- Islet transplantation is a promising treatment for patients with type 1 diabetes with severe hypoglycemia, hypoglycemia unawareness and/or glycemic lability.
- Successful islet transplantation can lead to insulin independence, but this is usually not maintained in the long term.
- Graft function is preserved for several years beyond the loss of insulin independence, resulting in better glycemic control in spite of a return to insulin use.
- Current immunosuppression protocols include the use of more potent induction agents which may lead to better long-term outcomes.
- The side effects of maintenance immunosuppression are better understood, and appropriate dose titration and/or change to alternative agents have led to fewer and more tolerable side effects.
- The long-term impact of islet transplantation on diabetes-related complications is not fully elucidated, but there appears to be stabilization of microvascular and macrovascular disease.
Introduction
The discovery of insulin dramatically changed the outcome for patients with type 1 diabetes mellitus (T1DM) and the acute lethal complications of diabetes, such as diabetic ketoacidosis, could be effectively treated. The improved survival, however, allowed the development of the secondary complications of diabetes [1]. Typically, the patient with T1DM develops retinopathy, nephropathy, neuropathy or vascular disease over time. It was not until 1993 that definitive proof for the value of good glycemic control was established. The landmark Diabetes Control and Complications Trial (DCCT) study showed that the microvascular complications of diabetes could be delayed or avoided by good glycemic control, which required intensive insulin therapy [2]. Such therapy was associated with a hemoglobin A1c (HbA1c) that was 1% (11 mmol/mol) lower than that of the control group. It should be noted that the HbA1c was not normalized and in the intensively treated group remained at least 1% (11 mmol/mol) above the upper limit of normal. Similar evidence was provided by the UK Prospective Diabetes Study (UKPDS) in T2DM with microvascular disease, showing a significant improvement for a decline of 1% (11 mmol/mol) in the HbA1c [3]. Thus, glycemic control is essential if the microvascular complications of diabetes are to be prevented.
Controlling glycemia with exogenous insulin remains a challenge. Given the non-physiologic subcutaneous route of insulin administration, its inherent delay in absorption, the variability of serum levels obtained and the systemic versus portal venous delivery, it is perhaps remarkable how well the glucose levels are actually controlled. The newer insulin analogs have removed some of the variability and delay in absorption, but even with intensive therapy it is difficult to achieve normoglycemia. Continuous subcutaneous insulin administration helps further, but neither earlier nor more recent studies have managed to normalize glucose values [4,5]. Carbohydrate counting and attention to diet improves the quest for normoglycemia but does not offer a complete solution. The largest study using all available tools to optimize therapy was still associated with an increased risk of hypoglycemia [2]. In the DCCT, the risk for severe hypoglycemia (defined as needing third-party assistance) was increased threefold. Concern about hypoglycemia remains the limiting factor in attempts to achieve optimal glycemic control.
Many efforts have been made to generate a closed-loop system for glucose control. The first successful efforts were with the Biostator®, which involved continuous monitoring of blood glucose and then an insulin infusion using a computer-derived algorithm [6]. Although effective for a short period of time, it was impractical being bulky and plagued with flow problems from the double lumen catheter being used for sensing the glucose level. Insulin infusion pumps especially using insulin analogs (such as lispro or insulin aspart) have helped but are not closed-loop systems and require frequent glucose monitoring and rarely result in normal glycemia without severe hypoglycemia. The goal of any closed-loop system is to have reliable glucose sensing linked to appropriate insulin delivery on a continuous basis. Mechanical closed-loop systems may in the future achieve this but have been fraught with problems despite early promise. Transplantation of islets of Langerhans or of the whole pancreas can achieve a physiologic closed-loop system today.
Background history
Transplantation of pancreatic tissue was first tried in 1890 when a surgeon in England transplanted fragments of a sheep’s pancreas into a boy with diabetic ketoacidosis [7]. The immune barriers, unknown at the time, were insurmountable, especially for a xenograft. The modern era of transplantation began in the 1960s when the use of steroids, especially when combined with azathioprine, allowed successful renal transplantation. The steroids block several cytokines and act as inhibitors of antigen presentation, and azathioprine is an inhibitor of purine synthesis and thus impedes the generation of active T cells. Cyclosporine A (CsA), a calcineurin inhibitor, which is associated with an impaired transcription in active T cells and thus blocks interleukin 2 (IL-2) activation, greatly improved transplantation graft survival [8]. In 1972, Ballinger and Lacy [9] demonstrated that chemical diabetes could be “cured” in mice with islet transplantation.
Many groups had been working in rodent and canine models to perfect islet isolation and maintain demonstrable β-cell insulin secretion [10,11]. Experiments in rats and mice showed that success in islet transplantations could be obtained in rodents [8] and the transplanted islets had a biphasic insulin secretory response to glucose [12]. Islet allotransplantation in animals was also successfully performed [13,14] and it has been possible to isolate human islets that would function in diabetic nude mice [15,16].
The results of these studies highlighted the need for purified islets and the importance of the site of transplantation. Multicellular islet tissue comprises 1.5% by weight of the whole pancreas [17]. Careful digestion is necessary to achieve a purified preparation, and collagenase became the mainstay in the digestion [10]. Success with isolation from human pancreases was more problematic than in animal studies [18,19]. Methods used for the dissociation of islets from the exocrine tissue required a combination of mechanical and enzymatic techniques.
The site of transplantation was also an issue. The pancreatic bed, because of the risk of inducing acute pancreatitis, was not a preferred option. Liver, spleen, renal subcapsule and an omental pouch have been the primary locations tried [14,20–22]. Work with immune privileged sites, such as the testes, has been ongoing but has not been routinely adapted [23]. A site with portal drainage has the natural advantage of mimicking the normal route of insulin delivery and is most commonly used for human islet transplantation [24]. The splenic site was associated with infarction [25] and so the liver, renal and omental pouch have been the preferred areas for islet transplantation. Compared to the native islet bed, all sites have the disadvantages that oxygenation is lower [26] and the intra-islet blood pressure is elevated [27]. Vascularization of the liver is advantageous and may help in the angiogenesis of the islets while an advantage of the omental pouch or renal site is the ability to remove the islets for histologic examination. When the liver is used, an unresolved question is whether the venous drainage of the islets appear in the portal sinusoids or the systemic circulation. A possible drawback of the portal site is that it allows more exposure to high levels of immunosuppressive drugs and their potential toxicity as they are absorbed [28].
Early human studies
The first human islet transplants were performed in the 1970s [29], with insulin independence rarely reported [30]. Up to 1998, approximately 260 patients with T1DM had received an islet transplant, with only 12% remaining insulin independent for more than 1 week [24]. The first two patients, transplanted as part of an early cohort transplanted in Edmonton in 1989 [31]. received approximately 260 000 islets at the same time as a renal transplantation. Exogenous insulin was used intravenously for 14 days with intensive glucose monitoring in order to maintain euglycemia, which may help preserve β-cell function [32]. The immunosuppression therapy included corticosteroids, azathioprine, CsA, and Minnesota antilymphocyte globulin. Both patients demonstrated positive C-peptide status post-transplant, but both developed cytomegalovirus infection and lost islet mass, never achieving insulin independence. The subsequent five patients had transplants using both fresh and cryopreserved islets so that the total islet mass given exceeded 10 000 islet equivalents per kilogram. One patient obtained insulin independence for 2 years [33]. One patient who was transplanted at this time had a liver transplant with partially purified islets infused. Complete portal vein thrombosis ensued necessitating an urgent repeat liver transplant [34]. Another patient attained insulin independence for a period of time but eventually all patients required insulin again. On long-term follow-up, two of these patients have continued C-peptide production, the longest being more than 9 years since her transplant; however, subsequent technical problems arose in Edmonton, particularly with the collagenase, and so purified islets could not be obtained and thus islet transplantation lapsed as an avenue for treating diabetes. Other major centers, particularly Miami/Pittsburg, St. Louis and Milan, also reported early success [35–38] and demonstrated that the transplanted cells may survive for a prolonged time [39,40].
Pancreas transplantation
With improved immunosuppression the possibility of performing whole pancreas transplants, particularly at the time of renal transplantation for end-stage diabetic nephropathy, became a possibility [41]. Initial efforts were associated with peritonitis from exocrine drainage of the pancreatic duct. These problems were surmounted by using bladder drainage [42] such that success rates reached over 80% for 1-year graft survival accompanied by low mortality rates [43–46]. With newer techniques of anastomosis connecting the transplanted duodenum to an enteric drainage site [47], fewer problems were encountered (especially with the acidosis secondary to bicarbonate loss in the urine associated with the bladder anastomosis [48]). More than 25000 whole pancreas transplants have been carried out worldwide. The 1-year graft survival rate has improved as a result of reduction in technical and immunologic failure rates [49]; however, the overall 10-year graft survival rate for deceased donor pancreas transplants has not substantially improved over time and was 48% for transplants between 1995 and 1999 [50]. Graft survival is better for simultaneous pancreas–kidney transplantation than either pancreas transplant alone or pancreas transplantation after kidney transplantation. The side effects are diminishing but it remains a technically challenging surgical procedure [51–53] with some morbidity and mortality. The excellent glycemic control [54] can lead to reversal of diabetic renal lesions [55], stabilization or improvement in neuropathy [56,57], vascular status [58,59] and stabilization but not necessarily improvement of retinopathy [60,61].
Islet transplantation in the new millennium
The unimpressive results of islet transplantation in the late 1990s, as illustrated by low rates of insulin independence [39,62,63], were related to the islet preparation (purity of preparations and adequate islet numbers) [64] and immunosuppression (potency and toxicity especially in terms of glucose tolerance), as has been reviewed by Hering and Ricordi [65]. The field of islet transplantation was rejuvenated with our report of seven consecutive cases, achieving insulin independence with islet transplantation using a steroid-free immunosuppression regimen (Edmonton protocol) [66]. Some of the factors associated with this success are discussed below.
Islet isolation
Islet mass availability remained a central issue for success. An adequate islet mass contributed to the success of the Edmonton protocol, with >11000 islet equivalents per kilogram recipient body weight being transplanted.
The normal pancreas has 1.0–1.7 million islets [67,68], yet early studies showed only 250 000 islets being recovered for allotransplantation. Minimizing warm and cold ischemia time and other donor issues are important [69,70]. Using the University of Wisconsin perfusate solution at the time of organ retrieval enhanced the yield [71]. In addition, the intraductal delivery of collagenase [18,19,72], particularly with improved collagenase preparations [73,74], further enhanced the number of islets obtained. Great strides were made in characterizing the collagenase necessary for purified islet isolation, resulting in much better preparations. The enzyme preparation Liberase was widely used for islet isolation and is a blend of primarily collagenase type 1 and 2, but also has collagenase 3 and 4, clostripain, thermolysin and proteases (trypsin, chymotrypsin and elastase), and is associated with a low endotoxin load [74].
Another major advance was the development of a metal chamber by Ricordi et al. [75] allowing disassociation of islets with mechanical digestion and the continuous removal and harvesting of the liberated islets [76]. Purification of islets using a refrigerated COBE centrifuge and Ficoll gradient is also important. While it leads to some loss of cells, it reduces the otherwise substantial risk of intraportal hypertension associated with the infusion of unpurified preparations [34,77]. The increased purification may also have a negative effect because ductal elements, which may be important as a source for islet neogenesis, are removed [78].
Short-term culture of the islets may lead to enhanced purity without excessive loss of islets. In addition, the newer methods of islet isolation have removed all xenoproteins from the process, and this could further reduce the risk of rejection.
Immunosuppression
The importance of the appropriate immunosuppression regimen is demonstrated by the fact that with autotransplantation once 2500 islet equivalents per kilogram are provided then insulin independence can usually be achieved; however, three or four times this number of cells are required for insulin independence in the allotransplant environment [79]. In the Edmonton protocol, daclizumab was used for induction, followed by maintenance immunosuppression therapy with sirolimus (target trough levels of 12–15 μg/L for 3 months, then 10–12 μg/L) and low-dose tacrolimus (target trough levels of 3–6 μg/L) [66]. Such a combination allowed the omission of steroids from the regimen, which was a major advantage in the setting of borderline islet mass by reducing β-cell toxicity. Effective blockade of IL-2 with sirolimus, which inhibits T-cell expression and activation, and daclizumab, an antibody to IL-2 receptors, allows inhibition of T-cell activation and provides potent immunosuppression. Sirolimus can result in lipid abnormalities but was not known to affect glucose tolerance [80,81]. Tacrolimus, a more potent calcineurin inhibitor than CsA, is associated with some diabetogenicity [9,82–84] by inhibiting insulin release [85] in a dose-dependent manner [86], a problem shared by its predecessor CsA [87,88]. Hence, part of the rationale for using sirolimus as the mainstay of immunosuppression with low dose tacrolimus was to reduce diabetogenicity of the maintenance immunosuppression regimen.
Islet transplantation today
Following this initial success, more than 650 islet transplants have been performed worldwide using the Edmonton protocol or variants of it, and incorporating newer advances. Indeed, islet transplantation today is quite different from 10 years ago.
Islet preparation
Pancreas preservation
The University of Wisconsin solution (UW) was effective in pancreas preservation, but prolonged storage before islet isolation led to reduced recovery of viable islets [89]. The two-ayer system using perflurodecalin (PFC) and UW for whole pancreas preservation was thus advocated for rescuing ischemically damaged pancreases [90]. This was because of the ability to ensure adequate oxygenation to the pancreas during preservation, and the reduction of cold ischemic injury by promoting adenosine triphosphate production [90]. It was also found that preservation in PFC resulted in the upregulation of anti-apoptotic genes and the downregulation of pro-apoptotic genes [91].
Indeed, islet recovery from pancreases preserved with the two-layer system was double that of UW alone [90,92]. Furthermore, PFC preservation resulted in an islet yield from pancreases procured from marginal donors that was sufficient for clinical transplantation in more cases than with UW alone [92]; however, more recently it was shown that there was no significant difference in islet yield or transplantation outcome regardless of whether the two-layer method or UW alone was used [93]. The authors currently use histidine-tryptophan-ketoglutarate solution alone for pancreas preservation, which appears to be equally effective as UW.
Islet culture
Previously, islets were infused into recipients within 2 hours of isolation to reduce the risk of ischemic injury to the islets [66]; however, this gave little time for appropriate quality control measures to be completed and potential recipients had to live near the transplant center. The authors’ current practice is to culture islets for up to 72 hours prior to transplantation. This has numerous advantages because the additional time when the islets are in culture allows for the administration of conditioning or other immunosuppressive therapies, can result in improved safety because transplants can be carried out when the entire transplant team is present and allows for better islet characterization before transplant [94]. In addition, the decrease in total tissue volume with culture may reduce the risk of portal vein thrombosis. Furthermore, the use of regional islet processing centers has been advocated as a means of standardizing the islet product, and hence improving transplant outcomes. Islet culture can result in better islet recovery after shipment [95], and islet culture has now become standard and routine practice at most islet transplant centers worldwide.
Enzyme preparation and digestion protocols
In 2007 it became apparent that the crude collagenase extract in Liberase®, a secretion product of Clostridium histolyticum bacteria, could have been contaminated with bovine brain infusion extract, as this extract contains high levels of lipid, carbon and nitrogen which apparently facilitates the proliferation and secretory capacity of the bacteria. The specific risk to an islet patient is the possibility of prion transmission from the cow brain extract through the enzyme and into the pancreas organ during digestion of the gland. The estimated risk is currently unknown, but a working number is currently less than one in ten million – in other words exceedingly remote. Since then, most islet isolation centers have switched to an alternative enzyme manufactured by Serva. This is a GMP (good manufacturing practice) grade enzyme where the potential risk of prion transmission should be dramatically lower as no bovine brain extracts are used in the manufacture process. Using high-pressure liquid chromatography and collagenase activity assay, we found that the Serva collagenase is less pure and less potent than Liberase. With modification of our digestion protocols, we have now managed to achieve a high rate of islet isolation success (defined as >300000 IEQs,>70% viability and <5mL packed tissue volume) that is superior to historical outcomes from the Liberase era (unpublished data). Specifically, we use different digestion protocols for younger (≤35 years) versus older donors. For younger donors, we use collagenase and neutral protease simultaneously, while for older donors, a higher amount of collagenase initially, followed by sequential digestion with a lower amount of neutral protease was found to be optimal. Others have found that islet isolation outcomes and islet function are similar with the two enzyme blends [96].
Transplant procedure
Once adequate pure islets are prepared the patient is brought to radiology and percutaneous access is established under midazolam and fentanyl sedation. After infiltration of local anesthetic a 22-gauge Cheba needle is advanced under fluoroscopic guidance into the portal vein. Others have used computer tomography (CT) guidance or it is possible to gain access by the transjugular route or with laparoscopy [97]. A guidewire is then inserted into the main portal vein and a catheter is positioned with confirmation by portal venogram. Purified islets are then infused with frequent monitoring of portal pressure. If the portal pressure doubles or rises above 22 mmHg infusion is halted until it resolves, and if it does not resolve the infusion is discontinued. Initially, we used a 60-mL syringe but quickly adopted the use of an intravenous bag which is prepared in the laboratory [98]. This aids aseptic technique and may also pose less shear pressure on the islets and further provides some constant monitoring of portal pressure during islet infusion.
This percutaneous approach can result in the risk of bleeding from the liver, which was seen in the first report. Subsequently, Gelfoam® pledgets and coils were used to seal the catheter tract, and there was no more bleeding seen in the next 28 cases [99]; however, in 2003, there was a spate of post-procedural bleeding (defined as an acute fall in hemoglobin of 20%, associated with free fluid on ultrasound, the need for blood transfusion or surgical intervention for control of bleeding) [99,100]. Since then, the portal tributary cannulation site was plugged with coils, and the tract ablated using tissue glue (Tisseel®) with no further recurrence of bleeds in the next 35 procedures [100]. More recently, Avitene® paste dissolved in radiologic contrast and saline has been used instead to seal the catheter tract. When adequately deployed, this has eliminated bleeding risks and has the practical advantage of being clearly visible during deployment on fluoroscopy.
Before transplant, intravenous insulin and dextrose infusions are started to maintain euglycemia during transplant. Initially, insulin was discontinued after transplantation and was avoided unless hyperglycemia (serum glucose ≥11.1mmol/L) occurred [66]. Subsequently, this threshold was lowered and insulin was given if pre-meal glucose was > 6.0 mmol/L or 2-hour post-meal glucose was >8.0mmol/L [99].
Recently, it was shown that maintaining euglycemia in the immediate post-transplant period could contribute to better graft survival [101]. Since mid 2005, it has been our policy to maintain euglycemia (serum glucose 4.0–7.0 mmol/L) following transplant by using intravenous insulin (minimum of 1 unit/hour) with dextrose infusions for the first 48 hours, and subcutaneous insulin thereafter.
Intravenous heparin is also infused to keep the pro-thrombin time 70–90 seconds for 48 hours after transplantation to promote engraftment by reducing the immediate blood mediated inflammatory reaction (IBMIR). Heparin is withheld if there is inadequate tract plugging with Avitene (<3 cm in length) until imaging confirms the absence of bleeding.
After the heparin infusion is discontinued, subcutaneous low molecular weight heparin (30 mg enoxaparin twice daily) is administered for 7 days and 81 mg/day aspirin for 14 days.
In addition, patients receive 400 mg sulfamethozazole–80mg trimethoprim one tablet daily for 6 months, Pneumocystis pneumonia prophylaxis and 900mg/day valganciclovir for 14 weeks when there is cytomegalovirus status mismatch between donor and recipient, and in patients who have received lymphocyte-depleting induction agents.
Immunosuppression
The immunosuppressive regimen of the original Edmonton protocol is still being used today, but with some modifications. Daclizumab was initially given at a dosage of 1 mg/kg every 2 weeks five times. After 2003, this was changed to 2 mg/kg at transplant and at 5 days post-transplant [99]. This was because the latter regimen was found to be efficacious and was more convenient for patients.
Recently, induction with antithymocyte globulin (6 mg/kg) and etanercept, with maintenance immunosuppression with tacrolimus (target trough level 8–10 μg/L) and mycophenolate mofetil (1 g twice daily), has been used [100]. Also, a lymphocyte depletion protocol consisting of alemtuzumab (Campath-1H), tacrolimus and mycophenolate mofetil is being evaluated. Preliminary data suggest that the use of these potent induction agents have improved short to medium-term graft outcomes [101–103].
Many of the initial patients who were on sirolimus and tacrolimus for maintenance immunosuppression have had intolerable side effects which were attributed to sirolimus, necessitating a switch of immunosuppression to tacrolimus and mycopheno-late mofetil. This latter combination appears to be as efficacious and better tolerated [104]. Furthermore, sirolimus impairs β-cell regeneration, and could contribute to the observed gradual loss of graft function seen following islet transplantation [105]. Thus, this combination is increasingly the first-line maintenance immunosuppression choice for islet transplantation.
Islet transplantation outcomes
Glycemic control
Insulin independence was achieved in 11 out of the first 12 patients, after a minimum of 9000 IE/kg were transplanted [66]. HbA1c levels improved in all patients, and this was achieved without hypoglycemia and was accompanied by an improved stability of glucose control [66]. Unfortunately, insulin independence was not sustainable in the long term for the majority of patients. From survival analysis, only approximately 10% of patients remained off insulin at 5 years, although most patients (approximately 80%) still had C-peptide present (Figure 61.6) [99].
In terms of overall blood glucose control, patients who remained off insulin did the best (median HbA1c of 6.2%, 44 mmol/mol), similar to the patients who were back on insulin but still had C peptide (median HbA1c of 6.7%, 50mmol/mol). Patients who had lost all graft function had relatively poor glucose control (median HbA1c of 9.0%, 75 mmol/mol) and required more insulin than before the transplant (Figure 61.7) [99].
The HYPO score and lability index (LI) were developed to measure the severity of hypoglycemia and glycemic lability. The HYPO score is generated using a combination of 4 weeks of self glucose monitoring results and the patients’ self-reported hypoglycemic episodes over the previous year. For every episode of hypoglycemia <3 mmol/L during the 4 weeks, patients were instructed to record all symptoms felt and whether assistance was required for recognition or treatment of the hypoglycemia. Higher scores were given for more severe hypoglycemic episodes (i.e. lower glucose values, absence of symptoms or neuroglycopenic symptoms, needing outside help for recognition/treatment), while from the self-reported episodes during the past year higher points were awarded if an ambulance was called or glucagon given. The LI was also calculated from the 4 weeks of glucose records, using a formula that takes the number of glucose readings, the glucose values and the time interval between testing into account [106]. These scores are now routinely used in the assessment of suitability of a candidate for transplant, as well as for follow-up of patients after transplant. In addition, the Clarke score [107] is also frequently used, with a score of four or more indicating hypoglycemia unawareness.
Figure 61.6 Current survival analysis for insulin independence and graft function as indicated by presence of C-peptide.
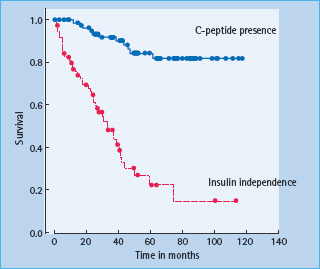
Both the HYPO score and LI show marked improvement post-transplant. With resumption of insulin use, there was more lability and some episodes of hypoglycemia, but both scores were still better than pre-transplant [99].
A key indication for islet transplantation is hypoglycemia unawareness. Patients who have received a pancreas transplant have restoration of their counter-regulatory response to hypoglycemia [108,109]. The autonomic response and hence hypoglycemia awareness is also improved post-pancreas transplant [110]; however, the same restoration in counter-regulatory responses and symptom recognition was not seen following successful islet transplantation [111]. Conversely, others have found that counter-regulatory hormonal and symptom responses to hypoglycemia do improve after islet transplantation [112–114]. The reasons for these differences are unclear; however, there may be a subset of patients who have return of hypoglycemia awareness, and/or the timing of testing could be critical. Indeed, we have noted that 85% of our cohort of islet transplant recipients reported return of symptoms of hypoglycemia, although 62% of them subsequently lost hypoglycemia awareness.
Diabetes complications
Retinopathy
Retinopathy has been reported to remain stable following islet transplantation [115,116]. The majority of our patients (approximately 80%) showed either no change or an improvement in retinopathy grade compared with baseline 3 years following islet transplantation; however, in our cohort, 18 of 98 (18.4%) subjects had either vitreous hemorrhage or need for laser photocoagulation after islet transplantation, suggesting that close ophthalmologic follow-up remains necessary.
Figure 61.7 Glycemic control is related to islet graft function. DCCT result (%) = (0.0915 × New IFCC result in mmol/mol) + 2.15.
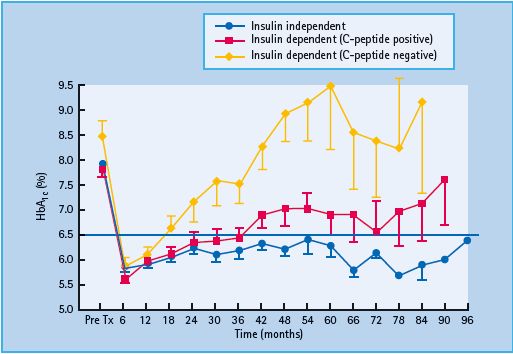
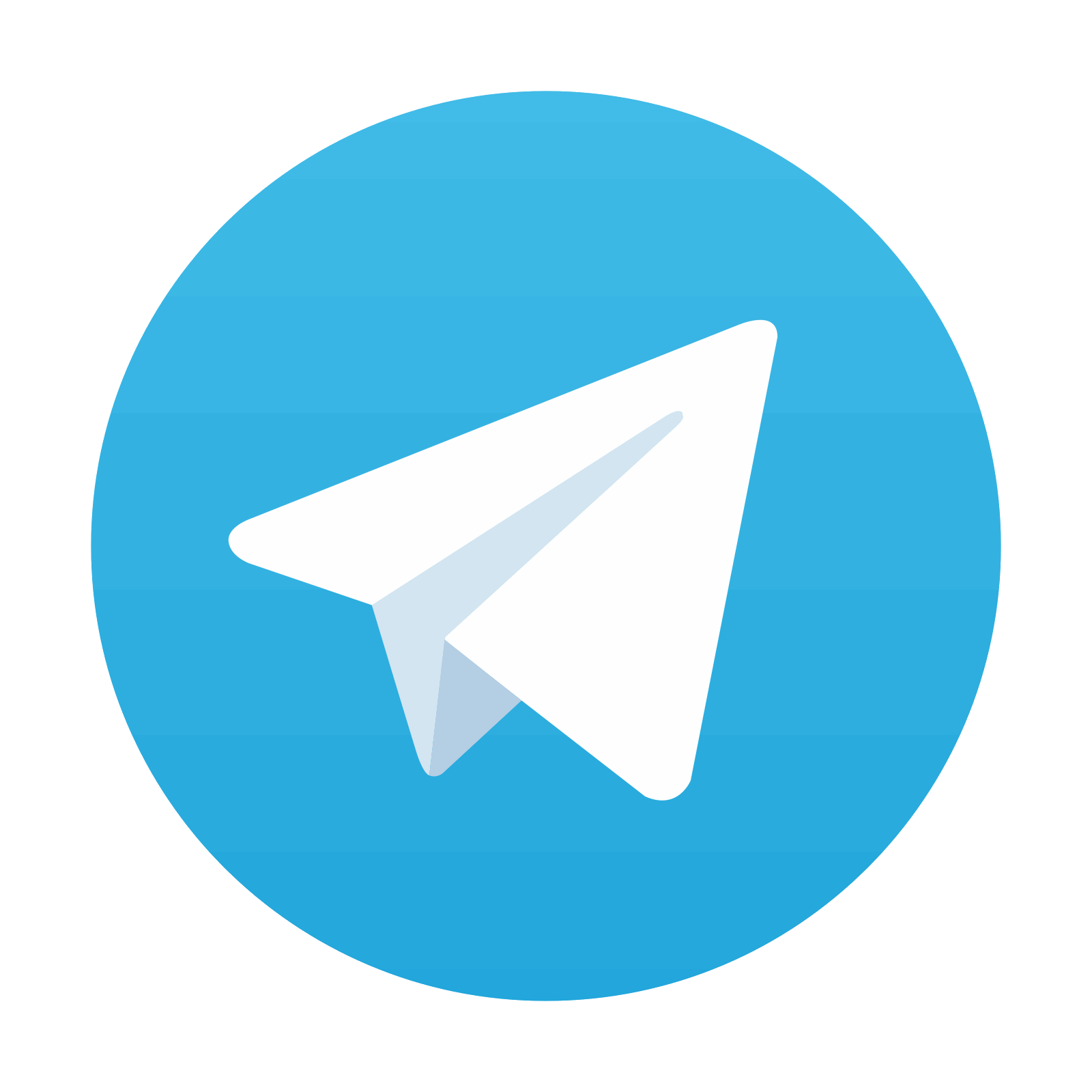
Stay updated, free articles. Join our Telegram channel

Full access? Get Clinical Tree
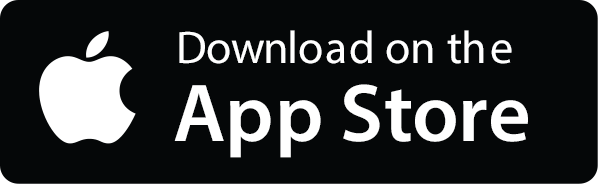
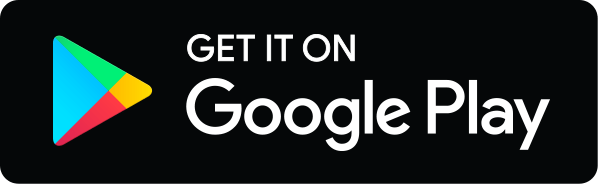