OVERVIEW
Brain tumors are a heterogeneous group of lesions that range from benign, slow-growing tumors found only incidentally on autopsy, to malignant, rapidly growing tumors that cause death within months. The most common intracranial tumors are brain metastases from systemic cancer, estimated at 200,000 new cases per year in the United States, based on a 10% to 15% incidence (1). In comparison, the incidence of primary brain and spinal cord tumors for 2014 was estimated at 23,380 new cases (American Cancer Society 2014 Facts and Figures [http://www.cancer.org/acs/groups/content/@research/documents/webcontent/acspc-042151.pdf (or, cancer.org]).
Because of the heterogeneous histology and often-refractory nature of primary brain tumors, their management is complex, ideally requiring a multidisciplinary team and individualized treatment. The diagnosis is made on the basis of histology, so an accurate characterization of the lesion pathology is crucial, often necessitating confirmation at a specialized cancer center. Optimal outcomes involve the coordination of neurosurgery, radiation oncology, and neuro-oncology specialists. Despite advances in neurosurgical techniques, radiation therapy, and chemotherapy, the prognosis for patients with high-grade gliomas such as glioblastoma (GBM), the most common form of glioma, remains dismal. Recent large clinical trials have reported a median survival of only 14 to 16 months with a 26% to 33% 2-year survival rate (2,3). A review of eight consecutive phase II chemotherapy trials for recurrent GBM demonstrated only a 6% response rate (complete response [CR] and partial response [PR]), with a 6-month progression-free survival (PFS) of 15% and a 1-year survival of 21% (4). It is therefore important to consider patients with high-grade gliomas for entry into clinical trials at all stages of disease because new therapies target patients from initial diagnosis, with presurgical protocols, to salvage therapy at relapse. This chapter provides basic principles that can be used for diagnosing and treating patients with brain tumors along with an introduction to the molecular mechanisms underlying gliomagenesis.
CLASSIFICATION AND INCIDENCE
Brain tumors are either primary tumors that arise de novo or secondary metastases, the latter being far more common. Most commonly, brain metastases result from lung cancer, followed by breast, melanoma, renal, and colorectal cancers. Most patients with brain metastases die from progression of their systemic cancer, although, because of improvements in systemic therapy, brain metastases are now seen more frequently and with increasing morbidity and mortality. On a more hopeful note, advances in treating brain metastasis with surgery and radiotherapy (RT) have improved overall survival when systemic disease is controlled.
Primary brain tumors are classified by the World Health Organization (WHO) grading system (Table 40-1), which is based on the histologic pattern of cell differentiation in the tumor, in addition to histologic features associated with biological aggressiveness (ie, mitotic figures, necrosis, vascular proliferation). Tumor grade is inversely correlated with prognosis. The most common primary brain tumors are gliomas (all glial tumors), followed by meningiomas, nerve sheath tumors, and pituitary tumors (5).
WHO grade I | |
Pilocytic astrocytoma | Meningioma |
Myxopapillary ependymoma | Craniopharyngioma |
Subependymoma | |
WHO grade II | |
Diffuse astrocytoma | Ependymoma |
Pleomorphic xanthoastrocytoma | Pineocytoma |
Oligodendroglioma | Atypical meningioma |
Oligoastrocytoma | |
WHO grade III | |
Anaplastic astrocytoma | Anaplastic oligoastrocytoma |
Anaplastic oligodendroglioma | Anaplastic ependymoma |
Anaplastic (malignant) meningioma | |
WHO grade IV | |
Glioblastoma | Pineoblastoma |
Gliosarcoma | Medulloblastoma |
Brain metastases occur far more commonly than primary brain tumors. Nearly any type of primary cancer can metastasize to the brain, including hematologic malignancies. Most commonly, brain metastases originate from lung cancer, which represents the second most common systemic cancer in men and women (6). The next most common pathology to metastasize to the brain is breast cancer, followed by melanoma, renal cancer, and colorectal cancer (7). Among solid tumors, melanoma has the highest propensity to metastasize to the brain. Based on autopsy findings, 40% to 60% of patients with melanoma develop brain metastases (8). Most brain metastases, particularly melanoma, present with multiple lesions, although when renal cancer metastasizes to the brain, it often results in a single lesion. Approximately 10% of metastatic lesions will present as an intraparenchymal hemorrhage. Hemorrhagic metastases are most commonly seen in patients with melanoma, renal cell carcinoma, thyroid carcinoma, and choriocarcinoma. The pattern of distribution of metastases in the brain varies depending on the primary cancer.
The incidence of brain metastases appears to be rising, which may be a consequence of increasingly sensitive imaging modalities such as magnetic resonance imaging (MRI), greater use of imaging, and the increasingly prolonged survival of patients with metastatic disease. The development of brain metastases usually occurs in the context of systemic relapse, although relapses can be isolated to the central nervous system (CNS). The impermeability of the blood–brain barrier (BBB), which often limits chemotherapy penetration into the CNS, may be the culprit in circumstances of isolated brain relapse.
Gliomas are the most frequently occurring primary brain tumors and include astrocytomas, oligodendrogliomas (ODs), and ependymomas. Combined, these histologies account for approximately 40% of all primary brain tumors and over 80% of all malignant CNS tumors (9). The next most common tumor is meningioma (32%), followed by nerve sheath tumor (9%) and pituitary tumor (8%) (9). The most recent data from the Central Brain Tumor Registry of the United States reported an incidence of all primary benign and malignant CNS tumors of 21.03 cases per 100,000 for a total count of 326,711 incident tumors (10).
The incidence of primary brain tumors differs by age. Glioblastomas, which account for more than half of all gliomas, typically peak in incidence between ages 65 and 74, anaplastic gliomas peak between ages 45 and 54, and low-grade gliomas are most typically seen between ages 20 and 34 (5). In addition to the disparities of age, gender differences are seen in the incidence of primary brain tumors. The incidence of malignant brain tumors per 100,000 person-years in males is 7.7, compared with 5.4 for females (10) (Fig. 40-1).
FIGURE 40-1
Distribution of all primary brain and central nervous system tumors by histology, Central Brain Tumor Registry of the United States (CBTRUS) 2007-2011 (n = 343,175). (Reproduced with permission from Ostrom QT, Gittleman H, Liao P, et al: CBTRUS statistical report: primary brain and central nervous system tumors diagnosed in the United States in 2007-2011, Neuro Oncol 2014 Oct;16 Suppl 4:iv1-63).
Primary CNS lymphoma (PCNSL), concordant with AIDS, has decreased since its peak in the early 1990s, when it reached 10.2 per 1 million person-years. By 1998, the incidence decreased to 5.1 per 1 million person-years, which was attributed to the treatment of human immunodeficiency virus (HIV) with highly active antiretroviral therapy (HAART) in males under the age of 60 (11). An increased incidence in PCNSL is seen not only in HIV/AIDS but also in iatrogenic immunosuppression, such as organ transplant, autoimmune disease, and cancer. The rate for persons over 60 years of age, however, has remained stable since 1994, at approximately 16 per 1 million person-years (11).
Although many factors have been considered as putatively involved in gliomagenesis, therapeutic ionizing radiation is the strongest established causative agent underlying the development of brain tumors. Children with acute lymphoblastic leukemia (ALL), following prophylactic cranial irradiation, have an increased risk for both gliomas and meningiomas (12). Increased risk of brain tumors has been seen following therapeutic irradiation for pituitary tumors. Even low doses of radiation previously used to treat scalp ringworm increased the risk of developing nerve sheath tumors, meningiomas, and gliomas (13). Fortunately, diagnostic radiation does not appear to be strongly associated with the development of gliomas (14).
While a link between brain tumors and chemical exposure has been suggested, no specific agent has been identified with a link to brain tumors that can be validated with an exposure-disease correlation. No consistent link has been proven for the occurrence of cancer in agricultural workers. Positive correlations, but not causation, have been drawn between the occurrence of brain tumors and occupations involving exposure to synthetic rubber, vinyl chloride, and petroleum refining (15). Whereas smoking is implicated in an increased risk for many cancers, it is not associated with an increased risk of brain tumors. Exposure to cured foods has been linked to meningiomas, and nitrosamines have been associated with gliomas with a relative risk of 1.48 in adults with a high intake of cured meat (16). No correlation between alcohol intake or cosmetic use and brain tumors has been found (17).
There has been concern, particularly in the popular press, about the relationship between exposure to cell phones and the risk for brain tumors. Thus far, several case-control studies and a cohort study have failed to establish such a link.
Other than the increased incidence of PCNSL in patients with HIV infection, associations between viral exposures and brain tumors have not been consistent. Human cytomegalovirus (CMV) has recently garnered increasing attention in regard to its role in gliomagenesis. There is sufficient evidence that CMV sequences and viral gene expression exist in most gliomas and that CMV could modulate the malignant phenotype in GBM, but a specific role of CMV in glioma development has yet to be defined (18).
A protective effect of allergies (asthma, eczema, and hay fever) was noted in a meta-analysis of over 3,000 patients, with a relative risk of glioma incidence of 0.61 (19), supporting a role for immune modulation in brain tumor genesis. A retrospective case-control series demonstrated a decreased incidence of glioma in patients who reported histories of chickenpox, shingles, herpes simplex virus and Epstein-Barr virus (20), and as a surrogate for exposure to infections in early life, birth order was correlated with increased risk for the development of glioma in adulthood (21).
Relatively few brain tumors are attributable to heredity; studies have cited from 1% to 5% (22). Brain tumors can arise as a component of familial tumor syndromes, such as neurofibromatosis type 1 (NF1). Patients with NF1 are at increased risk for the development of gliomas originating predominantly in the optic pathway and brainstem. These neoplasms are typically pilocytic astrocytomas with a tendency toward a more indolent course in comparison to their sporadic counterparts. Neurofibromatosis type 1 is caused by a mutation of the NF-1 gene and is also associated with leukemia and pheochromocytoma. Neurofibromatosis type II is marked by a mutation of the NF-2 gene and is associated with bilateral vestibular schwannomas, meningiomas, and gliomas, including an increased risk for ependymomas. The Li-Fraumeni syndrome results from an autosomal dominant mutation of the p53 tumor suppressor gene, located on chromosome 17p. This p53 mutation results in many types of malignancies, including glioma and medulloblastoma, as well as sarcoma, breast cancer, leukemia, and adrenocortical cancer. Turcot syndrome is an autosomal dominant disease characterized by multiple polyps of the gastrointestinal tract as well as brain tumors. Two separate mutations have been identified in Turcot syndrome. One involves the APC (adenomatous polyposis coli) gene, which is associated with medulloblastoma. A mutation of the hMLH1 DNA mismatch repair gene is associated with GBM.
BIOLOGY AND MOLECULAR GENETICS
The understanding of cancer relies on uncovering the underlying molecular biologic mechanisms and signaling pathways that drive tumorigenesis. Delineating such mechanisms is complicated by the tremendous degree of intertumoral and intratumoral heterogeneity. Newer drugs target specific extracellular receptors or block intracellular signal transduction systems.
Malignant glial tumors often exhibit significant histologic heterogeneity, which is reflected at the molecular level. Large-scale profiling efforts have accelerated our understanding of gliomas. The landscape of somatic genomic alterations has been comprehensively characterized by The Cancer Genome Atlas (TCGA). In the most recent publication by TCGA in 2013, alterations in three core overlapping pathways were described: receptor tyrosine kinase/RAS/phosphatidylinositol 3 kinase (RTK/RAS/PI3K) signaling (altered in 90% of GBMs), p53 signaling (altered in 86% of GBMs), and RB signaling (altered in 79% of GBMs). The alterations affecting the p53 pathway (MDM2, MDM4, and TP53); the Rb pathway (CDK4, CKD6, CCND2, CDKN2A/B, and RB1); and the PI3K pathway (PIK3CA, PIK3R1, PTEN, EGFR, PDGFRA, and NF1) were found to be mutually exclusive (23). Of the 251 GBMs analyzed, at least one RTK was found altered: EGFR (57.4%), PDGFRA (13.1%), MET (1.6%), and FGFR2/3 (3.2%). Mutations of PI3Kinase were found in 25.1% of GBMs and were found to be mutually exclusive for PTEN mutations/deletions. The p53 pathway was found to be altered in 85.3% of tumors through mutations/deletion of TP53 (27.9%), amplification of MDM1/2/4 (15.1%), or deletion of CDKN2A (57.8%) (23). The most recent TCGA analysis also identified mutations in genes for which targeted therapies have been developed, including BRAF (24) and FGFR1/FGFR2/FGFR3 (25).
The epidermal growth factor (EGF) pathway was found to be altered in 57.4% of GBMs in the most recent TCGA data. An alternate mutation in the external domain generates a truncated receptor, the EGFRvIII mutant, which is constitutively activated in gliomas (26). Growth factors such as EGF and platelet-derived growth factor (PDGF) activate multiple signal transduction pathways that lead to cell survival and proliferation. The EGFR can activate the PI3Kinase pathway, which is also frequently mutated in glioma. When the PI3Kinase pathway is activated, the activation of AKT (protein kinase B) is triggered, in turn activating multiple prosurvival pathways, such as nuclear factor kappa B (NFκB), forkhead, and glycolysis. The activation of the PI3Kinase pathway has been associated with the reduced survival of patients with glioma (27). Growth factors can also stimulate the ras pathway, which initiates a signal cascade through Raf/MEK/Erk and also promotes cell survival and tumorigenesis (28). PTEN has phosphatase activity that inhibits the PI3Kinase pathway. The deletion of MMAC/PTEN leads to AKT pathway activation. The activation of p53 can result in either apoptosis or cell cycle arrest and initiation of DNA repair mechanisms. The abrogation of p53 activity would be expected to increase proliferation and mutations, leading to genetic instability, the prodrome of tumorigenesis.
Cell cycle regulation is a key target of carcinogenesis. Cell cycle checkpoints are affected by multiple proteins, acting either as accelerators or inhibitors of cell regulation. Important molecules in this process include p53, p21, and MDM. Another cell cycle regulation pathway important for glial tumors involves the retinoblastoma (RB) gene. When the RB gene is phosphorylated, the E2F transcription factor is released and activates cellular proliferation. The regulation of RB activity is complex and involves multiple cyclins (cyclin D), cyclin-dependent kinases (CDK4/6), and CDK inhibitors (p16), whose activities are under investigation (29).
The majority of GBMs (~90%) are primary, characterized by rapid development in the absence of any clinical or histologic evidence of a lower-grade lesion, in contrast to secondary GBMs, which progress from a low-grade astrocytoma or anaplastic astrocytoma (AA). Primary and secondary GBMs arise from distinctly different genetic pathways. Primary GBMs are postulated to develop quickly from glial progenitor cells over a period of a few months, potentially acquiring mutations in EGFR, TP53, or PTEN. In contrast, secondary GBMs are believed to arise from a stepwise accumulation of mutations.
Isocitrate dehydrogenase (IDH) mutations are believed to be a very early event in gliomagenesis that persist during progression to secondary GBM. The IDH1/2 mutations likely occur prior to the acquisition of a TP53 mutation, a driving force toward astrocytic differentiation, and prior to the acquisition of 1p/19q loss, believed to be the driving force toward oligodendroglial differentiation. Codeletion of 1p/19q has been classified as the genetic signature of ODs. Recent exomic sequencing has revealed that mutations in the CIC gene at 19q13.2 and FUBP1 gene at 1p are also frequently observed in ODs. In addition to the acquisition of TP53 mutations, ATRX mutations are often noted in WHO grade II and III astrocytomas and secondary GBMs (30).
In anaplastic oligodendrogliomas (AODs), allelic losses of chromosomes 1p and 19q (1p19q LOH) have emerged as markers of chemotherapeutic response and longer survival (31), qualifying 1p19q loss as a prognostic factor. Recent studies have confirmed that 1p/19q LOH is also predictive of response to chemotherapy. In independent seminal studies by the European Organization for Research and Treatment of Cancer (EORTC) and Radiation Therapy Oncology Group (RTOG), patients with AODs harboring 1p/19q LOH did significantly better when treated with a combination of radiation and chemotherapy compared to treatment with radiation alone. Tumors without 1p/19q LOH did not incur a survival benefit (32,33,34).
O6-Methylguanine-DNA methyltransferase (MGMT) is a DNA repair protein that reverses DNA damage induced by alkylating agents such as temozolomide and has been implicated as a major mechanism of resistance to alkylating agents. Hypermethylation of the promoter region of the MGMT gene, which inactivates MGMT gene transcription, has been associated with response to alkylating agents and increased survival in glioma patients (35).
Isocitrate dehydrogenase mutations were first reported in 2008 (36) and quickly became a seminal discovery. The IDH mutations now definitively distinguish primary from secondary GBM and have been established as a positive prognostic factor with an increase in overall survival noted in patients harboring an IDH mutation over those with wild-type IDH (37). In low-grade glioma, anaplastic glioma, and GBM, IDH mutation status appears to be the most important prognostic factor (36,38). In a study of 382 patients with high-grade gliomas, IDH1 mutation was found to be of greater prognostic relevance than histological diagnosis according to the current WHO classification system. The sequence of more favorable to poorer outcome was (1) AA with IDH1 mutation, (2) GBM with IDH1 mutation, (3) AA without IDH1 mutation, and (4) GBM without IDH1 mutation (38).
A mutation in the tumor suppressor NF2 gene on chromosome 22q12, has been closely associated with meningiomas and is disrupted in approximately half of meningiomas (39). Germline mutations of this gene result in neurofibromatosis type 2, an autosomal dominant disorder that can manifest as multiple meningiomas, bilateral schwannomas, gliomas, and intracranial calcifications (40). Merlin, the product of the NF2 gene, functions as a tumor suppressor gene and is a member of a family of cytoskeleton-associated proteins linked to RTK activity and ECM interactions (41).
In a recent study, whole-genome or whole-exome sequencing was performed on 17 sporadic meningiomas. The majority of meningiomas harbored simple genomes, with fewer mutations, rearrangements, and copy number alterations than observed in other adult tumors. Focal NF2 inactivation was confirmed in 43% of tumors. A subset of meningiomas lacking NF2 alterations exhibited recurrent oncogenic mutations in AKT1 and SMO with immunohistochemical (IHC) evidence of activation of their pathways. Mutations involving SMO were observed in 3/17 tumors, and AKT1 mutations were noted in 5/17 samples. Interestingly, these mutations were seen in the more therapeutically challenging tumors involving the skull base and were of higher grade (42). Hyperactive Hedgehog (Hh) signaling has been linked to many other cancers and is under active investigation in meningiomas highlighting SMO as a potential therapeutic target (43). Inhibitors of PI3K/AKT/mTOR are also under active investigation for meningiomas harboring AKT1 mutations.
The development of brain metastases is an intricate sequential process. In addition to proliferating, tumor cells must migrate and enter the systemic circulation, survive, travel/transport through the blood to the brain, adhere to and extravasate through the endothelium, invade the brain parenchyma, and proliferate, which requires the recruitment of a secondary blood supply. Failure at any of these steps will halt the metastatic process. Each of these steps requires complex interactions between the tumor cell and its changing microenvironment.
An understanding of the biological processes of brain metastases and the role of the BBB provides potential targets for intervention to improve treatment. There are multiple complex regulators of cell adhesion, including molecules such as integrins, cadherins, selectins, and heparin sulfate proteoglycans (44). In addition, integrins can recruit intracellular signaling molecules such as focal adhesion kinase and src, which can lead to a cascade of cellular signaling that affects cell cycle control and proliferation. Integrins also play a part in regulating angiogenesis and tumor invasion. Other molecules that mediate invasion include the MMP family, serine proteases, and heparinase (45).
Tumor cells must generate their own blood supply if they are to grow successfully and remain viable. Important activators of angiogenesis include vascular endothelial growth factor (VEGF), angiopoietin, hypoxia-inducible transcription factor (HIF), cyclooxygenase 2 (COX-2), PDGF, integrins, MMPs, and others. Important inhibitors of angiogenesis include angiostatin, endostatin, tissue inhibitors of matrix metalloproteinases (TIMPs), interferons, and platelet factor 4 (46). Upregulation of activators or downregulation of inhibitors favors angiogenesis. As tumors grow, they begin to produce increasing numbers of angiogenic molecules that can participate in metastasis (46). Because a multitude of pathways are involved in tumor growth and invasion, it is likely that if one pathway is inhibited, cells may escape through alternate pathways.
Effective drug delivery through the BBB to tumor cells is a significant obstacle to chemotherapy, both for brain metastases and primary brain tumors. The P-glycoprotein family of transporters actively exports drugs such as anthracyclines, Vinca alkaloids, taxanes, and etoposide (47). The BBB can be breached by circulating cancer cells, which migrate across the BBB without degrading its permeability and proliferate. Once the tumor reaches a size that requires recruitment of new vessels, the BBB is disrupted, which allows imaging of brain tumors with contrast agents. In experimental models, brain metastases smaller than 0.25 mm in diameter are associated with an intact BBB, whereas larger tumors demonstrate BBB permeability (48). Despite the presence of the BBB, studies of drug levels in brain tumors from systemic delivery have demonstrated pharmacologically relevant concentrations of drugs such as etoposide, cisplatin, cytarabine, and methotrexate. Measurements of drug levels in cerebrospinal fluid are not accurate indicators of tissue drug levels and also vary widely depending on the agent (49).
CLINICAL PRESENTATION, DIAGNOSIS, AND PATHOLOGY
Brain tumors are usually diagnosed following presentation with symptoms such as seizure, headache, or focal neurologic deficits. We commonly see high-grade, malignant tumors presenting with headache, which reflects elevated intracranial pressure, and focal neurologic signs, such as weakness or aphasia. Low-grade glial tumors often come to attention with seizure, while other slow-growing tumors, such as meningioma, may be clinically silent and incidentally detected during imaging for an unrelated problem. We use contrast-enhanced MRI, the diagnostic standard, for brain tumor imaging. In addition to its superior sensitivity compared with computed tomography (CT), MRI provides more detailed anatomic as well as physiologic information that can contribute to a differential diagnosis. While contrast-enhanced CT can detect high-grade lesions that cause BBB breakdown, low-grade lesions may be detectable only on MRI, using sequences sensitive for edema and tissue changes. However, even in the case of known systemic primary cancer, contrast-enhanced CT may miss small foci of metastatic disease that are visible on MRI (Figs. 40-2,40-3,40-4,40-5,40-6,40-7,40-8,40-9).
The brain tumor imaging characteristics seen on MRI are helpful in making a diagnosis. However, confirmation of the diagnosis with pathology is necessary in nearly all cases. Noncancerous brain lesions that may be mistaken for malignancy include infection, demyelinating disease, vascular malformations, and stroke. A particular variant of demyelinating disease (tumefactive multiple sclerosis) with large focal tumor-like lesions, is known to resemble a malignant brain tumor. Unfortunately, some of these lesions have been irradiated, under the presumptive diagnosis of GBM, which only increases the severity of the demyelination. Conversely, patients with primary brain tumors are sometimes initially diagnosed with stroke or demyelinating disease. Further complicating the picture are patients who have brain tumors in addition to stroke, which is far more prevalent with increasing age. Patients with systemic cancer, often in remission, or in stable condition, can present with brain lesions that are suspected to be brain metastases but turn out to be a primary brain tumor. These types of cases often benefit from an interpretation by a specialized neuroradiologist who has been provided with a relevant patient history. A history of immunosuppression and multiple subcortical enhancing lesions may prompt the suspicion of a PCNSL or infection with toxoplasmosis. Further testing with brain thallium single-photon emission computed tomography (SPECT) scanning or fluorodeoxyglucose positron emission tomography (FDG-PET) imaging may serve to distinguish between the two possibilities.
Several classic radiographic appearances of brain tumors suggest malignancy. An irregular enhancing lesion with extensive edema following white matter pathways suggests a malignant glioma. Non–contrast-enhancing lesions with increased diffuse signals on FLAIR (fluid-attenuated inversion recovery) imaging suggest a low-grade astrocytoma. As a general rule for glial tumors, the presence of contrast enhancement suggests a high-grade malignancy. The WHO grade IV tumors nearly always enhance, as opposed to grade II tumors, which are typically nonenhancing. Two notable exceptions include pilocytic astrocytoma (WHO grade I) and pleomorphic xanthoastrocytoma (WHO grade II), which typically have an enhancing nodule and associated cyst. Meningiomas are typically homogeneously enhancing dural-based lesions associated with calcification. The appearance of multiple enhancing subcortical lesions with homogeneous enhancement suggests PCNSL. However, the same lesions, if associated with a known primary malignancy, may indicate brain metastases.
The discovery of a brain lesion should prompt referral to a neurosurgeon for consideration of biopsy or resection. The management of brain tumors is critically dependent on a definitive pathology for diagnosis. A referral to a specialized neuropathologist may be necessary for diagnosis of uncommon or rare tumors and also in cases where there is only limited biopsy tissue available. Biopsies must be of adequate quality and be representative of the overall tumor to allow accurate diagnosis. Our institution insists on reviewing patient diagnostic slides prior to rendering a treatment recommendation, and it is not uncommon for our neuropathologists to disagree with the diagnosis provided by the referring physician. Primary brain glial tumors are graded according to the most malignant portion of the tumor. A brain lesion that is predominantly grade III astrocytoma but has a few regions that meet the criteria for grade IV astrocytoma (GBM) should be graded as a GBM. The tumor grade may be underestimated if the most malignant portion of the tumor is not sampled. Typically, the most malignant region corresponds to an area of contrast enhancement. If a patient is suspected of having PCNSL, the use of corticosteroids should be avoided. Primary CNS lymphoma can be sensitive to steroids which are lymphocytolytic during initial presentation, and the preoperative use of even small doses of corticosteroids can lead to a nondiagnostic biopsy. These patients usually require repeat biopsy after steroid discontinuation. Patients may present with deep central lesions involving the brainstem or thalamus. These cases may require referral to a specialized neurosurgical center to evaluate whether open biopsy, resection, or stereotactic-guided biopsy is appropriate. In these cases, close coordination with a department of neuropathology will be critical in obtaining adequate tissue for diagnosis.
Diffuse astrocytomas are characterized by well-differentiated astrocytes—either fibrillary, gemistocytic, or rarely protoplasmic—with mildly increased cellularity. The cellular morphology of the tumor cells may differ within the same tumor sample and show great variability between tumors. Necrosis and microvascular proliferation are absent. Rare mitotic figures may occur, and nuclear atypia may be present, but not sufficiently to characterize the tumor as AA. The typical MIB-1 labeling index is less than 4% (Figs. 40-10 and 40-11).
Oligodendrogliomas are characterized by moderately cellular tumor cells with rounded, homogeneous nuclei, giving a “fried egg” artifactual appearance that is referred to as “classical OD.” A recent RTOG trial found that 80% of tumors with classical oligodendroglial morphology were associated with 1p/19q deletion, compared with only 13% of 1p and 19q deletions seen in nonclassical ODs (50). Microcalcifications, microcyst formation, extracellular mucin deposition, and a dense network of branching capillaries are other oligodendrogliomal features. Nuclear atypia may be seen, but significant mitotic activity or microvascular proliferation is suggestive of an anaplastic tumor. The MIB-1 index is typically less than 5% (Figs. 40-12 and 40-13).
Anaplastic ODs are characterized by oligodendroglial cells, with signs of increased cellularity, nuclear atypia, and mitotic activity. Cellular pleomorphism may be present, with formation of multinucleated giant cells or spindle cells. Gliofibrillary oligodendrocytes and minigemistocytes are common. Although microvascular proliferation and necrosis may be present, their presence does not change the diagnosis to GBM. There is currently no designation for a WHO grade IV OD. The MIB-1 ratio is usually greater than 5% (Figs. 40-14,40-15,40-16.)
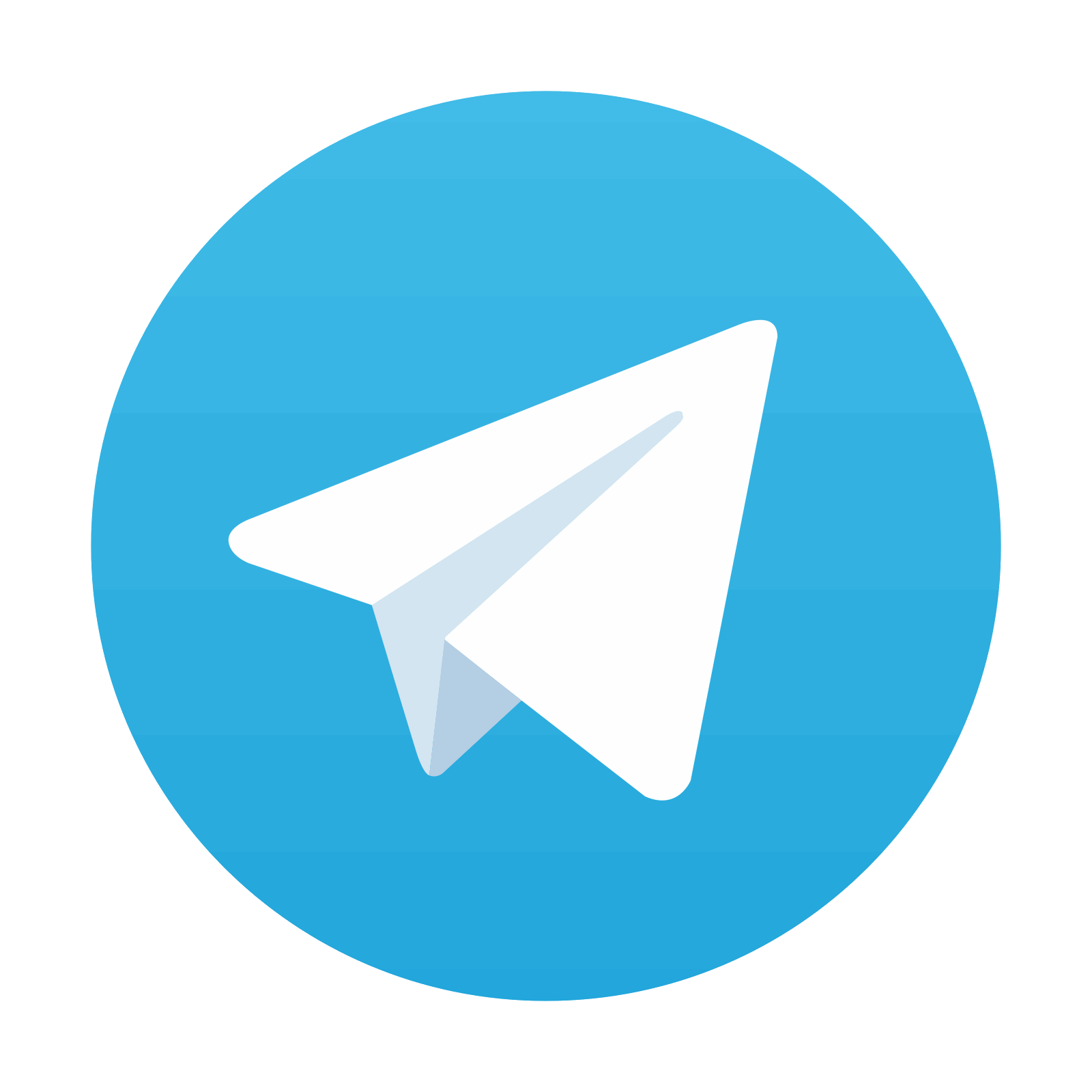
Stay updated, free articles. Join our Telegram channel

Full access? Get Clinical Tree
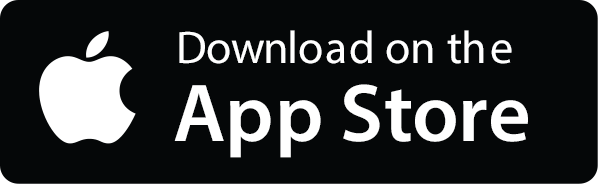
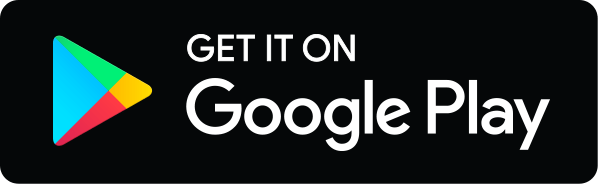