Fig. 1
The tumor microenvironment is a heterogeneous and complex system of tumor cells and ‘normal’ stromal cells, including endothelial cells and their precursors, pericytes, smooth-muscle cells, and fibroblasts of various phenotypes, located within the connective tissue or extra-cellular matrix (e.g. collagen). Leukocyte infiltration is an important characteristic of lung cancer and the main components of these infiltrates include natural killer (T) cells (NK/NKT), N1/N2 neutrophils, B- and T-lymphocyte (cytotoxic T cells [CTL]; T helper 17 [Th17]; CD4; regulatory T cells [Treg]; gamma delta T cells) subsets, myeloid derived suppressor cells (MDSC), M1/M2 macrophages (MØ), mast cells, and immature dendritic cells (iDC)/mature dendritic cells (mDC). Based on their functions, these cells can be divided into cells with a potentially positive impact on the antitumor response (right) and cells with a detrimental effect (left). The net effect of the interactions between these various cell types and their secreted products within the environment of an established tumor participates in determining anti-tumor immunity, angiogenesis, metastasis, overall cancer cell survival and proliferation
Natural Killer (T) Cells
Natural killer (NK) cells (expressing the surface markers CD16 and CD56, but not CD3) are lymphocytes that play an important role in the rejection of tumors without previous sensitization and without restriction by the major histocompatibility complex (MHC) [7, 8]. NK cells eradicate tumors through multiple killing pathways, including direct tumor cell killing. They also secrete cytokines and chemokines like interleukin (IL) IL-10, tumor necrosis factor (TNF)-α, and the principal NK-derived cytokine interferon (IFN)-γ, which can coordinate the innate and adaptive immune responses to tumor cells and may lead to apoptosis of the attacked cells. A large cohort study showed that an increase in NK cells in tumor tissue is a strong independent prognostic factor for the survival of lung cancer patients [9]. Natural killer T (NKT) cells (CD16+, CD56+, CD3+) are a subset of NK cells that are found in the peripheral blood, tumor tissue and pleural effusions of lung cancer patients in decreased numbers and with reduced functions [10, 11]. It has been shown that NKT cells in cancer patients produce a decreased amount of IFN-γ and are therefore less effective than NKT cells in healthy controls [12, 13].
Mast Cells
Accumulation of mast cells is common in angiogenesis-dependent conditions, like cancer, as mast cells are major producers of proangiogenic molecules as vascular endothelial growth factor (VEGF), IL-8, transforming growth factor (TGF)-β [14]. Mast cells also play a central role in the control of innate and adaptive immunity by interacting with B and T cells (in particular regulatory T cells) and dendritic cells. The density of mast cells in non-small cell lung cancer (NSCLC) tumors is correlated with microvessel density [15] and mast cells/histamine has a direct growth promoting effect on NSCLC cell lines in vitro [16]. Tumor-infiltrating mast cells can directly influence proliferation and invasion of tumors, by histamine, IL-8 and VEGF while the production of TNF-α and heparin can suppress tumor growth [16, 17]. It has been shown that in NSCLC mast cell counts were enhanced as tumor stage increased while another study did not found this correlation [14, 18]. The controversy of mast cells in other cancer types seems to be related to the type, microenvironment and stage of cancer and their role may depend on the tumor environment [19, 20].
Neutrophils
Neutrophils play a major role in cancer biology. They make up a significant portion of the infiltrating immune cells in the tumor and the absolute neutrophil count and the neutrophil to lymphocyte ratio in blood are independent prognostic factors for survival of NSCLC [21–23]. Neutrophils are attracted to the tumor under the influence of specific chemokines, cytokines and cell adhesion molecules. Tumor-associated neutrophils (TAN) have polarized functions and can be divided into the N1 and N2 phenotype in a context-dependent manner [24]. The N1 phenotype inhibits tumor growth by potentiating T cell responses while the N2 phenotype promotes tumor growth [25]. The antitumor activities of N1 neutrophils include expression of immune activating cytokines (TNF-α, IL-12, GM-CSF, and VEGF), T cell attracting chemokines (CCL3, CXCL9, CXCL10), lower expression of arginase, and a better capacity of killing tumor cells in vitro. N2 neutrophils support tumor growth by producing angiogenic factors and matrix-degrading enzymes, support the acquisition of a metastatic phenotype, and suppress the anti-tumor immune response by inducible nitric oxide (NO) synthase and arginase expression [26]. Neutrophils also influence adaptive immunity by interacting with T cells [27], B cells [28], and DC [29]. In resectable NSCLC patients, intratumoral neutrophils were elevated in 50 % of the patients and this was associated with a high cumulative incidence of relapse [30].
B Lymphocytes
B cells may affect the prognosis of patients with lung cancer , as patients with stage I NSCLC contain more intratumoral germinal centers with B lymphocytes than patients with stages II to IV [31]. These tertiary (T-BALT) structures provide some evidence of an adaptive immune response that could limit tumor progression in some patients. For instance, the production of antibodies by B cells can activate tumor cell killing by NK cells and other inflammatory cells [32]. Auto-antibodies against tumor antigens are commonly found in patients with lung cancer [33–35] and can inhibit micrometastasis [36]. The role of B cells seems depending on the context.
T Lymphocytes
Several subsets of T cells have been identified, and they all develop in the thymus from common precursor T cells. Based on cytokine secretion and function, these cells are classified as CD4, CD8, Th17, regulatory T cells (Tregs), or TH17 cells, amongst others. CD4+ cells and CD8+ cells represent the strong effectors of the adaptive immune response against cancer [37]. There is controversy on the impact of T cells and their localization on the prognosis of lung cancer [38–43]. This may be caused by the presence of a special subset of T cells, the regulatory T cells (Tregs), and myeloid-derived suppressor cells which are both discussed below. Also tumor-derived factors can exhaust T lymphocytes or induce their apoptosis [44]. Recently it has been shown that cytotoxic T lymphocytes (CTL) within the tumor (the tumor-infiltrating lymphocytes [TIL]) are of beneficial prognostic influence in resected NSCLC patients in both adenocarcinoma [45] and squamous cell carcinoma [46].
Tregs, characterized by the expression of CD4, CD25, Foxp3, but absence of CD127, are T lymphocytes that are generated in the thymus (natural Treg) or induced in the periphery (induced Treg) when triggered by suboptimal antigen stimulation or stimulation with IL-35, TGF-β and IL-10 [47]. Tregs are further characterized by the expression of glucocorticoid-induced TNF-receptor-related-protein (GITR), lymphocyte activation gene-3 (LAG-3), and cytotoxic T-lymphocyte-associated antigen 4 (CTLA4). In cancer patients, Tregs confer growth and metastatic advantages by inhibiting anti-tumor immunity. They have this pro-tumoral effect by promoting tolerance via direct suppressive functions on activated T-cells or via the secretion of immunosuppressive cytokines such as IL-10 and TGF-β [48, 49]. Tregs are present in tumor tissue [50, 51] and increased in peripheral blood of NSCLC patients compared to healthy controls [52, 53]. This increase in Tregs was found to promote tumor growth and was correlated with lymph node metastasis [54, 55] and poor prognosis [50, 56]. Many factors can increase Tregs in NSCLC tumors, among them are thymic stromal lymphopoietin (TSLP) [57] and intratumoral cyclooxygenase-2 (COX-2) expression [58]. Tregs are considered the most powerful inhibitors of antitumor immunity [59].
Th17 cells are a subpopulation of CD4+ T helper cells that are characterized by the production of interleukin-17 (IL-17, also known as IL-17A). IL-17 plays an important role in the host defenses against bacterial and fungal infections by the activation, recruitment, and migration of neutrophils [60, 61]. In vitro experiments have shown that IL-1β, IL-6, and IL-23 promote Th17 generation and differentiation from naïve CD4+ T cells [62]. Among the other cytokines secreted by Th17 cells are IL-17 F, IL-21, IL-22, and TNF-α. The role of Th17 cells in cancer is poorly understood. Th17 cells accumulate in malignant pleural effusion from patients with lung cancer [62]. Also higher levels of IL-17A were detected in serum and in tumor lesions of lung adenocarcinoma patients, indicating a potential role of these cells in cancer [63]. It has been shown that Th17 cells encouraged tumor growth by inducing tumor vascularization or enhancing inflammation, but other studies revealed also opposite roles for Th17 cells. Recent data indicate that IL-17 may play a role in the metastasis of lung cancer by promoting lymph-angiogenesis and is therefore an independent prognostic factor in both overall and disease-free survival in NSCLC [64]. So, it is controversial whether Th17 cells in cancer are beneficial or antagonistic; this may be dependent on the tumor immunogenicity, the stage of disease, and the impact of inflammation and angiogenesis on tumor pathogenesis [65].
Myeloid-Derived Suppressor Cells
Myeloid-derived suppressor cells (MDSCs) are a heterogeneous population of immature myeloid cells and myeloid progenitor cells. MDSCs inhibit T cells activation [66, 67] in a nonspecific or antigen-specific manner, alter the peptide presenting ability of MHC class I molecules on tumor cells [68], influence B cells [69], block NK cell cytotoxicity [70–72], inhibit dendritic cell differentiation [73], and expand Tregs [74, 75] signifying their crucial contribution in constituting a tumor suppressive environment. Furthermore, there is compelling evidence that MDSC, by secreting MMP9 and TGF-β1, are also involved in angiogenesis, vasculogenesis, and metastatic spread [76]. MDSCs suppress the immune system by the production of reactive oxygen species (ROS), nitric oxide (NO), peroxynitrite and secretion of the cytokines IL-10 and TGF-β [77]. Upregulated arginase-I activity by MDSCs depletes the essential amino acid L-arginine, contributing to the induction of T cell tolerance by the down regulation of the CD3ζ chain expression of the T cell receptor [78–81]. However, the mechanisms that are used to suppress the immune responses are highly dependent on the context of the tumor microenvironment [82]. An increased subpopulation of MDSCs in the peripheral blood of NSCLC patients was detected that decreased in those patients that responded to chemotherapy and patient undergoing surgery [83].
Tumor–Associated Macrophages
Macrophages are part of the innate immune system and play important roles in the first line of defense against foreign pathogens. They can be divided into M1 macrophages (classical activation) and M2 macrophages (alternative activation). M1 macrophages attract and activate cells of the adaptive immune system and have anti-tumor and tissue destructive activity, while the M2 phenotype has been linked to tumor-promoting activities by subversion of adaptive immunity, promoting tumor angiogenesis and supporting cancer cell survival, proliferation, invasion and tumor dissemination. Macrophages in tumors are usually referred to as tumor-associated macrophages (TAM) and their presence can be substantial (10–65 % of the tumor stroma). In the beginning, the TAM mainly consist of M1-like macrophages however, when the tumor starts to invade and vascularize, there is a skewing towards the M2 phenotype [84, 85]. This takes place especially at those regions in the tumor that are hypoxic [86]. It has been reported by several groups that there is an association between the number of tumor islet macrophages and NSCLC survival [87–91]. Moreover, when looking at the different phenotypes of TAM (M1 and M2), it is shown that high numbers of M1 macrophages infiltrating the tumor are correlated with improved survival. On the other hand, the presence of M2-like macrophages is associated with poor clinical outcome.
Dendritic Cells
Dendritic cells (DC) are widely acknowledged as the central surveillance cell type and play an important role in the activation of lymphocyte subsets to control or eliminate human tumors. Upon encountering tumor cells or tumor-associated antigens, DC engulf this material and begin migrating via lymphatic vessels to regional lymphoid organs. The density of immature DC (Langerhans cell and interstitial DC) and mature DC, present in the tumor microenvironment, is highly predictive of disease-specific survival in early-stage NSCLC patients [92] and the presence of DC in resected NSCLC material is a good prognostic factor [9]. Interaction between the DC and tumor cells results in the release of anti-tumor cytokines [93, 94]. This suggests that DC within the tumor microenvironment of early-stage NSCLC are capable in initiating adaptive immune responses in situ [95–97]. In the peripheral blood and regional lymph nodes of lung cancer patients, the number and function of mature DC is dramatically reduced [98, 99], partly due to abnormal differentiation of myeloid cells (e.g. MDSC) [100]. Tumor cells, stromal cells like fibroblasts, and tumor-infiltrating immune cells and/or their secreted products, like VEGF, M-CSF, IL-6, IL-10, and TGF-β are also responsible for systemic and local DC defects [101–104]. Affected DC are impaired in their ability to phagocytose antigen and to stimulate T cells, leading to a defective induction of anti-tumor responses. NSCLC-derived DC produce high amounts of the immunosuppressive cytokines IL-10 and TGF-β [105]. It has been shown that the T cell co-inhibitory molecule B7-H3 and programmed death receptor-ligand-1 (PD-L1) are upregulated on tumor residing DC and these molecules conveys mainly suppressive signals by inhibiting cytokine production and T cell proliferation [106, 107].
Context-Specific Nature of Immune Cells in Lung Tumors
From the above it is apparent that different types of tumor-infiltrating immune cells have different effects on tumor progression. Based on their functions, immune types can be divided into cells with a potentially positive impact on the antitumor response and cells with detrimental effects, but cell phenotypes can adapt on the changing environment (e.g. the macrophage M1/M2 phenotype polarization). The net effect of the interactions between all these various cell types and their secreted products within the environment of an established tumor participates in determining anti-tumor immunity, angiogenesis, metastasis, overall cancer cell survival and proliferation. These immune infiltrates are heterogeneous between tumor types, and are very diverse from patient to patient. The importance of these tumor-infiltrating immune cells for tumorigenesis, and their secreted chemokines and cytokines, was recently acknowledged by revisiting the hallmarks of cancer as described by Hanahan and Weinberg in 2000 and now include “tumor promoting inflammation” and “avoiding immune destruction” [108, 109]. Besides the type and density of the cells, also the location in the tumor (in the centre or core; or within the invasive margins of the tumor), in adjacent tertiary or secondary lymphoid structures or their presence in peripheral blood will shape the immune contexture. Histopathological analyses of the location, density and functional orientation of the different immune cell populations in large annotated collections of human lung tumors has identified a good association of effector T-cells (CD3 + CD8+), memory T cells (CD3 + CD45RO+) [38, 88, 110–112], and Th1 cells (IL-2 and IFN-gamma secreting CD3+ cells) [110] with longer disease-free survival and/or a better overall survival while Th17 [64, 110] and Tregs [56, 110, 113, 114] have a poor association with the prognosis [115, 116]. However, it is important to realize the complex spatiotemporal dynamics in the tumor-immune interactions in time due to perturbations at the gene and protein level of the immune cells and tumor cells within the microenvironment [116]. For example, when myeloid cells are attracted to the tumor, they are influenced by several signals able to shape the new cells as “needed” by the tumor. In an early phase of tumor development, the tumor-associated macrophages mainly consist of an M1-like phenotype and later in the tumorigenic process, when the tumor changes its local environment, there is a skewing towards the M2 phenotype [84, 85, 117]. This takes place especially at those regions in the tumor that are hypoxic (Fig. 2) [86, 118]. A subpopulation of TAMs gather in hypoxic sites in the tumor as a result of chemoattractants produced by tumor cells [119]. Exposure to hypoxia stimulates TAMs to acquire a pro-angiogenic M2 phenotype with high production of pro-angiogenic factors like VEGF and MMP-9 [120]. This preferential polarization is also a result of the absence of M1-orienting signals, such as IFN-γ or bacterial components in the tumor environment as well as the presence of M2 polarization factors. Thus the cancer immunoediting concept as described by Schreiber et al. [121], that comprises editing, equilibrium and escape, takes place continuously and this plasticity is a major source of heterogeneity between patients and a cause for therapy resistance [4].
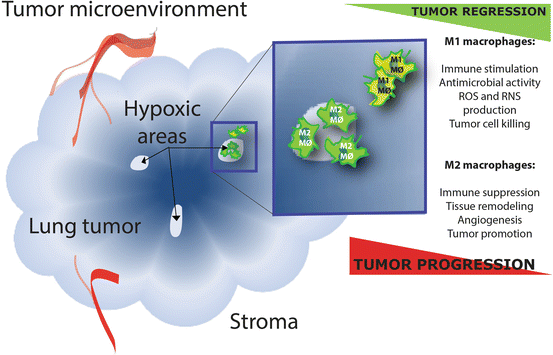
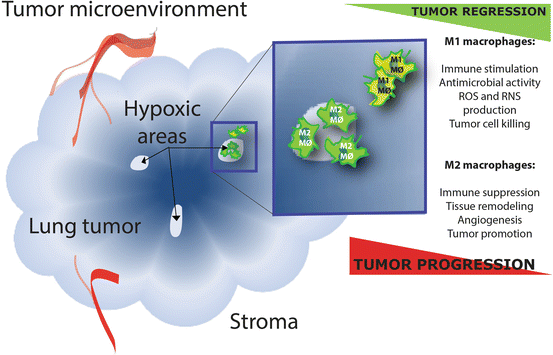
Fig. 2
Opposing effects of M1 and M2 tumor-associated macrophages (TAMs) in the tumor environment. ROS: reactive oxygen species and RNS: reactive nitrogen species. The classically activated M1 macrophages and alternatively activated M2 macrophages are at the opposing ends of the polarization continuum and have opposing effects in tumor biology. M2 TAMs can be abundantly present within tumors, especially in those areas that are oxygen deprived
Types of Immunotherapeutic Approaches
Immunotherapy attempts to stimulate or restore the body’s natural ability of the immune system to fight cancer. There are various strategies to activate the immune system and these are classified earlier by Aerts et al. and here into the following categories: biological response modifiers, monoclonal antibodies, peptide or tumor cell vaccines, and cellular immunotherapy (Fig. 3 and Table 1). There is no consensus regarding which of the four categories is the optimal approach for thoracic malignancies, this will probably be highly dependent on the tumor characteristics of each individual patient.
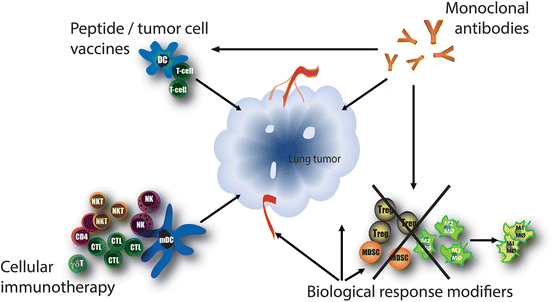
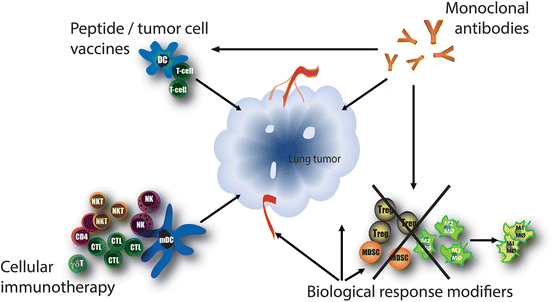
Fig. 3
Immunotherapeutic approaches. Different immunotherapeutic approaches are currently being developed for the treatment of lung cancer. All approaches aim to elicit an anti-tumor immune response and they can often work complementary and/or synergistically. Biological response modifiers can nonspecifically enhance the immune response, either by directly stimulating the immune system and/or by the direct induction of tumor cell apoptosis. Monoclonal antibodies bind specifically to one epitope and can be directed against numerous tumor- or immune cell related targets. Tumor vaccines are designed to deliver tumor antigens to antigen-presenting cells, which can subsequently induce a tumor specific immune response. Cellular immunotherapy includes the adoptive transfer of autologous or allogeneic activated immune cells
Table 1
Immunotherapeutic approaches that have been tested or are currently under investigation for lung cancer and mesothelioma
Approach types | Examples of clinical studies in lung cancer and mesothelioma | Mode of action | References |
---|---|---|---|
Biological response modifiers | |||
Triggering inflammation | PF-3512676 (CpG 7909) CpG-ODN 2006 Bacillus Calmette-Guerin (BCG), Mycobacterium vaccae (SRL172) | Toll-like receptor 9 agonist Downregulation of Tregs Nonspecific immune stimulants now often tested as adjuvants | [218] [219] |
Cytokine therapy | IL-2 + tumor necrosis factor alpha (TNF-α) or interferon alpha (IFN-α) Interferon gamma (IFN-γ) Mda-7 (IL-24) | Induces T-cell proliferation Induces tumor cell apoptosis Mda-7/IL-24 induces tumor cell apoptosis and inhibits tumor angiogenesis | [220] |
Colony-stimulating factors | Granulocyte colony-stimulating factor (G-CSF) | Treatment of chemotherapy-induced neutropenia | |
Multi-modal effectors | Multi-target VEGFR: thalidomide and analogues such as lenalidomide and pomalidomide Cyclophosphamide Cyclosporine Denileukin Diftitox Talactoferrin Trabectedin (Yondelis) All-trans-retinoic acid (ATRA) | Targets regulatory T-cells [225] Targets regulatory T-cells [226] and cancer cells which express the IL-2 receptor Recombinant human lactoferrin, promotes innate and adaptive immunity against tumor cells in the gut-associated lymphoid tissue [142, 227] Targets tumor-associated macrophages [228] and tumor cells Targets myeloid-derived suppressor cells (MDSCs) | [223] [231] [232] [237] |
Monoclonal antibodies | |||
Directed against tumor cells | Cetuximab Panitumumab Matuzumab Necitumab Trastuzumab (Herceptin) CAT-5001 (SS1P) Amatuximab (MORab-009) | Chimeric or fully humanized antibodies which target the epidermal growth factor (EGF) receptor on tumor cells Anti-HER2, targets tumor cells which overexpress the human epidermal growth factor 2 (HER2) protein Anti-mesothelin immunotoxin, targets mesothelin expressed in malignant mesothelioma and lung adenocarcinoma Chimeric anti-mesothelin monocloncal antibody | [242] [149] |
Directed against tumor products | Bevacizumab | Slows the growth of tumor blood vessels by targeting the VEGF protein. Blockade of VEGF is also immunomodulatory | |
Immune checkpoint inhibitors | Anti-CTLA-4 (Ipilimumab/tremelimumab) Anti-PD-L1 (BMS-936559/MPDL-3280A) /PD-1 (BMS-936558 (nivolumab)/MK3475 (lambrolizumab)) | Prevents T cell inhibitory mechanisms and allows T cells to continue cancer cell destruction | |
Peptide or tumor cell vaccines | |||
Vaccines | GVAX Belagenpumatucel-L (Lucanix) MAGE-A3 vaccine (L)-BLP-25 anti-MUC-1 (Stimuvax) TG4010 CimaVax EGF WT1 peptide vaccine CRS-207 Bec2/BCG GV1001 Racotumomab (Vaxira) Tergenpumatucel-L | GM-CSF gene-transfected tumor cell vaccine Allogeneic tumor cell vaccine made with four irradiated NSCLC cell lines modified with TGF-β2 antisense plasmid Vaccine composed of MAGE-A3 protein and adjuvant AS15 Vaccine which targets MUC-1 expressed on tumor cells Vaccinia vector coding MUC1 and IL-2 Vaccine composed of human recombinant Epidermal Growth Factor (EGF) conjugated to a carrier protein Vaccine composed of four WT1 (Wilms’ tumor suppressor gene) analogue peptides Live-attenuated Listeria monocytogenes vector encoding human mesothelin [148] Induces anti-GD3 antibodies (overexpressed on 60 % of SCLC patients) [245] Vaccine which targets the telomerase peptide GV1001 Anti-idiotypic antibody which mimicks the NGcGM3 ganglioside that is expressed on multiple human cancers [246] Vaccine composed of irradiated and gene-transfected lung cancer cell lines | [166] [168] [169] [182] [173] [250], ClinicalTrials.gov: NCT01460472 ClinicalTrials.gov: NCT01774578 |
Cellular immunotherapy | |||
Dendritic cells (DCs) T-cells Natural Killer (NK) cells | Ex-vivo generated DC-vaccines Ex-vivo generated lymphokine-activated killer cells (LAK) Cytokine-induced killer cells (CIK) Activated T-cells Gamma delta T cells NK cells | Dendritic cells loaded with tumor antigens Autologous lymphokine-activated killer cells [251] Autologous cytokine-activated T-cells and NK cells [252] Adoptive transfer of activated T-lymphocytes Adoptive transfer of zoledronate expanded gamma delta T-cells Adoptive transfer of allogeneic Natural Killer (NK) cells [253] | [200] [201] [203] [204] |
Biological Response Modifiers
Biological response modifiers are compounds which can nonspecifically enhance the immune response, either by directly stimulating the immune system and/or by the direct induction of tumor cell apoptosis. These compounds can activate the anti-tumor immune response via the direct stimulation of pro-inflammatory immune cells or via the inhibition of detrimental suppressive immune cells like Tregs or MDSCs.
The observation that lung cancer patients who developed an empyema after pneumonectomy seemed to have a longer survival gave rise to studies involving different biological response modifiers in the 1970s [122]. The idea that bacterial infection in the area of the draining lymph nodes of the resected tumor could lead to immune destruction of residual tumor cells provoked studies involving the intrapleural injection of bacterial antigens to induce immune activation. Bacillus Calmette-Guèrin (BCG) is a vaccine against tuberculosis that is prepared from a strain of attenuated live bovine tuberculosis bacillus and its potential for cancer immunotherapy has been thoroughly investigated. McKneally et al. were the first to study the effect of postoperative injection of BCG into the pleural space of early stage lung cancer patients [123]. Their observation that intrapleural BCG injection resulted in an improved survival lead to numerous studies regarding nonspecific immune stimulation with this vaccine. Currently, BCG is most often investigated as an adjuvants instead as a single therapeutic agent in lung cancer patients [124]. In contrast, in patients with superficial bladder cancer, the use of intravesical BCG is now well-established [125]. In addition to BCG, heat-killed mycobacterium vaccae (SRL 172) has been investigated as a nonspecific immunostimulant in lung cancer and mesothelioma patients in combination with chemotherapy [126, 127], unfortunately no survival benefits were reported. Mycobacterial adjuvant-based agents have been shown to activate antigen-presenting cells and induce a Th1-type immune response, partly due to the binding of components of the cell wall of Mycobacteria to Toll-like receptors (TLRs) [128]. TLRs are membrane glycoproteins and belong to a family of pattern recognition receptors (PRRs) that recognize specific microbial molecular structures, pathogen associated molecular patterns (PAMPs). Recognition of a PAMP belonging to a micro-organism by a TLR leads to activation, maturation and induction of proinflammatory cytokines. Immature dendritic cells express numerous TLRs and nonspecific immune activation via the stimulation of these TLRs has been extensively researched. In lung cancer specifically, TLR9 plays an important role and has been described to be overexpressed in lung cancer tissue [129]. Synthetic TLR9-activating compounds (e.g. PF-3512676, CpG-ODN) have been clinically tested in combination with chemotherapy in lung cancer patients, unfortunately no clinical benefit was found [130, 131]. However, since preclinical studies have shown that the use of the TLR9 agonist CpG-ODN as an adjuvants in tumor vaccines reduces the number of regulatory T-cells and increases the number of effector T-cells, TLRs remain a potential target in the field of cancer immunotherapy [132].
In addition to compounds that nonspecifically enhance inflammation, the administration of cytokines has been amongst the earliest approaches in cancer immunotherapy. Interferons (IFN) have been one of the major cytokine families of interest given their direct antiproliferative and immunopotentiating effects. In the 1980s, the first clinical trials were conducted in which lung cancer patients were treated with different types of IFN (recombinant alpha and beta) [133, 134]. Since then, the potential of IFN therapy in lung cancer patients has been researched extensively in a number of clinical trials, however no clinical benefits were found [135–138]. Other proinflammatory cytokines of which their potential as a therapeutic target in lung cancer patients has been investigated are IL-2 and TNF-α. In general, treatment with the combination of IL-2 and TNF-α induced relatively grave toxicities and no survival benefits [139]. Currently, the direct administration of proinflammatory cytokines in order to enhance the anti-tumor immune response has been mostly abandoned in lung cancer patients with the exception of the use of colony-stimulating factors with the purpose of the treatment of chemotherapy-induced neutropenia [140, 141].
The group of biological response modifiers consists of a subgroup of multi-modal effectors. The majority of the multi-modal effectors aim to enhance the anti-tumor immune response via the modulation of specific anti-inflammatory immune cells, e.g. Tregs, MDSCs and tumor-associated macrophages. Because of their capability to facilitate an optimal pro-inflammatory immune response, these multi-modal effectors have great potential in combination with more specific immunotherapeutic approaches, e.g. vaccines and cellular immunotherapy. A multi-modal effector which received a lot of attention recently is the recombinant human lactoferrin: talactoferrin alfa. Talactoferrin alfa is an immunostimulatory protein that stimulates dendritic cell maturation in the gut [142]. After promising preclinical and clinical studies in lung cancer patients, a phase 3 study showed no survival benefit of talactoferrin alfa monotherapy in patients with advanced lung cancer [143, 144]. However, the potential of this compound in combination with other immunotherapeutic strategies is topic of further research.
Monoclonal Antibodies
Monoclonal antibodies bind specifically to one epitope and their application as potential immunotherapeutic agents has received a lot of attention recently. The use of monoclonal antibodies directed against tumor growth related antigens on the tumor cell like epidermal growth factor receptor (EGFR) and human epidermal growth factor receptor 2 (HER2) has been well established in lung cancer patients [145, 146]. In addition to the direct effect of the inhibition of growth factors and/or their receptors, antibodies bound to the tumor cell surface can induce antibody-dependent cell-mediated cytotoxicity (ADCC) [147]. Mesothelin is another tumor-specific antigen which is an attractive target for treatment with monoclonal antibodies because of its expression on several epithelial tumors including mesothelioma and lung cancer. Clinical studies with monoclonal antibodies against mesothelin are currently ongoing in lung cancer and mesothelioma patients [148, 149].
In addition to monoclonal antibodies directed against antigens specifically expressed by tumor cells, antibodies that are directed against tumor products have been clinically implemented. In lung cancer patients, the monoclonal antibody bevacizumab which is directed against VEGF has been extensively investigated in clinical trials. In a recent meta-analysis, Cui et al. showed that bevacizumab accompanied by chemotherapy improves clinical outcomes compared to other targeted therapies in chemotherapy-naïve lung cancer patients [150]. Bevacizumab has been developed in order to target blood vessel growth of tumors, however recent evidence shows that bevacizumab also has an immunomodulating effect and enhances circulating CD8 T cells in treated cancer patients [151]. This twofold effect makes bevacizumab an interesting compound to study in combination with other immunotherapies.
The blockade of immune checkpoints using monoclonal antibodies can be considered one of the major breakthroughs in cancer research of the past years. In order to control the immune response and to mitigate collateral tissue damage the immune system is harnessed with a negative feedback system. T-cells have the capacity to upregulate co-inhibitory receptors in order to inhibit the immune response and mediate immune tolerance. Multiple immune-inhibitory pathways (checkpoints) and their accompanying inhibitory co-receptors have been identified. In chronic infection and in cancer, expression of these inhibitory co-receptors is enhanced and associated with an anergic state in T cells [152]. Antibodies that bind to these co-receptors can block inhibitory signals and therefore augment T cell activation and proliferation.
The development of antibodies which bind to co-inhibitory molecules activated during T cell activation has led to the possibility to prevent T cell inhibitory mechanisms and therefore enhance the anti-tumor immune response [153]. The first monoclonal antibody against a co-inhibitory molecule that showed clinical efficacy in cancer patients was anti-CTLA-4 (ipilimumab) [3]. Currently, ipilimumab is approved for the treatment of metastatic melanoma [154]. In patients with advanced lung cancer, treatment with ipilimumab and chemotherapy resulted in a modest survival benefit compared to treatment with chemotherapy alone [155]. Another monoclonal antibody directed against CTLA-4, tremelimumab, has shown encouraging clinical activity in patients with chemotherapy-resistant advanced mesothelioma [156]. In addition to CTLA-4, expression of other co-inhibitory receptors like programmed death protein (PD1) and its ligand PD-L1 have been shown to play a role in lung cancer [157]. Both treatment with anti-PD1 (nivolumab) and anti-PD-L1 resulted in objective responses in patients with advanced lung cancer [158, 159]. Although these results are without any doubt very promising, the implementation of these immune checkpoint inhibitors is hampered by serious immune-related toxicities (e.g. colitis, hepatitis) and low response rates. Therefore, the development of robust, predictive biomarkers is pivotal for the clinical implementation of monoclonal antibodies against co-inhibitory receptors [160]. In addition, phase 3 studies will have to determine whether these immune checkpoint inhibitors will earn their place in the standard treatment of lung cancer.
Tumor Vaccines
The research regarding cancer vaccines has made great progress since the discovery of human tumor antigens which can be recognized by T cell receptors [161]. Tumor vaccines are designed to deliver tumor antigens to antigen-presenting cells, which can subsequently induce a tumor specific immune response by the adaptive immune system. These vaccines can consist of various types of antigen sources. An antigen candidate needs to meet certain criteria in order to potentially be able to elicit a specific anti-tumor immune response. Tumor specificity, frequency and homogeneous expression in tumor cells, role as an oncogene and intrinsic immunogenicity are essential features of antigens which determine the success [5]. In lung cancer and mesothelioma, a broad spectrum of approaches using various antigen sources have been undertaken to develop cancer vaccines. These are here divided into (1) proteins and peptides, (2) liposomal complexes, (3) recombinant viruses and bacterial vectors, and (4) cell-based vaccines.
Proteins and Peptides
Melanoma-associated antigen A3 (MAGE A3) is an antigen which is specifically expressed by several human tumors, including non-small cell lung cancer (NSCLC). Activation of the MAGE genes is known to take place in early carcinogenesis of the lung, however the physiological function of MAGE gene products is unknown [162, 163]. MAGE A3 is detected in approximately 35–50 % of NSCLC and its expression has been shown to be inversely correlated with survival [164, 165]. Vaccines composed of recombinant MAGE A3 (and adjuvants) have shown promising results in lung cancer patients in phase 2 studies and have progressed to a phase 3 trial which is currently being conducted [166]. Epidermal growth factor (EGF) is another eligible candidate to be applied in lung cancer vaccines because of its broad expression in 85 % of NSCLCs. CimaVax EGF is a vaccine composed of human recombinant EGF conjugated to a carrier protein and has proven survival benefits in a phase 2 study with advanced-stage lung cancer patients [167, 168]. In addition to large proteins like MAGE and EGF, smaller peptides can also be used in tumor vaccines. The WT1 (Wilms’ tumor suppressor gene 1) peptide vaccine is composed of four WT1 analogue peptides. WT1 can be expressed in both lung cancer and mesothelioma and vaccination with this peptide has been shown to induce T-cell mediated immune responses in lung cancer and mesothelioma patients [169]. The enzyme telomerase is expressed in most human cancers, including NSCLC, and is therefore considered an attractive cancer vaccine target [170, 171]. Peptide GV1001 consists of 16 amino acids derived from the active site of human telomerase reverse transcriptase [172]. A phase 2 trial with GV1001 showed low toxicity, immune activation and promising clinical responses in NSCLC patients [173].
Liposomal Complexes
Liposomes are known to be potent vaccine delivery systems [174]. The best known cancer vaccine which makes use of this delivery technique is L-BLP25 or Stimuvax. This liposomal vaccine aims to generate an immune response against mucin 1 (MUC1), a cell surface glycosylated phosphoprotein that is frequently overexpressed by epithelial tumors including NSCLC [175, 176]. The L-BLP25 contains the BLP25 lipopeptide and a liposomal delivery system, which facilitates uptake by antigen-presenting cells [176]. A phase 2 trial with advanced stage NSCLC patients showed survival benefits and paved the way for a large phase 3 trial (the START trial) [177]. Unfortunately, Stimuvax failed to increase overall survival in this trial, however, subgroup analyses could still reveal beneficial effects for certain lung cancer patient groups and/or treatment schemes [178].
Recombinant Viruses and Bacterial Vectors
Viruses can be genetically modified in order to express certain antigens and/or co-stimulatory cytokines and are therefore useful as ‘viral vaccines’ in cancer immunotherapy. The earlier described MUC1 protein can also be targeted by the TG4010 viral vaccine. This vaccine consists of attenuated vaccinia virus Ankara which is genetically modified to express MUC1 and IL-2 as adjuvants [179]. In a phase 2 study it was shown that TG4010 enhances the effect of chemotherapy in advanced NSCLC patients, a phase 3 trial is currently being conducted [180].
In addition to viruses, bacteria can also be used as a vaccination vehicle. CRS-207 consists of a live-attenuated Listeria monocytogenes vector encoding human mesothelin [148]. The earlier described mesothelin is an attractive vaccine component because it has been shown to be able to induce a strong CD8 T cell response [181]. CRS-207 has proven to be safe and resulted in immune activation in a phase 1 study amongst patients with mesothelin-positive tumors (lung cancer and mesothelioma included) [182]. Future studies will have to reveal the clinical potential of this mesothelin encoding bacterial vector.
Cell-Based Vaccines
There is a variety of cell-based vaccines under development for the treatment of lung cancer. Cell-based vaccines can be autologous or allogeneic and transfected or not with immunostimulatory compounds. Autologous tumor cell vaccines are ideal antigen sources because they are capable of inducing an immune response to a large variety of antigens expressed by the patient’s tumor. However their practical implementation is complex and challenging for large scale development [183]. An example of an autologous tumor cell vaccine developed for lung cancer is GVAX. This autologous lung cancer vaccine consists of patient-specific irradiated lung cancer cells genetically modified to secrete granulocyte-macrophage colony stimulating factor (GM-CSF) to enhance the immune response. Although a phase 1 study with GVAX showed signs of clinical benefits in lung cancer patients, this study was not followed-up by a phase 2 or phase 3 study [184, 185]. An allogeneic tumor cell vaccine that did reach phase 3 clinical trials is belagenpumatucel-L (Lucanix). Lucanix consists of four irradiated NSCLC cell lines modified with TGF-β2 antisense plasmid. TGF-β is known to be associated with the immune escape of tumors and increased levels of TGF-β are associated with a worse prognosis in NSCLC patients [186, 187]. The addition of the TGF-β2 antisense plasmid aims to stimulate the vaccine-induced immune response by inhibition of the production of TGF-β by the tumor. It is possible to use a combination of tumor cell lines as vaccine cocktail because NSCLC tumor cell lines are described to share immunogenic epitopes with primary tumors [163]. A phase 2 study showed clinical response rates of 15 % amongst advanced stage NSCLC patients [188]. Unfortunately, in a phase 3 study belagenpumatucel-L did not meet its predefined endpoint in the entire patient population. However, in specific subgroups of patients marked improvements in survival were achieved resulting in a current continued development of belagenpumatucel-L for specific indications.
Cellular Immunotherapy
Cellular immunotherapy includes the adoptive transfer of autologous or allogeneic activated immune cells. Initially, adoptive immunotherapy was used for relapses after allogeneic bone marrow transplantation in leukemia patients [189]. Recent advances have facilitated the application and clinical success of this method in various solid tumors [190]. The most prominent success story regarding cellular immunotherapy is sipuleucel-T, a vaccine for prostate cancer that consists of autologous peripheral blood mononuclear cells (PBMCs) including antigen-presenting cells that have been activated ex vivo with a recombinant fusion protein (PA2024, a prostate antigen that is fused to GM-CSF) [2]. After it was demonstrated in a phase 3 clinical trial that sipuleucel-T prolongs survival in metastatic castration-resistant prostate cancer patients, FDA approval followed in 2010. The general goal of adoptive cellular immunotherapy is to induce a tumor-specific immune response via the infusion of e.g. tumor-antigen loaded DCs or specifically activated T cells. In lung cancer, cellular immunotherapeutic approaches using various cell types have been evaluated [191].
Dendritic Cells
As described earlier, DCs are the professional antigen-presenting cells of the immune system and they have emerged as the most powerful initiators of immune responses. Because of their capacity to engulf tumor antigens and activate T-cells in an antigen-specific manner, the use of DCs as immunotherapeutic agents is very promising. In DC-based immunotherapeutic approaches, DCs are generated ex vivo from monocytes and after arming with tumor-associated antigens, reinjected into the patient with the intention to restore proper presentation of tumor-associated antigens and T-cell activation. This concept has been researched in NSCLC and has shown promising results regarding the elicited immune response, safety and tolerability, despite the small sample sizes of the trials [192–194]. In mesothelioma, treatment with autologous tumor-lysate pulsed DCs was shown to be safe and elicited an anti-tumor immune response in a phase 1 clinical trial [195].
T Cells
Different sources and activation procedures can be used in specifically harnessing the T cell response and have been clinically evaluated in lung cancer. Lymphokine-activated killer (LAK) cells are autologous IL-2 stimulated lymphocytes and their application has shown clinical responses in several studies with lung cancer patients [196, 197]. Cytokine-induced killer (CIK) cells are generated in vitro by stimulation of peripheral blood lymphocytes with anti-CD3 antibodies, IL-2, IL-1α, and IFN-γ [198]. CIK cells have proven their clinical potential in multiple solid tumors including lung cancer [199] and these results warrant future clinical trials [200]. In addition, in a recent study autologous purified T lymphocytes activated with anti-CD3 antibodies and IL-2 demonstrated an extended survival in patients with advanced NSCLC, however this effect was seen in a historical cohort study and therefore these data will have to be confirmed in a prospective randomized trial [201]. Because expanded gamma delta T cells showed strong cytotoxicity to lung cancer cell lines in vitro, the exploitation of this cell type has been tested clinically. In a phase 1 study, autologous expanded gamma delta T cells unfortunately showed limited clinical responses in NSCLC patients [202, 203].
Natural Killer Cells
Adoptive transfer of allogeneic, in vitro activated and expanded NK cells from haploidentical donors was proven potentially clinically effective in NSCLC [204]. NKT cells are currently exploited for cancer treatment by harnessing these cells with CD1d agonist ligands [205], or by adoptive transfer of NKT cells activated in vitro [206].
Response Evaluation
Immunotherapy represents a new class of agents in the treatment of lung cancer. As demonstrated for sipuleucel-T in prostate cancer and ipilimumab in melanoma, treatment responses and improvement of overall survival can be seen in lung cancer patients. However, often the agents did not change initial disease progression. Even a transient worsening of disease manifested either by progression of known lesions or the appearance of new lesions can be seen, before disease stabilization or tumor regression. The commonly accepted treatment paradigm, however, suggests that treatments should initially decrease tumor volume, which can be measured using CT scan. Also, progression-free survival is increasingly used as an alternative end-point of studies. This seems to be unfortunate for immunotherapy, which may initiate an immune response that ultimately slows the tumor growth rate, resulting in longer survival, but not a decrease in tumor volume on CT scan or an increased progression-free survival [257]. Immune-related response criteria which adapt the standard response criteria and include the potential for delayed clinical response and initial increase of tumor mass after immunotherapy are currently being developed [207]. Therefore, clinicians at this moment may need to reconsider how to measure success of their immunotherapeutic approach [208].
The Future of Cancer Immunotherapy
For a long time, stimulating the patient’s immune system to attack tumors has been viewed as a rather meaningless intervention, an assumption that has radically changed since the recent clinical successes of cancer immunotherapy. However, despite the promising results achieved in some patients, the overall response rates are low. It has been shown that the immune contexture (i.e., the type, density and location of tumor-infiltrating immune cells) can predict the clinical outcome of patients affected by multiple types of cancer, but with consistent intra-patient variations [209]. It is therefore critical to identify the unique immunological profile of individual patients as a means to identify the best-suited immunotherapeutic approach for each patient [153]. This personalized way could greatly improve the efficacy of current immunotherapies. In addition to an individualized treatment plan, the future of immunotherapy in lung cancer patients includes multimodality treatment. Surgery, irradiation or chemotherapy vigorously reduce the tumor mass and the induced tumor cell death results in tumor antigen exposure. These therapeutic effects enhance the efficacy of immunotherapy and warrant combinatorial treatment approaches. In addition, multiple chemotherapeutical agents have been described to modulate the tumor microenvironment and therefore enhance immunotherapy [210, 211]. Gemcitabine and vinorelbine are known to be able to reduce circulating MDSCs [212, 213], increase the ratio of M1 to M2 macrophages [214] and stimulate APCs [215]. Therefore, the combination of immunotherapy with conventional treatments can elicit a synergistic treatment response and takes advantage of the full potential of cancer immunotherapy.
References
1.
Couzin-Frankel J (2013) Breakthrough of the year 2013. Cancer immunotherapy. Science 342(6165):1432–1433PubMed
2.
Kantoff PW et al (2010) Sipuleucel-T immunotherapy for castration-resistant prostate cancer. N Engl J Med 363(5):411–422PubMed
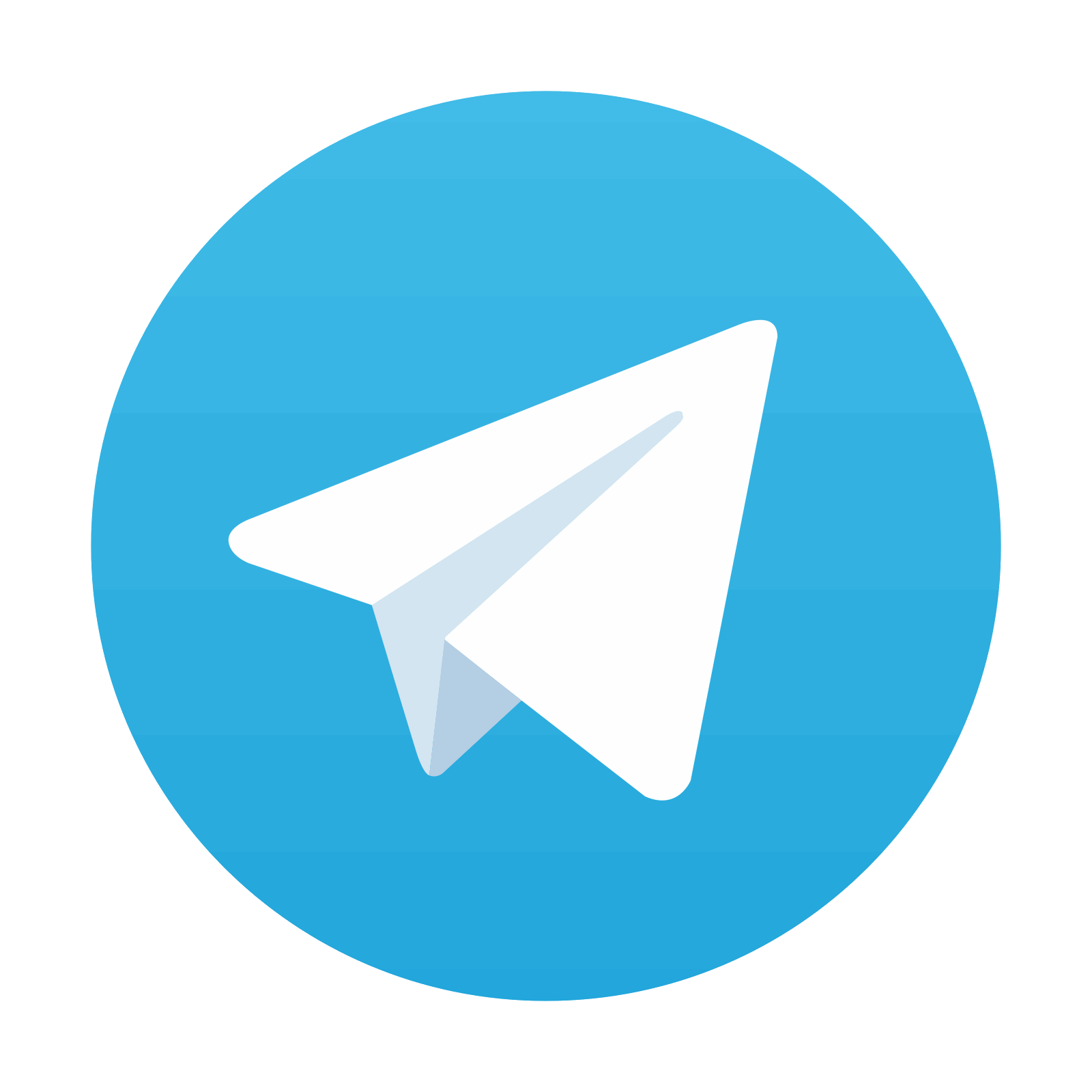
Stay updated, free articles. Join our Telegram channel

Full access? Get Clinical Tree
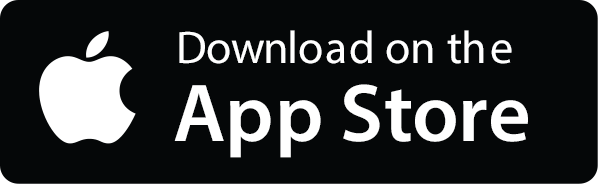
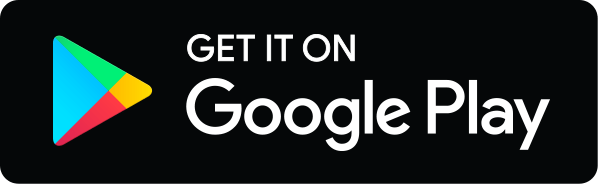