Fig. 9.1
Risk assessment algorithm for a patient with newly diagnosed localized renal cell carcinoma. Assessing risk occurs throughout the continuum of patient care. Risk assessment during initial evaluation requires quantitating treatment trade-offs in an objective manner. Pretreatment risk management requires education and communication about specific risks associated with a chosen therapy. Treatment risk management includes abatement of those risks during therapy using objective, metric-based data. Finally, posttreatment risk management involves mitigating future progression, complications, and anxiety using objective, data-driven strategies
The risk-benefit equation must be seriously considered when one realizes that the proportion of the US population who will be aged 65 years or older in 2030 is estimated to be 20 % [15]. Some authors have estimated that 60 % of cancers and 80 % of all cancer-related deaths in the United States occur in patients over the age of 65 [16]. Similarly, as patients age, they develop medical comorbidities that may be severe enough to impact their ability to receive or tolerate optimal cancer therapies [17]. Thus, the severity of a patient’s comorbidity needs to be contextualized against the biologic behavior of the cancer.
Today, such decision making regarding risks and trade-offs in the management of localized RCC remain largely qualitative; however, clinically useful methods to quantitate risk are beginning to emerge. For instance, several comorbidity indices and scores have been proposed [18], and new approaches are steadily being introduced [19]. The Charlson Comorbidity Index (CCI) [20] is one of the best studied and most commonly employed methods for risk stratification [18]. The CCI incorporates 19 disease entities that include such ailments as cardiovascular, pulmonary, hepatic, and renal dysfunction. The degree to which each condition contributes to the index depends on that condition’s calculated impact on mortality. Today, even in a busy clinical setting, the CCI can be rapidly calculated using web-based tools (e.g., http://www.medal.org/visitor/www/qhc/index.html).
Another potentially useful objective measure of a patient’s risk with surgery is the preoperative measurement of a patient’s “frailty.” Originally developed by geriatricians, frailty is a relatively new concept that encompasses not only a patient’s chronologic age but also a patient’s ability to withstand physiologic stressors. Fried et al. initially introduced this concept and described frailty “as a biologic syndrome of decreased reserve and resistance to stressors, resulting from cumulative declines across multiple physiologic systems, and causing vulnerability to adverse outcomes” [21]. In their initial study, Fried and colleagues operationalized the measurement of frailty focusing on the following domains: shrinking (weight loss or sarcopenia), weakness, slowness, poor endurance/exhaustion, and low activity. More recent studies have shown that the preoperative identification of intermediately frail or frail patients can predict for postoperative adverse events [22]. Ideally, in the future, an objective frailty score could be combined with other measures to develop a refined scale to precisely predict a patient’s risk of adverse events with surgical intervention.
Also, in order to make informed and calculated decisions regarding the management of small localized renal masses, the physician must be able to estimate a patient’s probability of dying from localized RCC and compare this to the patient’s chances of dying from competing causes. Indeed, such predictive models have been developed for non-genitourinary solid malignancies [23, 24]. Similar tools are starting to emerge for localized RCC [25–27]. Our group recently developed a nomogram from a multivariable model based on over 30,000 patients from the Surveillance, Epidemiology, and End Results (SEER) program database who had resection of localized RCC [27]. The nomogram affords the clinician and the patient an opportunity to quantitate three competing 5-year mortality outcomes: (1) death from RCC, (2) death from other (non-RCC) cancers, and (3) non-cancer death. For instance, using the nomogram, a 75-year-old white male with a 4 cm tumor would have a 5-year mortality of 5 % from RCC versus 4.5 % from other cancers and 14 % from noncancerous causes. Even more recently, this nomogram has been adjusted to include a patient’s comorbidities, using the CCI [28]. In light of these competing risks and the known short-term indolent behavior of many localized RCC, active surveillance (AS) has emerged as a viable treatment strategy for patients with renal tumors. In this situation, RMB may prove beneficial in objectively evaluating the histology and Fuhrman grade in an attempt to more accurately predict the behavior of the mass.
When considering AS as a management strategy for a newly diagnosed renal mass, it is helpful to consider absolute, relative, and elective indications. Absolute indications include patients in whom surgery poses an immediate and unacceptable risk of mortality. Relative indications for observation include concomitant diseases, such as a second malignancy and/or significant but not overriding medical comorbidities. Lastly, some patients may simply wish to undergo a period of AS despite being low-risk surgical candidates. This constitutes an elective indication for AS and requires the treating physician to inform the patient of the available data on renal tumor growth kinetics, with limitations and the uncertain long-term risk of progression. No matter what the indication for AS of a renal mass, it must be understood that the patient and physician are both taking a calculated risk due to the heterogeneous and occasional unpredictable behavior of RCC.
In summary, quantification of a patient’s perioperative risk as well as competing risks of death must be thoughtfully integrated into clinical decision making. Current ubiquitous qualitative approaches must be replaced by quantitative strategies. Given the known yearly growth rates of SRMs [14] and the low likelihood of developing metastatic RCC in masses less than 4 cm when followed for 24–30 months [14, 29], AS is a reasonable treatment strategy in the elderly or patients with severe medical comorbidities.
9.3 Objectification of Renal Tumor Anatomy
Despite, or because of, the myriad treatment options available to the patient and treating urologist, clinical decision making for localized RCC is overly subjective. It is based on numerous often qualitative factors, including competing health risks (real or perceived), the interpreted tumor anatomy, physician experience and comfort, and patient preference/perceptions of the ease/efficacy of various treatment modalities.
We introduced the RENAL nephrometry scoring system as a means to objectify the salient anatomic features of a renal mass on cross-sectional imaging. This system may be utilized to compare outcomes and develop metrics for treatment decision making [30] (Fig. 9.2). In the absence of a common nomenclature to describe the anatomical attributes of a renal tumor, treatment decision making is subject to an unmeasured physician’s biases and individual experience. A tumor’s nephrometry score is a structured and quantifiable method to describe the tumor’s relevant anatomical features as they relate to the complexity of a tumor, its difficulty of resection, and potential treatment risks.
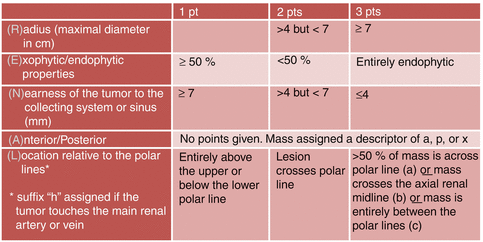
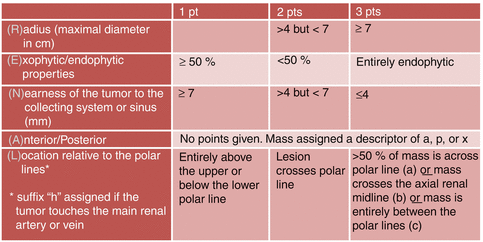
Fig. 9.2
RENAL nephrometry scoring system
The scoring system is based on the five most reproducible features that characterize the anatomy of a solid renal mass: (R)adius (scores tumor size as maximal diameter), (E)xophytic/endophytic properties of the tumor, (N)earness of the deepest portion of the tumor to the collecting system or renal sinus, (A)nterior (a)/posterior (p) descriptor, and (L)ocation relative to the polar line. All components except for the (A) descriptor are scored on a 1-, 2-, or 3-point scale. The (A) describes the principal mass location to the coronal plane of the kidney. The suffix “x” is assigned to the tumor if an anterior or posterior designation is not possible. An additional suffix “h” is used to designate a hilar location of the tumor (abutting the main renal artery or vein).
The RENAL nephrometry scoring system represents the first-introduced method to attempt to standardize the reporting of the salient anatomy of an enhancing renal mass. Subsequently, the PADUA score was introduced as another objective method to describe the anatomical features of a renal mass [31]. The PADUA score is remarkably similar to nephrometry with the exception of “the definition of the sinus lines and the evaluation of the anatomical relationship between the tumor and urinary collecting system or renal sinus” [31]. Lastly, the C-Index Method was introduced to characterize a tumor’s centrality. This method requires a complex geometric calculation using cross-sectional imaging to determine the distance from the tumor center to the center of the kidney [32]. We believe that the RENAL nephrometry scoring system is unique in that it is an accessible system that can be learned rapidly and applied that reliably describes the most salient renal mass features.
By creating a reproducible system based on the renal mass anatomy, we have objectified the descriptions of renal masses that previously were simply referred to in terms such as “simple” or “difficult,” thereby creating a platform to ascertain the optimal surgical approach. For example, in a recent evaluation of our institutional database, 94 % of low-complexity (nephrometry score = 4–6) masses were treated with a PN, most using an MIS technique. Nephrometry has several additional uses beyond aiding in surgical treatment decision making. Recent investigators have adopted nephrometry to examine its ability to predict for functional, perioperative, and pathologic outcomes. Cha et al. showed that patients with higher “nephrometric variables,” (R) and (E), were more likely to experience postoperative renal impairment after a MIS-PN [33]. Two other groups have shown that higher nephrometry scores predict for increased blood loss and longer ischemia time when undergoing either MIS-PN or open PN [34, 35]. Additionally, a prospective study at our institution validated that higher nephrometry scores can be used to predict prolonged warm ischemia time [36]. Finally, despite prior work reporting no significant biological differences between centrally and peripherally located tumors [37], nephrometry was recently evaluated to determine its ability to preoperatively predict the histology and grade of enhancing renal masses. In this work, the authors found a high correlation between nephrometry score and tumor grade (p < 0.0001) and histology (p < 0.0001) [38]. Specifically, papillary RCCs had the lowest total nephrometry score, while clear cell RCCs had higher nephrometry scores. Furthermore, benign lesions tended to be smaller, more endophytic, and non-hilar [38].
Nephrometry creates a platform to standardize salient renal mass anatomy. In doing so, objective treatment decision making can be performed when the urologist considers the functional, perioperative, and preoperative pathologic information that one can derive from the RENAL nephrometry scoring system.
9.4 Assessment and Implications of Chronic Kidney Disease (CKD)
The systematic review by the RCC guidelines committee of the AUA highlights the priority of goals when managing localized RCC: (1) optimize cancer treatment, (2) preserve renal function, and if the first two goals are met, (3) utilize a minimally invasive technique while minimizing the risk of adverse postoperative events [5]. Published series have established the oncologic efficacy of nephron-sparing surgery (NSS) for pT1a and pT1b renal tumors [5, 39–42]. Despite these findings and other data indicating that PN confers a non-oncological survival advantage, nationally the use of PN for tumors less than 4 cm continues to be less than 30 % [43]. As more incidental renal masses continue to be detected and the adverse relationship between long-term CKD and morbidity/mortality is uncovered, the importance of renal functional preservation continues to be paramount.
Traditionally, serum creatinine (sCr) has been used to measure the presence or absence of renal dysfunction; however, this can be a misleading value, since sCr can be affected by age, gender, muscle mass, and diet. Furthermore, since creatinine is both secreted and reabsorbed by renal tubules, certain medications, such as cimetidine and sulfonamides, can alter sCr by inhibiting its tubular secretion. Recent data suggest that serum creatinine measurements are a poor tool to estimate the degree of renal impairment [9, 10]. In fact, in a recent cross-sectional analysis comparing the National Health and Nutrition Examination Surveys (NHANES) between 1988–1994 and 1999–2004 consisting of approximately 29,000 patients, 25 % of patients with a “normal” sCr had chronic kidney disease (CKD) stage 3 or greater, as defined by the National Kidney Foundation [44]. With the recent data underscoring the prevalence of CKD in the general population, attention has focused on estimating the glomerular filtration rate (GFR) as a measure of a patient’s renal function. More precise measures of GFR have recently been adopted including the MDRD and CKD-EPI. Therefore, the socioeconomic and health implications of significant national underutilization of NSS are likely clinically underestimated.
The risk of postoperative chronic kidney disease after RN when compared to PN has been well studied. McKiernan et al. showed that the risk of having a postoperative baseline sCr greater than 2.0 mg/dL was significantly greater following RN when compared to a PN [45]. A more precise quantification of CKD after nephrectomy was undertaken by Huang et al. Using the MDRD equation to estimate GFR, the authors found in a multivariable analysis that RN was an independent risk factor for patients developing an eGFR of less than 60 ml/min and less than 45 ml/min [46]. The incidence of baseline renal dysfunction (eGFR <60) in their study was 26 %.
The relationship between CKD and the risks of death, cardiovascular events, and hospitalization rates is clinically relevant but has previously not received much attention because it is often an event that occurs well past the initial surgical loss of nephrons. With each 15 ml/min diminution of eGFR below 60 ml/min, the risk of death, cardiovascular events, and hospitalization increases [47]. For example, the adjusted hazard ratio for death in a patient with an eGFR of 45–59 ml/min is 1.2 while it is 5.9 for an eGFR less than 15 ml/min [47]. Furthermore, the interaction between age and CKD and their effects on survival requires the urologist to diligently assess an elderly patient’s renal function preoperatively. In one study, more than 50 % of patients older than 75 years died within 2 years after starting dialysis [48]. The median survival time for this aged population on dialysis was 22 months.
The prevalence of CKD stage III or higher based on NHANES 1999–2004 data has increased to over 8 % [44]. It is unclear if a population enriched for patients with radiographically concerning RCC reflects this trend or has a potentially higher risk of CKD. In a recent review of our institutional kidney cancer database, we showed that although 88 % of all patients presenting for surgery with a solid renal mass at our institution had a “normal” sCr (≤1.4 mg/dl), 12.5 % of these patients had CKD stage III when estimating GFR [11]. Moreover, 23 % of patients 70 years old or greater with a seemingly normal sCr had CKD stage III. These findings support reports by other authors who have argued for more precise measurement of a patient’s renal function, either by the MDRD equation or the newly developed Chronic Kidney Disease-Epidemiology Study equation, to better assess a patient’s renal function [9, 10]. Finally, the national average of NSS, ranging from 27 % [49] to 40 % [43] for pT1a tumors, is concerning in light of our findings showing an underestimation of chronic kidney disease by routine serum creatinine monitoring. This study highlights the fact that both eGFR and CKD stage must be routinely calculated and clinical decisions based on these variables not sCr, especially in the elderly.
9.5 Treatment of Early-Stage RCC: Excise
9.5.1 Comparison of Oncologic Outcomes Between Radical Nephrectomy and Partial Nephrectomy
The mainstay of treatment for RCC is surgical therapy due to its resistance to chemotherapy and radiation therapy. Recent advances in the development of targeted therapies for advanced RCC have resulted in longer survival for patients with metastatic RCC; however, treatment for localized RCC remains surgical extirpation. The management of RCC has been governed by Robson’s initial description in 1963 of a radical nephrectomy (RN) for the treatment of all renal tumors [50]. Utilizing a flank, subcostal, or midline incision, Robson’s description of an RN included the removal of the entire kidney, perirenal fat, surrounding Gerota’s fascia, overlying peritoneum, and adrenal gland [50]. This approach resulted in excellent oncologic outcomes [51]. In cases where surgical extirpation of the kidney would render a patient functionally or anatomically anaphoric, an “essential” partial nephrectomy (PN) was performed in these select patients to avoid the need for renal replacement therapy. As data on oncologic outcomes of patients who underwent an “essential” PN emerged, the use of PN for elective indications was gaining acceptance. During the past decade, the paradigm has shifted toward treating localized RCC with nephron-sparing surgery (NSS) as oncologic outcomes have proven to be equivalent to traditional RN (Table 9.1). In fact, in the recent AUA guidelines, which reviewed all the existing literature for oncologic outcomes for RN and PN, recurrence-free survival rates were equal at 98.0–99.2 %, respectively [5].
Table 9.1
Oncologic outcomes in patients treated with radical nephrectomy compared to nephron-sparing surgery
Series | Year | Tumor stage | No. patients/average tumor size (cm) | % 5-year CSS | Controlled for | ||
---|---|---|---|---|---|---|---|
RN | NSS | RN | NSS | ||||
Robson | 1969 | Stage 1 (confined to the kidney) | 33 | n/a | 66 | n/a | |
Butler | 1995 | T1a | 42 (2.7 ± 0.8) | 46 (2.5 ± 0.8) | 97 | 100 | Age, gender, tumor size, location and stage, renal function, comorbidities |
D’Armiento | 1997 | T1a | 21 (3.21) | 19 (3.34) | 96 | 96 | Age, gender, date of surgery, tumor size, grade |
Indudhara | 1997 | T1a, T1b | 71 | 35 | 94 | 91 | Age, gender, date of surgery, tumor size, stage |
Belldegrun | 1999 | T1a, T1b | 125 (6.2) | 108 (3.6) | 91.2 | 98 | Age, gender, date of surgery, tumor stage, follow-up |
Barbalias | 1999 | T1a, T1b | 48 (3.8) | 41 (3.5) | 98.4 | 97.5 | Age, tumor size, stage, location |
Lee | 2000 | T1a | 183 (3.0) | 79 (2.5) | 95 | 95 | Gender, tumor histology, stage, age, gender, date of surgery, tumor stage, grade, location |
Thompson | 2009 | T1b | 704 (5.5) | 239 (5.0) | 91 | 96 | Age, gender, local or systemic symptoms at presentation, Charlson comorbidity index, diabetes, solitary kidney, preoperative serum Cr, eGFR, CKD, 2002 primary tumor classification |
Crepel | 2010 | T1a | 5,658 (2.8) | 1,622 (2.5) | 97.5 | 98.2 | OCM (other cause mortality), age, year of surgery, tumor size, and Fuhrman grade |
In addition to oncologic equivalency, nephron preservation also results in improved renal functional outcomes after surgery [45, 46]. Furthermore, several recent studies have shown a defined benefit with PN compared to RN in terms of overall survival and reduced rates of cardiovascular events and non-cancer-related deaths [52–54]. Weight et al. published the Cleveland Clinic’s follow-up data comparing survival outcomes in patients undergoing RN or a PN for a cT1b renal mass. In this cohort of 1,004 patients, postoperative eGFR was an independent predictor of overall survival and cardiac-specific survival on multivariate analysis. Patients treated with PN had a statistically significant improved 5-year OS compared to patients treated with RN (85 % vs. 78 % (p = 0.01)) [52]. Interestingly, of the 175 deaths in this cohort, 48 were due to cardiovascular events and 19 were related to renal failure. Similar conclusions were reached by Thompson et al. and Huang et al. when examining the Mayo Clinic nephrectomy registry as well as the SEER cancer database. Their data demonstrated that in patients younger than 65 years old treated for a pT1a renal mass, RN was significantly associated with death from any cause (RR 2.16, p = 0.02) [53]. Also, a query of the SEER cancer registry showed a statistically significant increase in the risk of cardiovascular events (p < 0.05) and all-cause mortality (HR 1.46, p < 0.001) for patients treated with RN for a pT1a renal mass [54]. Furthermore, in a graded fashion, renal dysfunction has been shown to be associated with significantly increased cardiovascular risks, hospitalizations, and mortality [47]. Finally, when employed in elective situations, health-related quality of life scores were higher in the PN compared to RN group [55] with equivalent lengths of stay and direct hospital costs [56].
Despite oncologic equivalency and improved renal functional outcomes, NSS carries a higher risk of a major urologic complication that must be considered in the risk/benefit equation. In the recent AUA guidelines concerning the management of the clinical T1 renal mass, the complication rate for open PN ranges from 4.5 to 8.7 % based on the results of 15 published studies [5]. Also, the recent EORTC trial comparing PN to RN in tumors less than 5 cm highlights this risk/benefit balance. In this prospective randomized study of 541 patients, PN was associated with a statistically significant increased risk of severe hemorrhage, defined as >1 L, and urinary fistulas (p < 0.001) [57]. Conversely, patients who underwent a PN had a statistically significant lower sCr at follow-up (p < 0.0001). Similarly, other studies have shown that as tumor size or tumor complexity increases, the incidence of technical adverse events increases too. Patard et al. compared morbidity in patients undergoing PN for tumors <4 cm and >4 cm. In this study, there was a statistically significant increase in the rates of blood transfusions (p = 0.001) and urinary fistula (p = 0.01) in patients undergoing PN for tumors >4 cm [58]. Clearly, the risks of chronic kidney disease and their attendant detrimental health effects need to be quantified and weighed against the more immediate and short-term surgical risks.
9.5.2 Partial Nephrectomy for pT2 Renal Masses
Extending the partial nephrectomy experience for pT1b tumors, newer series have demonstrated that PN for T2 renal masses has equivalent oncologic outcomes compared to RN with acceptable perioperative complication rates. Hansen et al. published a study examining the SEER database which compared 245 patients who underwent a PN and 8,602 patients who underwent an RN for a renal mass >7 cm. In their analysis, the cancer-specific mortality (CSM) for an RN was 5.7 % and 17.7 % at 2 and 5 years, respectively [59]. For PN, the CSM was 3.4 and 11.9 % at the same time points which was not statistically significant different [59]. Breau et al. found that RCC-specific survival and OS were similar when their institution matched PN to RN for stage T2 tumors or greater. Local recurrence occurred in 4 (6 %) patients, while distant spread occurred in 15 (22 %) individuals [60].
Similarly, our institution performed an analysis of 49 renal tumors >7 cm that were treated by PN. The 5- and 10-year RCC-specific survival was 94.5 and 70.9 % [61]. In our series, 8.2 % of patients required blood transfusions, and 12.2 % developed urinary fistulas [61]. As previously mentioned, in a recent EORTC trial, 541 patients with cT1 renal masses ≤5 cm were randomized to PN or RN. PN was associated with a statistically significant increased risk of severe hemorrhage (defined as >1 L) and urinary fistula (p < 0.001) [57]. Conversely, patients who underwent a PN had a statistically significant lower serum creatinine at follow-up (p < 0.0001). Our study, among others, has shown that in the appropriate setting, PN for pT2 masses is oncologically sound with acceptable complications rates. Furthermore, as expected, PN is associated with better renal functional preservation. Urologists must weigh the risks and benefits of PN, including improved renal functional outcomes and improved cardiovascular status, with an increased risk of short-term morbidity in the setting of oncologic equivalence.
9.5.3 Comparison of Open and Minimally Invasive Techniques in the Treatment of Localized RCC
With the advent of minimally invasive surgery, laparoscopic techniques have been applied to the kidney. There was an initial reluctance to adopt laparoscopic renal surgery widely because of concerns for tumor seeding of the peritoneum. Also, maculation of specimens raised concerns for inadequate staging. Today, nephrectomy specimens are removed intact, and concerns over tumor seeding have not been substantiated. Indeed, although prospective randomized trials of open versus laparoscopic radical nephrectomy were never completed, long-term retrospective data suggest oncological equivalence between the two approaches [62–66] (Table 9.2). Today, given significantly lower intraoperative blood loss and shorter convalescence, laparoscopic RN is the standard of care for renal surgery that requires total removal of the kidney [62].
Table 9.2
Oncologic comparison between open and laparoscopic partial nephrectomy
5-year disease-free survival (%) | |||||
---|---|---|---|---|---|
Series | No. patients | T1a | T1b | T2 | Positive margins (%) |
Fergany et al. (2000) | 107 | 97.6 | 95 | 100 | – |
Gill et al. (2002) | 200 | 91 vs. 73 | 9 vs. 27 | N/A | 3.0 vs. 1.0 |
Permpongkosol et al. (2005) | 143 | 91.4 vs. 97.2 | 75 vs. 75 | N/A | 2.4 vs. 1.7 |
Gill et al. (2007) | 1,800 | 99.3 vs. 99.2 | N/A | 2.9 vs. 1.3 |
In 1990, the first laparoscopic radical nephrectomy (LRN) was performed by Clayman et al. for a 3 cm oncocytoma [67]. In that case report, each segmental artery was dissected and individually ligated, because the clips available at that time were not large enough to secure the main renal artery. Furthermore, a preoperative angioinfarction of the kidney was performed, and intraoperatively, a ureteral catheter was placed. Since that initial report, the laparoscopic renal surgery rapidly gained traction. Presently, at centers of excellence, the vast majority of nephrectomies are performed via a laparoscopic approach. Furthermore, surgery for large renal tumors and tumors with thrombi extending into the renal vein and even the vena cava is now being performed laparoscopically [68–70].
Coincident with the growth of laparoscopy has been the increased detection of incidental SRMs during the last two decades, as cross-sectional imaging has become a routine diagnostic tool [1]. Thanks to the widespread acceptance of NSS and refinement of laparoscopic instrumentation, a patient can be offered a PN via laparoscopic approaches (with and without robotic assistance) utilizing only three or four small incisions, none measuring greater than 1.2 cm. A large multi-institutional retrospective study comparing laparoscopic partial nephrectomy (LPN) with OPN provided evidence on multivariate analyses that LPN was associated with decreased blood loss and shorter operative times and hospital stays [71]. However, perioperative/postoperative complications, such as prolonged warm ischemia and renal hemorrhage, and re-exploration rates were notably higher in the LPN group, while oncologic control appeared to be equivalent in the two groups. The AUA systematic review published its guidelines on the treatment for stage I renal tumors identifying a nearly 50 % increase in “major complications” in LPN compared to OPN [5]. Despite the increase in major urologic complications, cancer control for appropriately selected patients appears to be preserved [72].
More recently, robotic-assisted laparoscopy has emerged as another tool in the armamentarium for treatment of localized kidney cancer (Fig. 9.3). As urologists have become more familiar with robotic techniques, the usage of robotics has broadened to include NSS. Robotic assistance enables the surgeon to perform more efficient intracorporeal suturing and thus safely resect larger, more anatomically complex lesions. Furthermore, the learning curve for robotically assisted laparoscopic partial nephrectomy (RALPN) may be less steep than LPN, based on equivalent same surgeon results when comparing initial RALPN versus vast LPN experience [73]. Sitting at the console, the robotic user can rotate one’s wrists 180° and pass suture from virtually any angle. Renal reconstruction can be performed in 3-D, and the passing of suture through the kidney is easier than with pure laparoscopic technique due to the wrist motions of the robot.
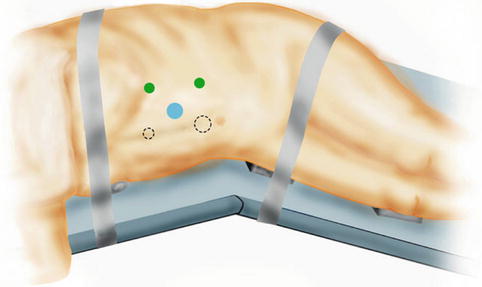
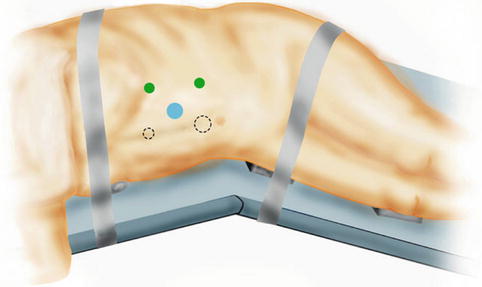
Fig. 9.3
Usual port site arrangement for left transperitoneal robot-assisted partial nephrectomy. Two common arrangements are depicted. Blue circles indicate camera ports. Dashed circles indicate assistant ports. Larger circles represent 12 mm ports, while the smaller circles represent 5 mm ports. Green circles represent 8 mm ports that accommodate the robotic arms
Many small series have been published showing that a RALPN is technically feasible without increasing patient morbidity [74–77] (Table 9.3). These series do not have long enough follow-up to show equivalent oncologic control as the open or laparoscopic approaches; however, currently, there is no suspicion that the technique is inferior [74–76]. The largest recent series concluded that RALPN is an oncologically sound approach with acceptable immediate nephron-sparing outcomes [77].
Table 9.3
Short-term outcomes of published robotic-assisted partial nephrectomy series
Series and institution | RAPN (n) | Tumor size (cm) | Complications by Clavien Grade (II–V) | Positive margins (n) | Urine leaks (n) |
---|---|---|---|---|---|
Gettman et al. Mayo Clinic | 13 | 3.5
![]() Stay updated, free articles. Join our Telegram channel![]() Full access? Get Clinical Tree![]() ![]() ![]() |