as normal, but EEGs show diffuse slowing with focal delta activity in the temporal region, which may mimic HSV-1 encephalitis (116). WEE virus is occasionally isolated from the CSF or from diagnostic brain biopsies (117). Detection of IgM in the CSF or serum is the preferred diagnostic test (30).
Treatment and Prevention
There is no specific treatment, although equine immune serum was used following laboratory exposure in one patient (118). A nonlicensed inactivated vaccine is available for laboratory workers and others at high risk (119). A recent study has shown the potential of an adenovirus-vectored WEEV vaccine, Ad5-WEEV, for development into an emergency vaccine during an outbreak of WEEV (120). Furthermore, a human adenoviral vector (Ad5-mIFNα) expressing mouse IFN-α was shown to offer 100% protection against mice challenged with various WEEV strains (121). Surveillance and vector control measures are practiced in some areas where the virus circulates.
Venezuelan Equine Encephalitis
Epidemiology
VEEV was first isolated from the brains of dead horses in 1938 (122), and subsequently six antigenically related subtypes (I through VI) have been identified based on serologic cross reactivity (123). Tonate virus is a subtype IIIB VEE complex virus that circulates in French Guiuan and has caused encephalitis (124,125). Subtype I viruses were further subdivided serologically into IAB, IC, ID, IE, and IF. More recent molecular genetic studies suggest a revision of some of these groups. VEEV circulates in an enzootic sylvatic (forest) cycle (Fig. 15.2) and emerges to cause major epizootics in horses and human epidemics every 10 to 20 years. Sylvatic VEEV is found in Central and South America, and although most epizootics have occurred in northern South America, particularly Venezuela and surrounding countries, they have extended as far north as Mexico and Texas (21,85,126). In its sylvatic cycle, VEEV is transmitted between small mammals and aquatic birds mainly by Culex melanoconion mosquitoes (Table 15.2). Sporadic human cases occur when people enter the swampy and forested locations where the viruses circulate or when bridging mosquitoes such as Aedes taeniorhynchus carry the virus to nearby human habitats. In contrast, human epidemics occur at the same time as equine epizootics when the virus emerges from its enzootic cycle and is transmitted between susceptible horses by Aedes, Psorophora, Mansonia, and Deinocerites mosquito species. Some studies suggest that the E2 protein encodes mosquito infection determinants for VEE, suggesting selection for efficient infection of epizootic mosquito vectors may mediate VEE emergence (127). Major epizootics and epidemics of VEE have occurred every 10 to 20 years in cattle-ranching areas of Venezuela, Peru, Colombia, and Ecuador. Although epizootic viruses (subtypes IAB and IC) were originally thought to be maintained in a separate transmission cycle from enzootic viruses (subtypes 1D, 1E, or 1F), later studies have suggested that epizootic IC viruses arise by mutation from sylvatic ID viruses (128–131). The 1C strains remain in the sylvatic cycle until the ecologic factors support its emergence into the epizootic cycle. Such outbreaks occur when heavy rainfall and flooding expand the mosquito breeding habitats and support expansion of the rodent population but also require a large susceptible equine population. Outbreaks of VEE IAB subtype that spread to Central America and Texas in the late 1960s and early 1970s are thought to have originated from improperly activated equine vaccine strains, as indicated by the fact that the epizootic strains are genetically almost identical to the vaccine strains (132).
Seroprevalence studies indicate that up to 50% of the population have antibody to VEEV in some sylvatic areas, whereas the remainder of the population is immunologically naive until an outbreak occurs. During the 1995 outbreak of VEE in Venezuela and Colombia, an estimated 85,000 human cases occurred, of which 3,000 had neurologic disease and 300 were fatal (128,133). One third of the human population seroconverted, and 8% of the equines died. Unlike most of the other arboviral encephalitides, human viremias are sufficiently high to infect mosquitoes, suggesting humans may not always be dead-end hosts. Isolation of virus from the pharynx of up to 40% of patients suggests direct spread between humans is possible (134). No epidemiologic evidence of such spread has ever been demonstrated (133,134).
Clinical Features
Compared with the other alphavirus encephalitides, only a very small proportion of symptomatic VEE infections results in severe neurologic disease. The incubation period is brief (<1 to 5 days), and most patients then develop a febrile illness with severe headache, made worse by eye movements, photophobia, facial flushing, conjunctival injection, myalgia, arthralgia, nausea, vomiting, and dizziness. Pharyngeal inflammation and pain associated with cervical lymphadenopathy is common, and there may be subcostal tenderness (133,135,136). Somnolence and tremulousness occur often. More severe neurologic features occur in 4% to 14% of patients, particularly the young and elderly. Seizures, particularly focal seizures, and raised ICP are common (133,137). In 5% to 10% of hospitalized patients, cranial nerve palsies, motor weakness, paralysis, or cerebellar signs are reported. Respiratory tract infections, including interstitial pneumonia and tracheobronchitis, are common and may result in secondary bacterial infection. Overall, 0.2% to 1.0% of symptomatic VEE infections are fatal, but this increases to up to 10.0% to 25.0% of those with encephalitis. Children are estimated to have ten times the risk of neurologic disease of adults, and younger children have a greater case-fatality rate.
Diagnosis
Rather like the arboviral fever-arthralgia-rash syndromes, VEEV infection is typically associated with a leukopenia and elevated serum aspartate amino transferase levels (135). When lumbar punctures are performed, the CSF reveals a lymphocyte pleocytosis that may reach several hundred cells/mm3 and is reported to be associated with an elevated CSF glucose level. As expected, given the relatively high viremias, VEEV can be isolated from blood and pharynx up to the eighth day of illness and can be detected by PCR (134). VEEV antibody can be detected by IgM capture ELISAs (138). A VEEV-specific blocking ELISA that also identifies serotype-specific antibodies against VEEV in sera of humans, equids, or rodents has been reported (139).
Treatment and Prevention
There is no antiviral treatment for VEE. Supportive measures include attention to seizures and increased ICP. Equine vaccines for VEE interrupt transmission during outbreaks and prevent epizootics. An inactivated vaccine has largely been replaced by a live vaccine (TC-83), attenuated by serial passage in guinea pig heart cells (140). VEEV is considered a useful agent for biological warfare, because of its potential for droplet spread and tendency to cause an incapacitating flulike illness, rather than a fatal encephalitis, and for this reason, the vaccine was produced by the U.S. military. TC-83 is given to laboratory workers and produces neutralizing antibodies in more than 80% of recipients; however, it is associated with a high incidence of adverse events including fever and aseptic meningitis (37%). Most of those who do not respond immunologically to the vaccine seroconvert when subsequently given a killed vaccine (TC-84). An improved genetically engineered vaccine V3526 is being developed (141,142). Another vaccine candidate in development is the recombinant, chimeric Sindbis/VEEV (SIN-83) that is more highly attenuated than TC-83 (143,144). In addition, the use of antisense technology and humanized monoclonal antibody has been shown to be effective against mice infected with VEEV (145,146).
Chikungunya Virus
Epidemiology
A large outbreak of a disease locally known as “Chikungunya” in the Newala district of Tanganyika (now Tanzania) occurred in 1952 to 1953 (147). However, CHIKV may have caused outbreaks as early as 1779 as the disease was frequently mistaken with dengue (148). In the local language, Chikungunya means “the disease that bends up the bones.” CHIKV was first isolated in 1952 from the serum of a febrile human during this outbreak (147,149). Although initially assessed as a dengue outbreak, further characterization of the isolates by serologic and antigenic techniques indicated that CHIKV was an alphavirus (150). Since then, frequent outbreaks of CHIKV have been reported from many African and Asian countries. There are three CHIKV genotypes (West African, East/Central African, and Asian) but only one CHIKV serotype, and therefore, infection with a given genotype should result in lifelong immunity against any genotype (151).
In Africa, CHIKV typically circulates in a sylvatic, enzootic cycle involving wild primates and forest-dwelling mosquitoes with sporadic infection of rural human populations. The principle enzootic mosquito vectors include Aedes furcifer-taylori (southern and western Africa), Aedes africanus (central Africa), Aedes luteocephalus and Aedes dalzieli (Senegal), Aedes cordellieri (South Africa) and Aedes neoafricanus. Human migration has attributed to the introduction of CHIKV to the urban setting, where human-to-human transmission occurs through domestic and peridomestic mosquito vectors. The main domestic vector, Aedes aegypti has been responsible for the major outbreaks of CHIKV in Comoros, India, Kenya, Seychelles, and Singapore during the early phases of the global emergence of CHIKV during 2004 to 2011 (151,152). However, during the later phases (2006 onward), genetic adaptation of CHIKV to a new vector, A. albopictus led to major outbreaks in several Indian Ocean islands (Reunion Island, Sri Lanka), India, and Southeast Asian countries (Singapore, Malaysia and Thailand) (153,154). The Indian outbreak resulted in an estimated 1.4 million cases during 2006 to 2009, whereas an estimated 266,000 cases (258 deaths) in the Reunion Island were reported, with an overall attack rate of 34% during 2005 to 2006 (152,155). Later studies confirmed that the epidemics in the Indian Ocean islands and in India were associated with an amino acid substitution in the E1 envelop glycoprotein (E1-A226V) in CHIKV, which allowed the virus to adapt for dissemination by A. albopictus. A further mutation (E2-L210Q) has been described in the 2009 Indian outbreak, which again resulted in an increase in the dissemination of CHIKV in A. albopictus (156). Autochthonous transmission of CHIKV has been reported in Italy (2007) with over 250 cases and in France (2010), signaling the emergence of CHIKV in Europe for the first time (157,158). Imported cases of CHIKV infection have now been reported in several European countries, Australia, and the Americas (159–161).
Clinical Features
The clinical features of CHIKV infection have some similarities with dengue infection (162). Asymptomatic CHIKV infections are rare. After an incubation period that can range from 1 to 12 (average of 2 to 4) days, a sudden onset of high fever followed by severe arthralgia and a skin rash develops. Almost all CHIKV patients have symmetrical polyarthralgia. The most affected joints are the fingers, wrists, ankles, elbows, toes, and knees. The fever can reach as high as 40°C and may last up to 10 days. A transient maculopapular and erythematous rash on the face, limbs, and torso is common and may last for 2 to 3 days. Other symptoms include myalgia, headaches, photophobia, lumbar back pain, chills, weakness, malaise, nausea, and vomiting (151,163). The acute phase of CHIKV infection usually resolves within 1 to 2 weeks, but the arthralgia may persist for many months or years in some patients (164,165). Age and underlying joint disorders have been shown to increase the likelihood of developing persistent arthralgia. The case-fatality rate for CHIKV is about 0.1%, with most deaths occurring in the elderly, neonates, and adults with an underlying disorder. Heart failure, multiple organ failure, hepatitis, and encephalitis are the most common causes of death (163).
CHIKV has not been generally considered to be a neurotropic virus. However, reports from recent outbreaks have given strong evidence of neurologic involvement of CHIKV. The main neurologic manifestations of adults infected with CHIKV are encephalopathy, acute flaccid paralysis, and Guillain-Barré syndrome (166–169). A recent study has reported that about 25% of adults admitted with atypical CHIKV infection during the 2005 to 2006 Reunion Island had neurologic involvement (mainly encephalitis, malaise, and meningoencephalitis) (170). Neurologic manifestations were more common in children. The most common manifestations were encephalitis, febrile seizures, and acute encephalopathies (171). For the first time, vertical transmission of CHIKV, from mother-to-child was reported during the Reunion Island outbreak. Encephalopathy was the most common neurologic manifestation among newborns infected by vertical transmission (172).
Diagnosis
A possible CHIKV case is suspected when a patient is presented with an acute onset of high fever and severe arthralgia unexplained by other medical conditions. The case becomes probable if the patient is living or has visited an endemic area within 15 days before onset of symptoms. The case is confirmed when the patient test positive for one of the diagnostic laboratory tests for CHIKV (163). Diagnosis of CHIKV is made during the acute phase of infection by detection of viral RNA in serum samples by RT-PCR and by virus isolation. Viral RNA can be detected in samples obtained from 1 day before symptom onset to day 7. Real-time RT-PCR assays capable of detecting a region of the nonstructural protein (nsp1) gene or the envelope (E) gene are also used for diagnosis (173,174). For later samples, indirect immunofluorescence and ELISA can be used to rapidly and sensitively distinguish between IgG and IgM antibodies. CHIKV antigen can be detected in serum and CSF samples obtained 2 days after onset of symptoms by antigen capture ELISA. Several commercial serologic assays as well as in-house ELISA techniques have been used for CHIKV diagnosis and have been shown to accurately distinguish CHIKV from other alphaviruses (except o’nyong-nyong virus) and flaviviruses (175–177).
Treatment and Prevention
Nonsteroidal antiinflammatory drugs are the only recommended treatment for arthralgia caused by CHIKV infection. Ribavirin, chloroquine, and passive transfer of antibodies are other potential treatments being investigated (163,178). Vector control remains the main method of CHIKV prevention (91,179). Several vaccine candidates are under development. These include inactivated, whole-virus, DNA-based, viruslike particle, and adenovirus-vectored vaccine approaches as well as a chimeric alphavirus vaccine (105,180–183).
FLAVIVIRUSES
Infectious Agent
There are three important genera in the family Flaviviridae: the genus Hepacivirus, which includes hepatitis C virus, the genus Pestivirus, which includes bovine viral diarrhea viruses, and the genus Flavivirus (Table 15.1). This genus includes many important causes of arboviral encephalitis, as well as arboviral fever-arthralgia-rash and hemorrhagic fever syndromes, such as dengue viruses and yellow fever virus (Fig. 15.1). The genus and family are named after the Latin word for yellow (flavus). Flaviviruses are thought to have evolved from a common ancestor as recently as 10,000 years ago and are rapidly evolving to fill new ecologic niches (184,185). They consist of a single strand of positive-sense RNA, wrapped in a nucleocapsid and surrounded by a glycoprotein-containing envelope. The RNA comprises a short 58 untranslated region (UTR), a longer 38 UTR, and between them a single open reading frame (186). This codes for a single polyprotein, which is cotranslationally and posttranslationally cleaved by viral and host proteases into three structural proteins (core, or C, premembrane, or PrM, and envelope, or E), and seven nonstructural (NS) proteins (NS1, NS2A, NS2B, NS3, NS4A, NS4B, and NS5). The C protein is highly basic and combines with the RNA to form the nucleocapsid. The PrM is closely associated with the E protein, forming a heterodimer, and is thought to act as a “chaperone” to it, impairing its function until after virion release. Immediately before virion release, the PrM protein is cleaved by a furin-like protease to its mature M protein form. This allows the formation of E protein homodimers, which are thus “activated” (187). The E protein is the largest structural protein, consisting of nearly 500 amino acids with up to two potential glycosylation sites. It is the major target for the humoral immune response and is thought to be important in viral entry into host cells. Studies with monoclonal antibodies and more recently with x-ray crystallography have determined the composition of the E proteins’ three domains (188,189). Domain III is the putative receptor-binding domain (by which virions attach to the yet-to-be-identified host cell receptor), domain II is the dimerization domain, and domain I has a central beta barrel and is the hinge domain that links the other two domains. Following viral attachment to the cell surface, flaviviruses enter cells by endocytosis. Subsequent fusion of the virus’ lipid membrane with the endosome membrane allows viral RNA to penetrate into the cytoplasm of the infected cell (186). Recent cryoelectron microscopic studies have shown an arrangement of 90 E-protein dimers lying flat on the surface of the virion, which rearrange to form E homodimers as the pH level decreases, exposing an internal fusion peptide and a patch of viral membrane for fusion. Interestingly, recent studies have shown the E1 protein of alphaviruses has a striking similarity to the flavivirus E protein, in terms of structure and function (96). Together, they have been labeled class II fusion peptides (190).
West Nile Virus
Epidemiology
WNV was first isolated from the blood of a febrile woman in the West Nile region of northern Uganda in 1937 (191). It was soon shown to be transmitted between vertebrate hosts (especially birds) by mosquitoes. Sporadic cases and larger outbreaks of febrile disease (West Nile fever) were reported in Africa, the Middle East, and Asia (192). Although meningeal irritation was noted in some patients with West Nile fever, the first cases of encephalitis due to WNV occurred when the virus was given as an experimental (and unsuccessful) treatment for advanced cancer in 1951. The first naturally occurring West Nile encephalitis cases were in the elderly residents of a nursing home in Israel (193). Outbreaks of equine and human meningoencephalitis occurred in southern France during the 1960s, and a subtype of WNV (Kunjin virus) was isolated in Australasia. Since the 1990s, the clinical epidemiology of WNV appears to have changed with increasing frequency and severity of outbreaks, including urban disease. These included an outbreak with nearly 400 confirmed cases in Romania in 1996, nearly 200 cases in the Volgograd region of Russia in 1999 and more than 200 cases in Israel in 2000 (194–197). In 1999, the virus appeared in North America for the first time, with 62 confirmed cases (198,199). It spread across the continent during 2000 and 2001, causing a largest encephalitis outbreak in 2002, with nearly 3,000 cases and 250 deaths (75). WNV is now the leading cause of domestically acquired arboviral disease in the United States of America and has spread throughout Canada, the Caribbean, Colombia, and Mexico (200,201). In 2010, WNV outbreaks occurred in Greece with 262 confirmed human cases with 197 cases of neuroinvasive WNV and in Romania with 57 cases, out of which 54 was neuroinvasive (202,203). Another outbreak occurred in Greece in 2011 with 31 cases of neuroinvasive WNV (204).
Most human infections with WNV are asymptomatic. In the 1998 to 1999 outbreaks, approximately 1 in 5 infections resulted in West Nile fever and only about 1 in 150 developed CNS disease (194,197). Over 75% of patients infected with WNV had neuroinvasive disease (encephalitis, meningitis, or acute flaccid paralysis) during the recent outbreak in Greece and over 94% during the Romanian outbreak (202,203). In contrast, the proportions were much lower in earlier outbreaks in Africa (192,205). In outbreaks across Europe and America, the risk of febrile disease and of neurologic disease increased with age, which may in part explain the differences compared to parts of Africa. In Egypt, most of the population is infected during childhood, and neurologic disease is rare (205). In a South African outbreak of 18,000 people of all ages, only a single encephalitis case was reported (192).
Recent molecular phylogenetic studies show that isolates of WNV can be divided into seven lineages. Lineage 1 has been subdivided into a further three clades: 1a, 1b, and 1c. Lineage 1b has spread to Australasia as Kunjin virus, whereas lineages 1a and 5 are found in India, and lineage 6 in Malaysia (206). Lineage 2 strains have mostly been found in Africa, whereas lineage 1 strains are more widely distributed and are responsible for all the large outbreaks during 1998 to 1999 (207,208). This has led to the suggestion that they may be more virulent, although neuroinvasive strains have been shown in both lineages in animal models (209). However, lineage 2 was more prevalent during the recent outbreaks in Europe (Greece, Romania, and Italy) and therefore may disperse wider in the future (210,211).
In nature, WNV is transmitted in an enzootic cycle between birds by mosquitoes. WNV infects over 300 different bird species and 50 mosquito species (1). Members of the order Passeriformes (jays, blackbirds, finches, warblers, sparrows, and crows) appear to be important in maintenance of the virus in nature (because of viremias); the family Corvidae (crows, blue jays) seems to be particularly susceptible Culex mosquitos, particularly Culex pipiens, appear to be important in the enzootic cycle, although different species may act as “bridging vectors” transmitting the virus to humans.
How WNV is introduced to new areas is not completely understood. Migratory birds are thought to be important for the movement of WNV from Africa into southern Europe. They may have been involved in the virus’ introduction into the United States, although imported exotic birds, a viremic human, or inadvertently transported mosquitoes seem more likely (212). Molecular genetic evidence suggests there was a single introduction into the United States of a strain closely related to one isolated from a goose in Israel (207). A complex interplay of viral, avian, mosquito, human, and climatic factors may contribute to the large outbreaks that have characterized the disease recently (Fig. 15.4). During the 2002 outbreak in the United States, transmission also occurred via transplanted organs, infected blood products, and possibly breast milk (213).
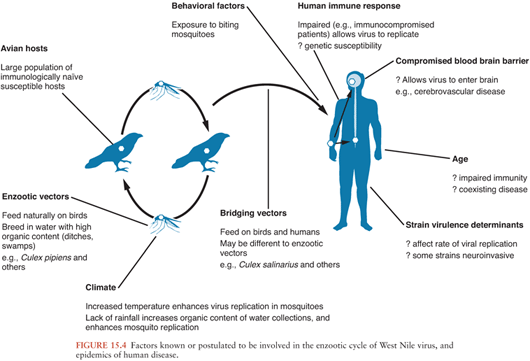
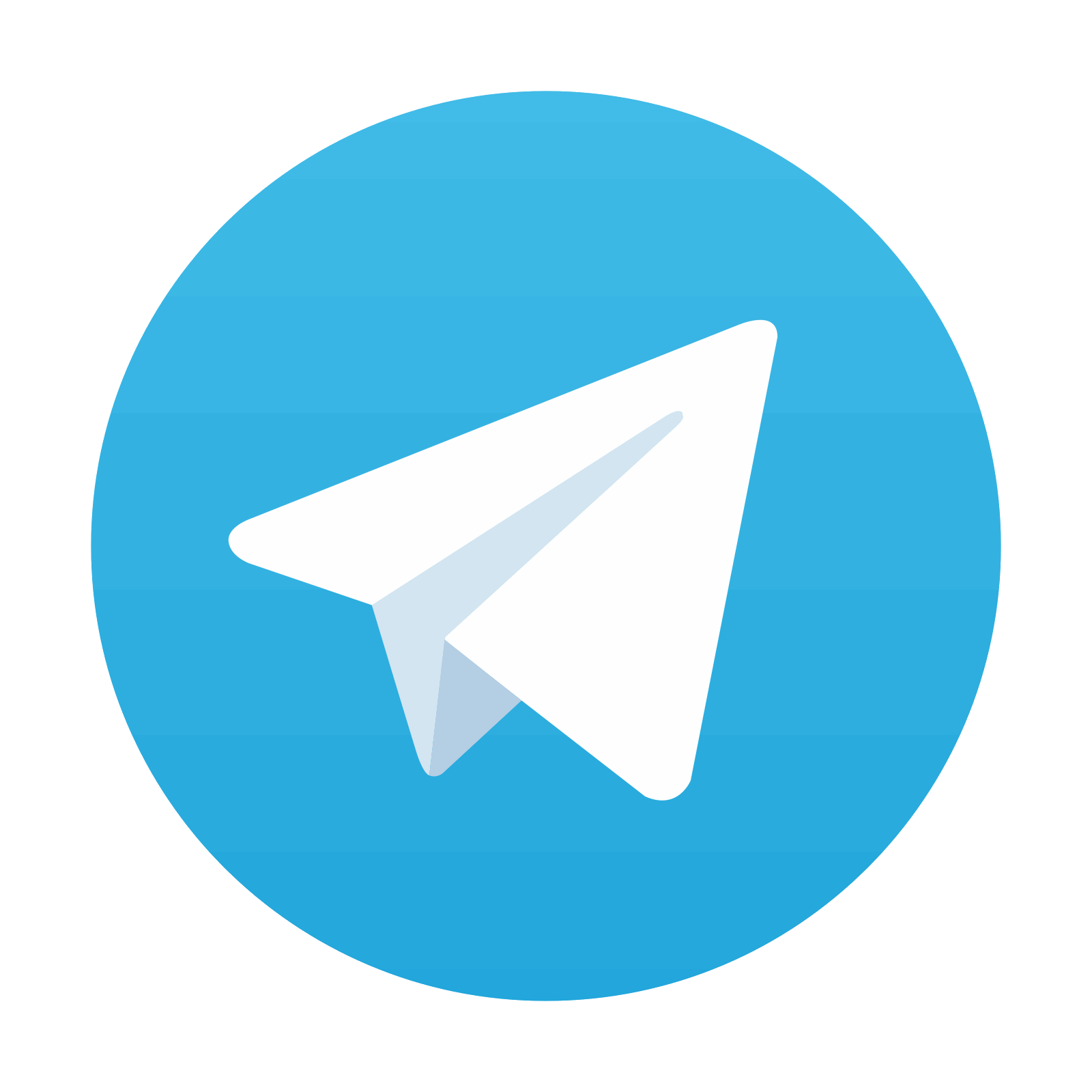
Stay updated, free articles. Join our Telegram channel

Full access? Get Clinical Tree
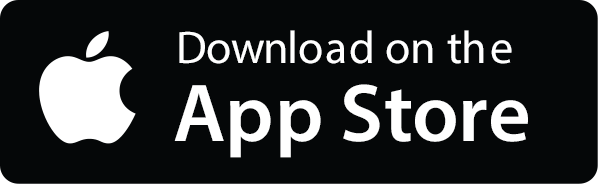
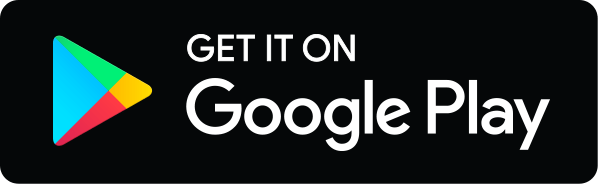