INTRODUCTION
SUMMARY
Most external influences upon cells of any organ are mediated by biochemical and molecular mechanisms that are triggered by interactions with membrane, cytoplasmic, or nuclear receptors. Our understanding of the receptors and the intermediate molecules that couple them with cellular pathways that influence the proliferation, activation, differentiation, or survival of hematopoietic cells has expanded significantly. Proteins on the surface of blood cells that transmit vital information from the extracellular environment include single-pass, homodimeric, heterodimeric, and heterotrimeric transmembrane proteins that do, or do not, contain intrinsic kinase activity, but either way signal by inducing the tyrosine phosphorylation of a multitude of cytoplasmic proteins, seven transmembrane domain proteins that signal through G proteins, heterodimeric integrins that recruit large focal adhesions, and large families of heterodimeric proteins that induce serine and threonine phosphorylation. This chapter describes the receptors that influence blood cell production and function, the secondary mediators and the biochemical modifications they undergo to alert the cell to an external influence, the molecular mechanisms that allow for the coordination of multiple signals impacting a cell simultaneously, and the processes upon which they impact.
Acronyms and Abbreviations:
AP2, adaptor protein-2; BCR, B-cell antigen receptor; BMP, bone morphogenic protein; CNTF, ciliary neurotrophic factor; CT-1, cardiotrophin-1; DD, death domain; DR, death receptor; EPO, erythropoietin; EPOR, erythropoietin receptor; ERK, extracellular response kinase; FADD, Fas-associated death domain; FAK, focal adhesion kinase; G-CSF, granulocyte colony-stimulating factor; Gab, Grb binding; GH, growth hormone; GM-CSF, granulocyte-macrophage colony-stimulating factor; GPCR, G-protein-coupled receptor; HCR, hematopoietic cytokine receptor; IAP, inhibitors of apoptosis; IKK, I-κB kinase; IL, interleukin; IRS, insulin receptor substrate; ITAM, immunoreceptor tyrosine-based activation motif; ITIM, immunoreceptor tyrosine-based inhibitory motif; JAK, Janus family kinase; JNK, c-Jun N-terminal kinase; LIF, leukemia inhibitory factor; M-CSF, macrophage colony-stimulating factor; MAPK, mitogen-activated protein kinase; NR, nuclear receptor; OSM, oncostatin M; PI3K phosphoinositol 3′-kinase; PIAS, protein inhibitor of activated STATs; PIP, phosphoinositol phosphate; PKC, protein kinase C; PTP, protein tyrosine phosphatase; RACK, receptor for activated C kinase; RTK, receptor tyrosine kinase; SARA, SMAD anchor for receptor activation; SCID, severe combined immunodeficiency; SH2, Src homology 2; SOCS, suppressors of cytokine signaling; STATs, signal transducers and activators of transcription; SUMO, small ubiquitin-like modifier; TGF, transforming growth factor; TM, transmembrane; TNF, tumor necrosis factor; TPO, thrombopoietin; TRADD, TNF receptor death domain; TRAF, TNF receptor-associated factor; TRAIL, tumor necrosis factor-related apoptosis-inducing ligand.
AN OVERVIEW OF CELL SIGNALING
Blood cells and their marrow-based progenitors are exquisitely responsive to their environment. A wide variety of cues are detected by mature blood cells that impact significantly on their function. For example, leukocytes respond to noxious stimuli by chemokine-induced migration toward inflammatory stimuli, cross endothelial cell barriers and the extracellular matrix by engaging integrins, and then respond to chemotactic gradients to enter inflammatory foci to contact and engulf microorganisms on encountering bacterial products. Likewise, platelets adhere to reactive endothelial surfaces or denuded subendothelial cell matrix by engagement of extracellular adhesive proteins. Adherent platelets can also recruit additional platelets and aggregate with them through interactions with platelet integrins, secrete growth factors that will recruit cells that mediate repair of vascular injury and then contract to strengthen the platelet plug by engagement of numerous granule substances. Even the anucleate erythrocyte responds to mechanical deformation and hypoxemia with adenosine triphosphate (ATP) release. Adrenergic receptors also play important roles in the normal erythrocyte response to parasitic infection or in the pathologic red cell’s interactions with endothelial cell surfaces (e.g., patients with hemoglobinopathies). Each of these events induces an intracellular signal that leads to further cellular reactivity toward the initiating stimulus, or that prepares the cell for subsequent functional events. Like the functional activation of mature blood cells, the generation of blood cells is under tight regulation, mediated by soluble hematopoietic growth factors, cytokines, and components of the marrow microenvironment. Here again, the erythropoietin (EPO) response to anemia is sensed by erythroid progenitor cell surface receptors; their coordinated reaction involves a myriad of signals that impact on the survival, growth, and differentiation of both undifferentiated and lineage-committed cells. Although anemia induces red cell production and inflammation leads to the production and functional activation of leukocytes, many of the intracellular signals that mediate these two responses overlap substantially. This chapter illuminates a number of principles that mediate the growth and functional responses of blood cells and their progenitors in health and disease. A better understanding of how blood cells respond to their environment can lead to improved strategies to intervene in pathologic processes in which too many or too few blood cells are produced, or in which the functional activation of blood cells is insufficient or overly exuberant and leads to disease. Moreover, a thorough knowledge of how the signaling pathways that mediate growth and cell survival are disrupted in the hematologic malignancies has begun to allow the rational intervention in such diseases.
TYPES OF RECEPTORS AND THEIR MECHANISMS OF ACTIVATION
The erythropoietin receptor (EPOR) was cloned in 1989,1 settling several controversies and setting many important paradigms in receptor biology. Like other hematopoietic cytokines of this class (granulocyte colony-stimulating factor [G-CSF], thrombopoietin [TPO], and growth hormone [GH]), EPO binds to a homodimeric receptor2,3 with picomolar affinity.4 Numerous studies in these and multiple other cell-signaling systems demonstrate the importance of phosphorylation of vital cytoplasmic mediators in signal transduction,5,6,7 yet one initial conundrum was that the cloned EPOR bears no kinase domain.1 Rather, subsequent studies revealed that the EPOR employs a cytoplasmic kinase of the Janus family (JAK) to initiate signaling.8 JAK kinases bind to the cytoplasmic domain of hematopoietic receptors through motifs termed Box1 and Box2 domains. This information, along with the availability of the tertiary structure of EPOR and of EPO bound to EPOR,9,10 has provided a key insight into the initiation of signal transduction. EPOR exists as a preformed cell surface dimer (Fig. 17–1), in a conformation that separates the two cytoplasmic domains of the subunits (and hence the two tethered JAK molecules). EPO binds sequentially to the two subunits of the preformed EPOR dimer at two distinct faces of the molecule, first to one subunit with the high-affinity face of the ligand (also termed site I), and then to the second subunit of EPOR with a lower-affinity face (termed site II), but an interaction that reduces the off-rate of the ligand. Upon engagement of the two EPOR subunits, a conformational change ensues, shifting the distance between the two cytoplasmic domains of the receptor subunits, that is thought to bring the two inactive JAK molecules into sufficiently close juxtaposition to allow cross-phosphorylation and kinase activation. Once the two tethered JAK molecules are active, multiple additional tyrosine residues become phosphorylated, residues of the receptor itself and those on a number of tethered signaling molecules, events that trigger the totality of cellular EPO responses. Although direct proof for this model of signal initiation is not available for other cytokine receptors of this class, it is widely assumed that a variety of growth factors, interleukins, and hormones activate cellular events in the same manner.
Figure 17–1.
An illustration of cell surface receptors. Each member of the cell surface receptors is depicted as an extracellular region of one or multiple domains, with conserved disulfide bonds indicated by thin cross lines, and the conserved WS box indicated by a thick cross line. The founding member of each receptor class is indicated. EPOR, erythropoietin receptor; GPCR, G-protein–coupled receptor; gp130, glycoprotein 130; IL, interleukin; TGF, transforming growth factor; TNF, tumor necrosis factor. Each receptor subunit illustrated is a single-pass transmembrane protein with the exception of the heptahelical GPCR.
The understanding that a single molecule of EPO can bind simultaneously to two EPOR molecules, and the realization that multiple other cytokines employ a similar stoichiometry of activation has allowed for therapeutic engineering of cytokines into peptide and chemical receptor antagonists. Following EPO binding to a first molecule of EPOR through site I, the receptor conformational change becomes dependent on binding of EPO site II to a second EPOR subunit. By altering the residues at site II, it is possible to block binding at site II, and hence block receptor activation. If site I is altered to increase its affinity for binding to a first receptor subunit so that the affinity of the mutant protein rivals that of the intact molecule, a potent rationally designed antagonist is generated. This strategy has been successfully employed to create pegvisomant, a GH antagonist useful for the treatment of acromegaly.
The engagement of two receptor subunits by a cognate ligand is one mechanism of inducing the receptor conformational change necessary for JAK activation, but several other mechanisms exist that have been exploited by man and nature. Small molecules and dimeric antibodies can induce signaling through the EPOR and at least for the former, can serve as EPO mimetics for therapeutic use.11 Moreover, the 55-kDa glycoprotein (gp55) of the Friend erythroleukemia virus hijacks the EPOR for virus-induced proliferation12 by directly binding to EPOR and (presumably) by inducing the same receptor conformational changes as induced by the authentic hormone. Thus, there are many ways to activate EPOR, and many subtleties dependent on the actual tertiary structural changes induced.13
The interleukin (IL)-6 family of cytokine receptors displays several properties distinct from those of EPOR and its related receptors.14 Unlike the receptors discussed thus far, the IL-6R family members are composed of a heterodimer. The α subunit of each receptor binds cognate ligand with modest affinity, but plays no role in signaling. Instead, a second receptor subunit, glycoprotein (gp)130 (named for its apparent molecular weight [Mr]), or oncostatin-M (OSM) receptor, molecules that alone have no affinity for ligand, but together with the α subunit enhance the binding affinity of the heterodimeric receptor, and are responsible for initiating signal transduction in the presence of ligand.15 In addition, soluble forms of some of the receptors, such as IL-6R, if loaded with IL-6, can bind to cells bearing only gp130 and activate the latter.16 Like EPOR and other members of that subfamily, gp130 and OSM-R engage JAKs to initiate signal transduction.17 Moreover, it is almost certain that the mature IL-6R complex is composed of at least two molecules of IL-6R and two of gp130,18 the latter required to bring the requisite two JAK molecules to the signaling complex. In addition to serving as signaling subunit for IL-6R, gp130 serves as the signaling receptor subunit for IL-11, OSM, leukemia inhibitory factor (LIF), ciliary neurotrophic factor (CNTF), cardiotrophin-1 and -2 (CT-1, CT-2), cardiotrophin-like cytokine (CLC), and IL-27, and OSM-R serves as a signaling subunit for OSM and IL-31. Similar to its role in the IL-6R, gp130 binds to each of these ligands only in the additional presence of a cytokine-specific receptor subunit (e.g., IL-11R, LIF-R) to form a complete signaling receptor. As a consequence of this shared coreceptor physiology, when two or more of the cytokine-specific receptors are present on a cell, the two corresponding ligands can compete for a limiting amount of gp130, and hence for cytokine-specific signaling. This physiology also allows therapeutically engineered cytokine-receptor complexes to stimulate signaling in all cells that express gp130.19 Furthermore, the same principles that allow the rationale design of an EPO or GH antagonist can be used to engineer IL-6 antagonists for treatment of pathologic states dependent on interactions with receptors that require gp130 for receptor signaling.20
The IL-2 family of receptors is also quite complex, in most cases sharing one, two, or three subunits with receptors for other cytokines of the same class (see Fig. 17–1). IL-2Rβ is shared with the IL-15R, and IL-2Rγ (also termed γC [for common]) is shared with the IL-4, IL-7, IL-9, IL-15, and IL-21 receptors.21 Another feature of the IL-2R not yet discussed for the EPOR or IL-6R families is that of a devoted JAK. While JAK2 is employed by all the EPOR subfamily members along with some of the IL-6R subfamily members, and JAK1 and TYK2 are also shared amongst these latter receptors, the fourth and final JAK family member, JAK3, is engaged almost exclusively by γC(one study identified JAK3 activation by IL-8, implying use by the CXCR1 and/or CXCR2 receptors).22 In addition to providing a more fundamental understanding of the principles of signal transduction, careful investigation of the IL-2 family of receptors also has afforded detailed insights into a number of clinically important immunodeficiency states.23 The complexity of this family of receptors was illustrated by the progressive investigation into the origins of severe combined immunodeficiency (SCID).24 As is discussed in Chap. 80, SCID is a severe loss of natural killer (NK) and T lymphocytes, and has been traced to deficiencies of either γC or JAK3, a phenotype recapitulated quite well (but not perfectly) by genetic elimination of the same molecules in mice. However, genetic elimination of IL-2 leads to a phenotype quite different than SCID of humans or engineered mice. Instead, of the multiple cytokines for which γC and JAK3 support signaling, only elimination of IL-7 or the IL-7R recapitulates the phenotype,25,26 a finding now consistent with the finding that IL-7 affects common lymphoid progenitors (Chap. 18), while other cytokines in the family affect more differentiated lymphoid cells.
At present the tumor necrosis factor (TNF) superfamily of receptors and ligands comprises at least 30 receptors and 20 ligands,27,28 and illustrates several novel points in signal transduction pathways: trimeric binding (see Fig. 17–1), receptor promiscuity, and decoy receptors. Although many TNF ligand family members (TNF-α, TNF-β, CD40L [CD154], receptor activator of nuclear factor-κB ligand [RANKL; osteoprotegerin ligand (OPGL)], OX40L, etc.) can bind to several receptors, the ligands are, for the most part, subfamily specific. For example, TNF-α only binds to the six TNF-α receptors and TNF-related apoptosis-inducing ligand (TRAIL) binds to the five TRAIL receptors,29 although it can also bind to the receptor termed osteoprotegerin (OPG). Ligands in this family bind as trimers to homotrimeric receptors, leading to recruitment of secondary signaling molecules to the cytoplasmic domain of the receptors. In general, there are two classes of cytoplasmic domains in these receptors, based on whether they contain the death domain (DD), a region capable of binding signaling mediators that initiate apoptosis (Chap. 15). As such, receptors that do not contain a DD or other signaling domain can function as “decoy receptors,” diverting ligand from initiating programmed cell death in the target cell. For example, among the TNF-α receptors, TNFRI (death receptor [DR]2) contains a DD, and among the five TRAIL receptors, DR4 and DR5 contain DDs, whereas TNFR2 and DcR1 and DcR2 and OPG act as decoy receptors for TNF and TRAIL, respectively. The biologic consequences of ligand binding to individual TNFR family members depend on the relative affinity of their cytoplasmic domains for multiple adaptor proteins; TNF receptor death domain (TRADD) and Fas-associated death domain (FADD) engagement trigger apoptosis pathways, whereas recruitment of one of the seven TNF receptor-associated factor (TRAF) family members leads to activation of transcription factors such as nuclear factor-κB (NF-κB) and kinases such as c-Jun N-terminal kinase (JNK) that lead to cell survival, proliferation, and activation of inflammation.
The receptor tyrosine kinases (RTKs) comprise another class of receptors that contains members vital for hematopoiesis and mature blood cell function (see Fig. 17–1). The first hematopoietic member of this family to be identified was the eukaryotic version of the v-fms oncogene, designated c-fms. Further study revealed that the protooncogene is the sole receptor for macrophage colony-stimulating factor (M-CSF),31 and although somewhat distinct in possessing a split kinase domain, was immediately grouped with other RTKs, such as the receptors for insulin, vascular endothelial cell growth factor and epidermal growth factor, among several others. Subsequently, two additional hematopoietic receptor family members have been identified, c-Kit and Flt-3. These receptors were each cloned based on their homology to the viral oncogene v-kit or c-fms, respectively.32,33 Like all other members of the family, upon engagement of their cognate ligand the kinase domains of homodimeric RTKs become activated, leading to the phosphorylation of receptor cytoplasmic domain tyrosine residues and other tethered substrates. In an apparent example of convergent evolution, like members of the hematopoietic cytokine receptor (HCR) family, RTKs were also found to employ JAKs in their signaling pathways34; as a result, many of the same secondary signaling pathways are activated by both classes of receptors. But perhaps serving as an even more striking example of convergent evolution, the tertiary structure of the index ligand for a hematopoietic RTK, M-CSF, bears substantial homology to essentially all the ligands of the HCR family, such as granulocyte-macrophage colony-stimulating factor (GM-CSF).35
The transforming growth factor (TGF) receptor family consists of seven type I and five type II receptors that heterodimerize to form receptors for multiple TGF-β family members, including the TGF-β/activin/nodal and bone morphogenic protein (BMP) subfamilies. The precise stoichiometry of binding involves a ligand dimer, stabilized by disulfide and/or hydrophobic bonds, and two type I and two type II subunits (see Fig. 17–1); the tertiary structure of the complex has been carefully investigated.36 Both type I and type II receptors contain an N-terminal ligand binding, transmembrane, and cytoplasmic ser/thr kinase domains; the type I receptors additionally contain a Gly/Ser (GS)-rich domain.36 For TGF-β subfamily members, the type II subunit bears a high-affinity ligand binding site, which on TGF-β or activin engagement recruits type I receptors, bringing the two cytoplasmic domains into close juxtaposition, enabling the type II kinase to phosphorylate Ser residues on the type I receptor GS domain, thereby activating the type I kinase. Cell-surface-bound coreceptors also exist and aid in generating the signaling complex for TGF-β, but not activin or BMP ligands. For BMP family members, the type I receptor bears the high-affinity ligand binding site, such that BMP initially binds to type I receptor, with the type II subunit subsequently recruited to form the signaling complex. Once the two receptor kinases are activated, they recruit and phosphorylate the SMAD (Sma- and Mad-related protein) adaptor proteins, allowing their nuclear translocation and transcriptional activation. However, SMAD-independent TGF-β signaling pathways also exist.37
Several molecules that play essential roles in blood cell development or function signal by engaging G-protein–coupled receptors (GPCRs), the largest family of cell surface receptors in organisms as diverse as yeast and humans, estimated to comprise approximately 1000 distinct gene products, or approximately 3 percent of the human genome. Also termed serpentine or heptahelical receptors (for their seven transmembrane domains that form four extracellular and three intracellular loops; see Fig. 17–1), GPCRs are so named because they use three small guanine nucleotide (or G)-binding proteins (Gα, Gβ, and Gγ) for signal transduction. In the unstimulated state, all three G proteins bind to the intracellular loops of the receptor. Individual ligands engage GPCR in one of many different ways. For example, small lipophilic molecules (e.g., epinephrine) bind to transmembrane (TM) domains of the receptor, disrupting the interactions between TM3 and TM6, leading to conformational changes that alter G-protein binding.38 Other GPCRs use additional extracellular domains (e.g., the “Venus flytrap” domain)39 to bind and dimerize receptors. Still others, which are engaged by proteases, are activated by protease cleavage of the receptor amino terminus, leading to the “unmasking” of a hexapeptide at the new amino terminus, which then interacts with one of the receptor extracellular or TM domains.40 By each of these and other mechanisms a conformational change occurs in the GPCR, allowing monomeric Gα and dimeric Gβγ complexes to dissociate from the intracellular loops and each to engage secondary signaling pathways.41 Examples of critical molecules that employ GPCRs and display hematologic activity are thrombin, adrenergic hormones, and chemokines. The outcomes of such engagement include cellular growth and survival, functional activation, and migration.
Although adhesion molecules were named for the vital structural role they play in tissue cohesion, physically bridging cells in the marrow with each other and with extracellular matrix macromolecules, and at sites at which mature blood cells interact with the endothelium, engagement of blood and progenitor cell integrins and other adhesion molecules also generates vital cellular signals that affect their survival, proliferation, and functional activation.42,43,44 In fibroblasts, cell adhesion is most clearly manifest at contact sites termed focal adhesions, and the signaling complexes that form on cytoplasmic domains of the integrins that support them are termed focal adhesion complexes.45 Within such complexes are components of the actin cytoskeleton, kinases both specific for focal adhesions and several others found in other cytoplasmic sites,46,47,48 and a number of scaffolding molecules upon which adhesion strengthening and signaling take place. Moreover, growth factor receptors functionally interact with integrins, adding to signaling complexity. Thus, adhesion molecules must also be considered as signaling receptors.
Nuclear receptors (NRs) are nascent transcription factors that play a wide variety of roles in cellular physiology by binding small lipophilic hormones. Some NRs, such as glucocorticoid hormone receptors, remain sequestered in the cytoplasm in the absence of their cognate ligand, and upon ligand engagement translocate to the nucleus and bind and activate palindromic, direct repeat, or inverted palindromic sequences that comprise nucleotide hormone response elements.49 Other NRs, such as receptors for vitamin A metabolites (retinoids), remain bound to nuclear DNA and repress transcription, until engaged by ligand upon which nuclear coactivators are recruited leading to enhancement of gene transcription.50,51,52 Although sex, glucocorticoid, and thyroid hormones may play subtle roles in blood cell biology, retinoid receptors, which most commonly bind as heterodimers with the RXR receptor to retinoid response elements of the form PuGTTCA(N)2,5PuGTTCA, play vital developmental roles in a myriad of cell systems, and play similar roles in hematopoiesis. Amongst the hematopoietic targets of retinoid receptors are c-myc, C/EBPε, and p21.53 However, because this class of receptors represents a nearly direct pathway from stimulus to response, without intervening signaling, they are not discussed further in this chapter.
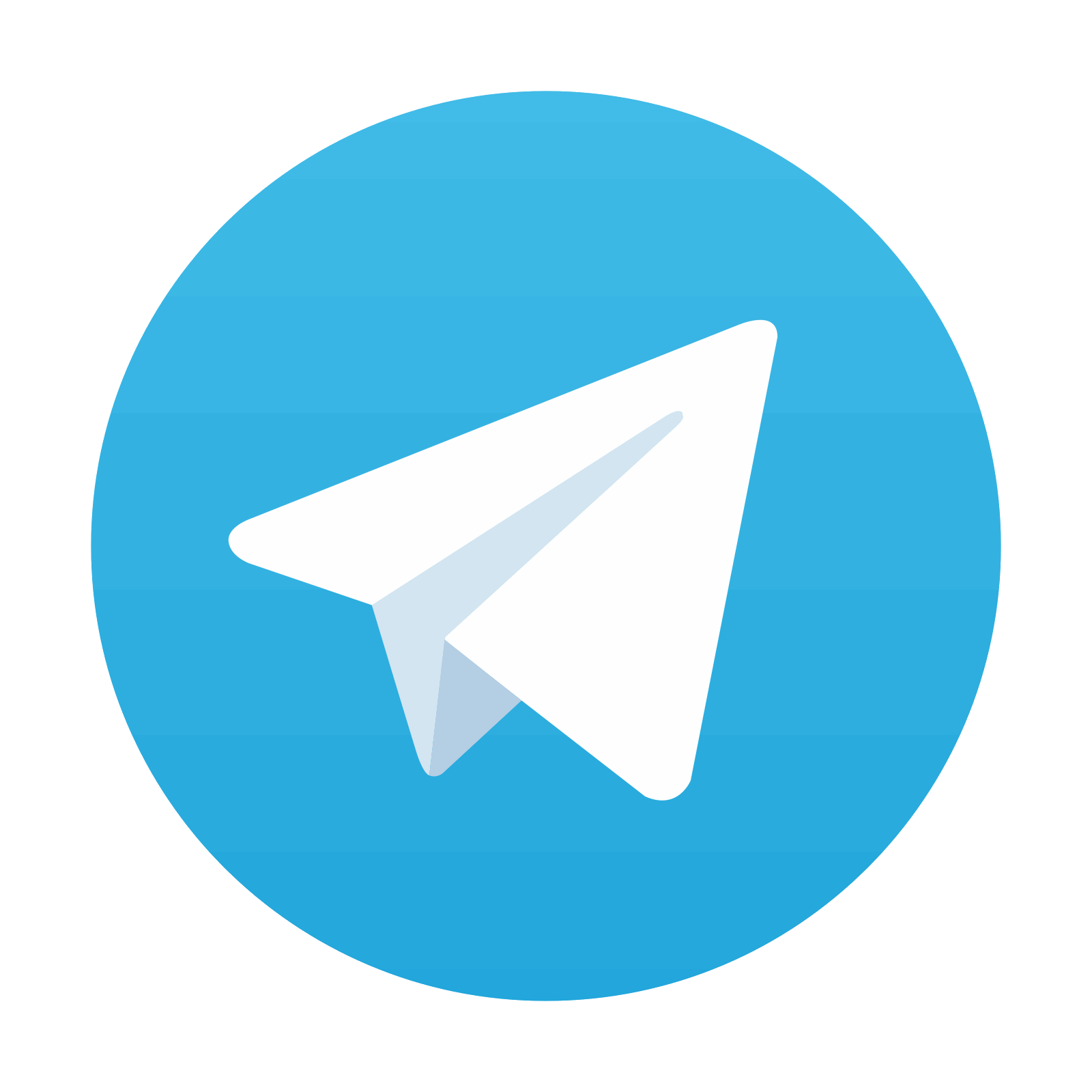
Stay updated, free articles. Join our Telegram channel

Full access? Get Clinical Tree
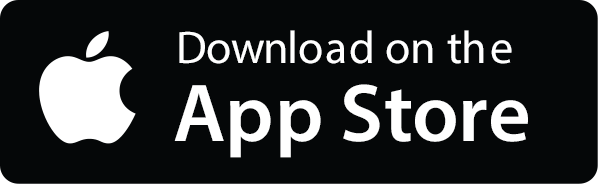
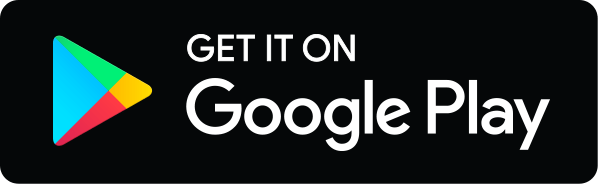