INTRODUCTION
SUMMARY
The anemia that results from deficiencies of vitamin B12, folic acid (Chap. 41) or iron (Chap. 43) are, in general, clearly defined and are relatively common. In contrast, characteristics of anemia that may occur with deficiencies of the other vitamins and minerals are poorly defined and relatively rare in humans. When present, they usually exist not as isolated deficiencies of one vitamin or one mineral, but rather, as a combination of deficiencies resulting from malnutrition or malabsorption. In this context, it is difficult to deduce which abnormalities are the result of which deficiency. Studies in experimental animals may not accurately reflect the role of micronutrients in humans. Accordingly, our knowledge of the effects of many micronutrients on hematopoiesis is fragmentary and based on clinical observations and interpretations that may be flawed. Inborn metabolic errors that affect single micronutrient pathways may shed light on specific effects of those micronutrients on hematopoiesis. Daily requirements of some micronutrients are available at: http://www.nal.usda.gov/fnic/dga/rda.pdf, and levels normally found in serum, red cells, and leukocytes are shown in Table 44–1.
Acronyms and Abbreviations:
MCHC, mean corpuscular hemoglobin concentration; MCV, mean corpuscular volume; RDW, red cell distribution width; T3, triiodothyronine; T4, thyroxine.
Vitamin or Mineral | Serum Level | Plasma Level | Red Cell Level | White Cell Level |
---|---|---|---|---|
Copper | 11–24 μmol/L | 14–24 μmol/L | ||
Folate | 7–45 nmol/L | >320 nmol/L | ||
Riboflavin (B2) | 110–640 nmol/L | 265–1350 nmol/L | ||
Vitamin A | 1–3 μmol/L | |||
Vitamin B6 | 20–122 nmol/L | |||
Vitamin C | 25–85 μmol/L | 11–30 attomol/cell | ||
Vitamin E | 12–40 μmol/L | |||
Selenium | 1200–2000 nmol/L | |||
Zinc | 11–18 μmol/L |
VITAMIN-DEFICIENCY ANEMIAS
Chronic deprivation of vitamin A results in anemia similar to that observed in iron deficiency.1,2,3,4 Mean corpuscular volume (MCV) and mean corpuscular hemoglobin concentration (MCHC) are reduced. Anisocytosis and poikilocytosis may be present, and serum iron levels are low. Unlike iron-deficiency anemia, but similar to anemia of chronic disease, iron stores in the liver and marrow are increased, serum transferrin concentration usually is normal or decreased, and administration of medicinal iron does not correct the anemia. However, there is some evidence to suggest that vitamin A deficiency may result in impaired iron absorption or utilization5 and this may be mediated through effects on the expression of genes involved in the regulation of intestinal iron absorption.6 The suggestion that vitamin A may facilitate iron absorption7 was not confirmed.8 Supplementation with vitamin A alone may ameliorate the anemia, although coadministration of vitamin A and iron resulted in a better response than with either nutrient alone.9
Surveys conducted in developing countries suggest that vitamin A deficiency represents a public health problem among infants, schoolchildren and women of childbearing age.10,11 The prevalence of vitamin A deficiency closely coincides with the prevalence of iron deficiency in this demographic setting. However, there is no known causal relationship between the two nutrients beyond both occurring in a setting of generalized malnutrition. Although vitamin A deficiency is recognized to occur in the United States, the relationship between it and anemia is not known.
Isolated nutritional deficiencies of members of the vitamin B group, with the exception of folic acid and vitamin B12, are very uncommon in humans. Evidence linking isolated nutritional deficiencies of pyridoxine, riboflavin, pantothenic acid, and niacin to anemia in patients is inconclusive. In animals, experimentally induced deficiency states are more commonly associated with hematologic abnormalities.
Vitamin B6 includes pyridoxal, pyridoxine, and pyridoxamine. These components are converted to pyridoxal 5-phosphate, which acts as a coenzyme in decarboxylation and transamination of amino acids and synthesis of aminolevulinic acid, the porphyrin precursor (Chap. 58). Vitamin B6 deficiency induced in infants is associated with a hypochromic microcytic anemia.12 A malnourished patient with a hypochromic anemia who failed to respond to iron therapy but subsequently responded to administration of vitamin B6 has been described.13 In some anemic pregnant women who did not respond to iron supplementation alone, vitamin B6 administration resulted in subsequent improvement in hemoglobin level.14 Occasionally, patients receiving therapy with antituberculosis agents, such as isoniazid, which interfere with vitamin B6 metabolism, develop a microcytic anemia that can be corrected with large doses of pyridoxine.15 Pyridoxine is usually prescribed with isoniazid to prevent such an effect. Some patients with sideroblastic anemias (Chap. 59) respond to administration of large doses of pyridoxine, but these patients are not deficient in this vitamin. A review of more than 200 patients with acquired sideroblastic anemia reported that fewer than 7 percent showed greater than 1.5 g/dL improvement in hemoglobin concentration with pyridoxine treatment.16 Pyridoxine is involved in many metabolic processes. Derangements in these pathways, sometimes involving anemia, are usually the result of inborn errors affecting the pathways of vitamin B6 metabolism and specific pyridoxal phosphate-dependent enzymes or inborn errors that lead to accumulation of small molecules that react with pyridoxal phosphate and inactivate it.17 Other acquired conditions that may influence pyridoxine metabolism include drugs that react with pyridoxal phosphate or affect metabolism, malabsorptive states such as celiac disease, and renal dialysis which leads to increased loss of vitamin B6 vitamers from the circulation as these vitamers are bound to plasma albumin.18
Riboflavin deficiency results in a decrease in red cell glutathione reductase activity because this enzyme requires flavin adenine dinucleotide for activation. Glutathione reductase deficiency, induced by riboflavin deficiency, is not associated with hemolytic anemia or increased susceptibility to oxidant-induced injury (Chap. 47).19 Human volunteers maintained on a semisynthetic riboflavin-deficient diet and fed the riboflavin antagonist, galactoflavin, developed pure red cell aplasia.20 Vacuolated erythroid precursors are evident prior to the development of aplasia. This anemia is reversed specifically by administration of riboflavin. It has been suggested that riboflavin deficiency causes anemia,21 possibly by interfering with iron release from ferritin.20 Although the relationship between dietary riboflavin deficiency and anemia is not clear, inadequate riboflavin intake increased the risk of anemia in Chinese adults and was associated with a high probability of anemia when iron intake was low.16 Thus, poor riboflavin status may interfere with iron handling and contribute to the etiology of anemia when iron intake is low. There is also some evidence to suggest that riboflavin may exert its effects secondarily on other nutrients, such as folate and cobalamin.22
Pantothenic acid deficiency, when artificially induced in humans, is not associated with anemia.23
Pellagra (niacin deficiency) is associated with anemia, which responds to treatment with niacin.24 However, it is not clear whether the anemia is a direct or indirect effect of niacin deficiency.
Megaloblastic anemia, responsive to thiamine, occurs in a childhood syndrome in association with diabetes and sensorineural deafness. There is usually profound anemia, megaloblastic changes with or without ringed sideroblasts in the marrow, and occasionally thrombocytopenia.25 Most cases have been reported in patients of Middle and Far Eastern descent. The underlying defect in this condition is in the high-affinity thiamine transporter, which primarily affects synthesis of nucleic acid ribose via the nonoxidative branch of the pentose cycle.26 This decrease in ribose synthesis is a consequence of the thiamine-dependent pentose-cycle enzyme transketolase. Reduced nucleic acid production through impaired transketolase catalysis appears to be the underlying biochemical disturbance that likely induces cell-cycle arrest or apoptosis in marrow cells and leads to thiamine-responsive megaloblastic anemia syndrome in these patients, which responds to lifelong administration of oral thiamine (25 to 100 mg/day). The SLC19A2 gene on chromosome 1q23.3 is implicated in all cases of thiamine-responsive megaloblastic anemia.27 The folate carriers and thiamine transporters evolved from the same family of solute carriers.28
Although approximately 80 percent of patients with scurvy29 are anemic, attempts to induce anemia in human volunteers by severely restricting dietary ascorbic acid have been unsuccessful.30 Anemia observed in subjects with scurvy is not simply the result of a deficiency of ascorbic acid, but rather a result of bleeding or deficiency of folic acid.29 Human subjects with scurvy and megaloblastic anemia fail to correct their anemia with vitamin C administration if they are maintained on a folic acid–deficient diet. When folic acid is given to these patients in a dose of 50 mcg/day orally, a prompt hematologic response is observed.31
Ascorbic acid, in common with other compounds that contribute to cellular reducing potential, participates in maintenance of dihydrofolate reductase in its reduced, or active, form. Impaired dihydrofolate reductase activity results in an inability to form tetrahydrofolic acid, the metabolically active form of folic acid (Chap. 41). Patients with scurvy and megaloblastic anemia excrete 10-formylfolic acid as the major urinary folate metabolite. Following ascorbic acid therapy, 5-methyltetrahydrofolic acid becomes the major urinary folate metabolite. This observation has led to the suggestion that ascorbic acid prevents the irreversible oxidation of methyltetrahydrofolic acid to formylfolic acid.32 Failure to synthesize tetrahydrofolic acid or protect it from oxidation ultimately results in megaloblastic anemia. Under these circumstances, ascorbic acid therapy produces a hematologic response only if enough folic acid is present to interact with the ascorbic acid.33 Dietary iron deficiency in children often occurs in association with dietary ascorbic acid deficiency. Iron balance may be compromised by ascorbic acid deficiency because this vitamin serves to facilitate intestinal iron absorption by maintaining iron in a more soluble reduced or ferrous (Fe2+) state. Patients with scurvy, particularly children, may require both iron and vitamin C to correct hypochromic microcytic anemia.34 Vitamin C affects the oxidoreduction involved in compartmental iron release and may stimulate iron mobilization from endosomes, as well as transferrin-dependent iron uptake. Scurvy itself may cause iron deficiency as a consequence of external bleeding. In patients with iron overload from repeated blood transfusions, the level of vitamin C in leukocytes is often decreased because of rapid conversion of ascorbate to oxalate.35 Deferoxamine (desferrioxamine)-induced iron excretion is diminished when stores of vitamin C are reduced, but excretion returns to expected values with vitamin C supplementation.36,37 Large doses of ascorbic acid may be harmful in patients with iron overload and should be given only after an infusion of deferoxamine mesylate (Desferal) has been initiated (Chap. 43). The presence of scurvy in patients with iron overload may protect them from tissue damage.38 In scorbutic guinea pigs and Bantu subjects with nutritional vitamin C deficiency and dietary hemosiderosis, iron accumulates in the monocyte-macrophage system rather than in the parenchymal cells of the liver.39,40
Vitamin E, α-tocopherol, is a fat-soluble vitamin that appears to be an antioxidant in humans. It is not an essential cofactor in any recognized reactions. Nutritional deficiency of vitamin E in humans is extremely uncommon because of the widespread occurrence of α-tocopherol in food. The daily requirement of d-α-tocopherol for adults ranges from 5 to 7 mg, but this requirement varies with the polyunsaturated fatty acid content of the diet and the content of peroxidizable lipids in tissues. Hematologic manifestations of vitamin E deficiency in humans are limited to the neonatal period and to pathologic states associated with chronic fat malabsorption.
Low-birthweight infants are born with low serum and tissue concentrations of vitamin E. When these infants are fed a diet unusually rich in polyunsaturated fatty acids and inadequate in vitamin E, hemolytic anemia frequently develops by 4 to 6 weeks of age, particularly if iron is also present in the diet.41 This anemia often is associated with morphologic alterations of the erythrocytes,42 thrombocytosis, and edema of the dorsum of the feet and pretibial area.43 Treatment with vitamin E produces a prompt increase in hemoglobin level, a decrease in the elevated reticulocyte count, normalization of red cell life span, and disappearance of thrombocytosis and edema. Modifications of infant formulas have all but eliminated vitamin E deficiency in preterm infants.44
Vitamin E deficiency is common in patients with cystic fibrosis who are not receiving daily supplements of a water-soluble form of the vitamin.45 Red cell life span in such patients is shortened to an average chromium-51 (51Cr) half-life of 19 days (normal: approximately 30 days). Severe anemia may be present.45 After vitamin E therapy, red cell half-life increases to 27.5 days.46
Pharmacologic doses of vitamin E have been employed with apparent success in the absence of vitamin deficiency to compensate for genetic defects that limit erythrocyte defense against oxidant injury. Chronic administration of oral vitamin E 400 to 800 U/day lengthened red cell life span in some,47,48 but not all,49 studies of patients with hereditary hemolytic anemias associated with glutathione synthetase deficiency or glucose-6-phosphate dehydrogenase deficiency.
Administration of vitamin E (450 U/day for 6 to 36 weeks) to patients with sickle cell anemia significantly reduced the number of irreversibly sickled erythrocytes.50 Adult patients with sickle cell anemia have been reported to have significantly lower serum tocopherol values compared with normal controls,51,52 and in children with sickle cell anemia, those with vitamin E deficiency have significantly more irreversibly sickled cells than did children without vitamin E deficiency.53
TRACE METAL DEFICIENCY
Copper is present in a number of metalloproteins. Cytochrome c oxidase, dopamine β
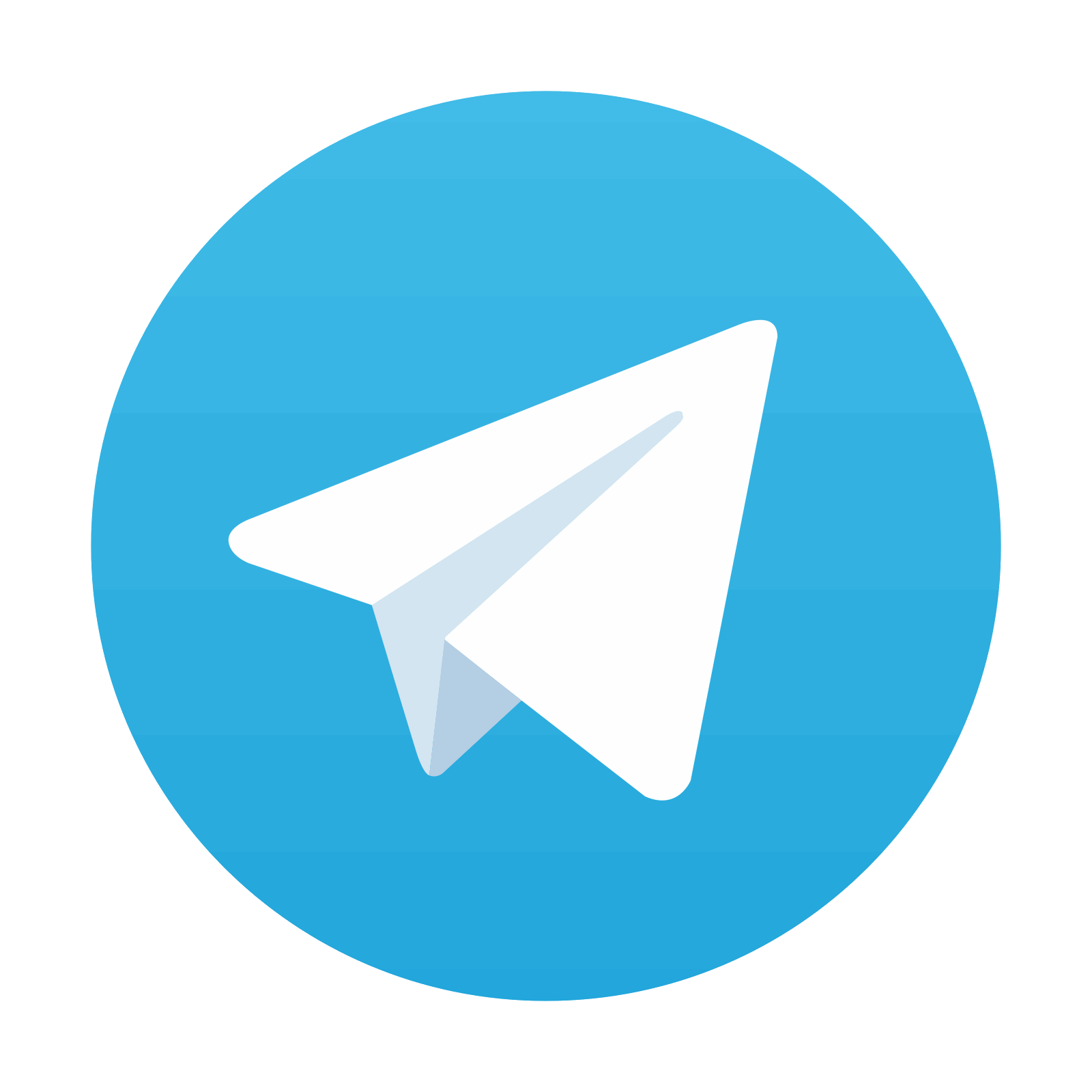
Stay updated, free articles. Join our Telegram channel

Full access? Get Clinical Tree
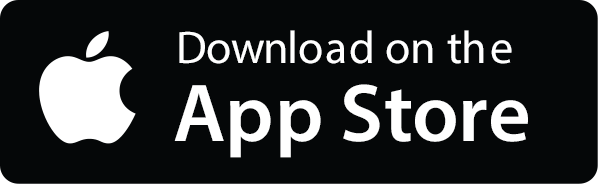
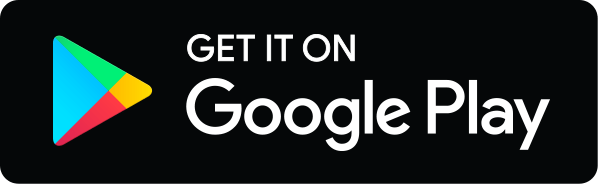
