Screening and Early Detection
▪ 6A Early Detection of Prostate Cancer with PSA
Stacy Loeb
William J. Catalona
INTRODUCTION
Prostate cancer is the leading noncutaneous cancer diagnosis, as well as the second-leading cause of cancer death among men in the United States (1). Currently, most prostate cancer cases are identified through prostate-specific antigen (PSA)-based screening. Screening involves testing an asymptomatic population with the objectives of earlier disease detection, thereby improving outcomes. This chapter reviews the use of PSA and its derivative measurements and discusses its ability to meet these objectives of screening.
HISTORY OF PSA SCREENING
PSA was initially used in forensic science as a marker for human semen (2). Later studies determined that it was prostate-specific (3) and that serum levels could be measured as a marker for prostatic disease (4,5). In medicine, PSA was first used to monitor prostate cancer and subsequently as a tool for screening (6,7).
The first large-scale study of PSA screening began in 1989 and initially involved a sequential protocol with PSA testing followed up by a confirmatory digital rectal examination (DRE) and/or transrectal ultrasonography (TRUS) (7). The results of this study showed that PSA and DRE were the optimal two-test combination for use in early detection. Accordingly, the protocol was modified to include PSA and DRE as simultaneous screening tests, as they continue to be utilized in contemporary practice. Indeed, a proportion of cancers are detected by either PSA alone or, less frequently, DRE alone, illustrating their complimentary nature (8). Important findings from this screening study include that the risk of prostate cancer increases with increasing PSA levels. Also, prostate cancer found at lower PSA levels has more favorable pathologic features and treatment outcomes (9).
The ability of PSA to stratify prostate cancer risk is well established. Thompson et al. reported a significant direct relationship between PSA and prostate cancer detection among men undergoing empiric biopsy in the Prostate Cancer Prevention Trial (PCPT) (10). For example, 6.6% of biopsies were positive at PSA levels ≤0.5 ng/mL compared to 26.9% at PSA levels of 3.1 to 4.0 ng/mL.
PSA is also well validated as a predictor of future prostate cancer risk. This concept was first demonstrated in the Physician’s Health Study (11). Compared to the reference group with a baseline PSA level <1 ng/mL, men with baseline PSA levels of 1 to 1.5, 1.5 to 2, 2.0 to 3.0, and 3.0 to 4.0 ng/mL had a 2.2-, 3.4-, 5.5-, and 8.6-fold increased risk, respectively, of later being diagnosed with prostate cancer outside a screening study. Similarly, among men from a Swedish cardiovascular study, a baseline PSA measurement at age 44 to 50 years predicted future prostate cancer risk (12). In the Baltimore Longitudinal Study of Aging, men in their 40s with a baseline PSA level greater than the median of 0.6 ng/mL had a 3.75-fold increased relative risk of prostate cancer as compared with those with a lower baseline PSA (13). This relationship between the PSA level at a young age and future prostate cancer risk has also been confirmed in young community men from the Child Health and Development Study (14), as well as in single institution series (15) and in large prostate cancer screening populations (16). Some professional organizations such as the National Comprehensive Cancer Network (NCCN) incorporate a baseline PSA measurement at age of 40 years into screening protocols to assess future risk (17).
Finally, the correlation of PSA levels to prostate cancer aggressiveness has been extensively studied and reported. The Gleason score on biopsy has a significant direct association with increasing PSA levels. For example, in the PCPT, 12.5% of cancers detected at PSA levels ≤0.5 ng/mL were high-grade, compared to 25% at PSA levels of 3.1 to 4.0 ng/mL (10).
Along with clinical stage and biopsy features, the PSA level at diagnosis is incorporated into virtually all major prognostic nomograms. For example, the Partin tables (18), Kattan nomogram (19), and Cancer of the Prostate Risk Assessment (CAPRA) score (20) all include PSA to help predict pathologic tumor features, biochemical progression, and/or disease-specific mortality.
Indeed, PSA is frequently used to predict prognosis following prostate cancer treatment. For example, in a large series of men undergoing radical prostatectomy at PSA levels ≤4, 4.1 to 10, 10.1 to 20, and >20 ng/mL, the 10-year biochemical progression-free survival rates were 91%, 79%, 57%, and 48%, respectively (21). In a recent multi-institutional radical prostatectomy series, PSA also predicted the risk of prostate cancer-specific mortality (PCSM) at 15 years (22). Men with preoperative PSA levels <4 had a 4% 15-year PCSM rate compared to 11% with a PSA of 10.1 to 20 ng/mL, and 22% with a PSA of 20.1 to 50 ng/mL.
PSA DERIVATIVE MEASUREMENTS
Numerous adjunctive PSA-based measurements have been described for use in prostate cancer screening. PSA circulates in both “free” and protein-bound forms within the bloodstream (23). The proportion of PSA that circulates in the free form (or percent free PSA, %fPSA) was shown to help differentiate prostate cancer from benign prostatic conditions. In an early multi-institutional study of %fPSA in screening for men with PSA levels of 4 to 10 ng/mL, the median %fPSA was 12% in men diagnosed with prostate cancer compared to 18% in men with benign disease (p < 0.001) (24). On receiver operating characteristic analysis, %fPSA was associated with an area under the curve of 0.72 (95% CI 0.68-0.75) in this population.
Another way to examine PSA is in relation to the prostate volume. Veneziano et al. showed that men with prostate cancer
had a higher PSA-to-volume index (1.73) compared to men with Benign prostatic hyperplasia (BPH) (0.099) or controls (0.090) (25). Benson et al. first described the use of “PSA density” to distinguish prostate cancer from BPH (26,27). In this study, prostate volume measurements were based upon magnetic resonance imaging (noncancers) and the surgical specimen (cancers). Although MRI may measure prostate size more accurately (28), numerous subsequent studies have confirmed the utility of PSA density (PSAD) in prostate cancer screening instead using TRUS prostate volume estimates that are more readily available in clinical practice (29,30). Moreover, PSAD has been associated with disease prognosis in men undergoing various forms of prostate cancer management, including active surveillance and definitive treatment (31,32). In a radical prostatectomy population, favorable pathology (organ-confined disease, Gleason score <7, and ≤10% cancerous involvement of the specimen) was found in 74% with a preoperative PSAD ≤0.15 as compared with only 36% with a PSAD >0.15 (30).
had a higher PSA-to-volume index (1.73) compared to men with Benign prostatic hyperplasia (BPH) (0.099) or controls (0.090) (25). Benson et al. first described the use of “PSA density” to distinguish prostate cancer from BPH (26,27). In this study, prostate volume measurements were based upon magnetic resonance imaging (noncancers) and the surgical specimen (cancers). Although MRI may measure prostate size more accurately (28), numerous subsequent studies have confirmed the utility of PSA density (PSAD) in prostate cancer screening instead using TRUS prostate volume estimates that are more readily available in clinical practice (29,30). Moreover, PSAD has been associated with disease prognosis in men undergoing various forms of prostate cancer management, including active surveillance and definitive treatment (31,32). In a radical prostatectomy population, favorable pathology (organ-confined disease, Gleason score <7, and ≤10% cancerous involvement of the specimen) was found in 74% with a preoperative PSAD ≤0.15 as compared with only 36% with a PSAD >0.15 (30).
By definition, PSAD requires prostate volume measurement. However, neither TRUS nor MRI is utilized as a primary screening tool, and prostate volume estimation by DRE is unreliable. A poor correlation of 0.27 has been demonstrated between DRE volume estimates and prostatectomy specimen weight (33). Accordingly, PSAD may be more practically useful in men with a volume measurement from a prior prostate biopsy, such as those undergoing repeat biopsy for cancer detection or active surveillance (31).
A third way to use serial PSA measurements is a calculation of PSA velocity (PSAV), or the change in PSA units per year (ng/mL/year). This concept was first tested in the Baltimore Longitudinal Study of Aging to distinguish prostate cancer from BPH (34). More recently, Carter et al. reported that a PSAV greater than 0.35 ng/mL/year predicted long-term disease-specific mortality more than a decade before prostate cancer diagnosis (35). PSAV in the year before prostate cancer diagnosis has also been shown to predict cancer-specific mortality after radical prostatectomy as well as after radiation therapy for localized disease (36,37).
However, some studies have reported conflicting results with regard to the utility of PSAV. For example, in a large radical prostatectomy population, O’Brien et al. reported an association between PSAV and treatment outcomes; however, they found that it did not substantially enhance prediction beyond PSA alone (38). Due to the colinearity between PSA and PSAV, it can be difficult to statistically demonstrate independent predictive value. Some of the differences between studies may also relate to the time interval and number of component PSA measurements used in the calculation. The latter differences are important because increments in PSA over time are often not linear. Thus, the PSAV of an individual may vary, depending upon the interval over which it is calculated.
Another important issue is that PSA kinetics calculations are not always possible in men without a lengthy PSA history. Nevertheless, a benefit of baseline PSA testing of men in their 40s is also the ability to establish a baseline PSA velocity (39). Alternatively, it is possible that PSAV has greater prognostic significance among men with low total PSA levels and may increase the specificity of screening for clinically significant disease more in this setting (40).
PSA dynamics can also be reported as PSA doubling time (PSADT), or the time required for the PSA level to double. Shorter PSADT has been associated with more aggressive prostate cancer in some studies but not others (38,41,42,43). Overall, there is ongoing controversy regarding the utility of PSA kinetics measurements and which method of calculation has the greatest prognostic value (44).
More recently, several free PSA isoforms have been described whose relative proportion may relate to prostate cancer risk. Specifically, two isoforms—“B”-PSA and “i”-PSA—have been associated with BPH; whereas, the “pro” PSA isoforms comprise a greater percentage of free PSA in the tissue and serum in men with prostate cancer (45,46). Numerous recent studies have suggested a possible role for proPSA isoforms to increase the specificity of screening, particularly in the diagnostic gray zone (47,48,49,50). The [-2]pro-PSA isoform has been approved as an adjunct to screening in Europe and is undergoing additional study in the United States.
Finally, there has been recent study into the interplay between genetic factors with PSA levels in screening. A rapidly increasing number of single nucleotide polymorphisms (SNPs) have been identified, which are associated with prostate cancer susceptibility (51). Some of these SNPs are located near the PSA gene, and the risk of prostate cancer at a given PSA level may differ based upon genotype (52). Despite the commercial availability of personal genomics testing, additional study will be necessary to examine whether the incorporation of genetics information can improve the outcomes of screening.
CONTROVERSIES RELATED TO PSA SCREENING
No screening test is perfect, and PSA is no exception. The first major drawback is the limited specificity of PSA, since it may also be elevated from urinary tract manipulation (e.g., prostate biopsy, catheterization) or benign prostatic conditions, such as benign hyperplasia or prostatitis (53). For example, BPH is prevalent in older men and can lead to PSA elevations. However, even in men with highly symptomatic BPH, the average PSA change is approximately 0.15 ng/mL/year (54), a substantially lower PSA velocity than in most prostate cancers (34). Thus, the specificity of PSA-based screening can be improved through the use of adjunctive PSA-based measures.
Both symptomatic and asymptomatic prostatitis may also lead to PSA elevations, which can confound the use of PSA and PSA kinetics (55,56). Accordingly, there have been several studies evaluating the use of empiric antibiotics to decrease the number of unnecessary biopsies triggered by prostatitis. Although some studies have shown lower rates of prostate cancer detection among men with a PSA decrease following antibiotics (57), other studies have reported conflicting results (58), and the practice remains controversial (59). An alternative approach is to perform repeated PSA measurements after a period of observation, in light of the short-term variability in PSA (60).
Another major issue is the diagnosis and treatment of some tumors detected through screening that would not have caused harm. Similar concerns have been raised in other screening programs. For example, there is major ongoing controversy regarding breast cancer overdiagnosis through mammography, with estimates of up to 52% including carcinoma in situ (61). Pap smears for cervical cancer screening also detect some atypical lesions with ultimate spontaneous regression (62).
With regard to the magnitude of “overdiagnosis” for prostate cancer, Etzioni et al. reported that 15% and 37% of autopsy tumors would have been detected through PSA screening in white and black men, respectively (63). More recently, Welch and Albertsen used incidence and mortality trends from 1986 to 2005 to estimate substantial rates of overdiagnosis in the past two decades. They estimated that at least 20 men had to be diagnosed with prostate cancer to prevent one prostate cancer death (64). However, the inclusion of more recent years in the denominator for mortality may have led to an overestimation of overdiagnosis. In addition, these estimates do not take into account temporal trends (e.g., decreasing mortality from cardiovascular disease) and exclusively consider the endpoint of mortality. Indeed, reducing the burden of locally
advanced and metastatic disease is also a critical goal. Not only does metastatic prostate cancer have profound implications on quality of life, but there are also limited therapeutic options as the disease progresses. Screening has been shown to result in substantial reductions in advanced/metastatic disease (65).
advanced and metastatic disease is also a critical goal. Not only does metastatic prostate cancer have profound implications on quality of life, but there are also limited therapeutic options as the disease progresses. Screening has been shown to result in substantial reductions in advanced/metastatic disease (65).
In addition to screening, improvements in prostate cancer treatment account for a portion of the declining prostate cancer mortality rates. In a randomized Swedish trial, radical prostatectomy was shown to reduce metastasis and cancer-specific mortality compared to watchful waiting (66). However, sufficiently early diagnosis at a localized stage is necessary to allow the use of effective treatment; as such, screening and curative therapy are not mutually exclusive and contribute jointly to reducing morbidity and mortality from prostate cancer.
Another limitation of screening is the potential for false-positive results. As mentioned, numerous noncancerous conditions and interventions lead to PSA elevations, including infection, catheterization, and benign enlargement. DRE may also have false-positive results. Even when prostate cancer is detected following an abnormal DRE, “serendipitous” cancer may be found in a different region of the prostate from the palpable abnormality (67).
Abnormal screening results are usually followed up by prostate biopsy to obtain a histologic diagnosis. This confirmatory procedure itself may be associated with pain, anxiety, bleeding, and/or infectious complications. Indeed, a recent large-scale Canadian study reported a significant increase in the rate of infectious complications following prostate biopsy (68), highlighting the importance of this issue to contemporary practice. Increasing the specificity of screening practices is crucial to reduce unnecessary biopsies.
RESULTS OF PSA SCREENING
One of the most well-documented consequences of PSA screening is a stage migration. The rates of metastatic disease at diagnosis have declined drastically (by ˜75%), with corresponding increases in the proportion of organ-confined disease. Age-adjusted PCSM rates have also decreased substantially (by more than 40% during the PSA era in the United States) (69). These trends have been most apparent in the United States and other countries where PSA screening is widely practiced, compared to countries where screening is not routinely performed (70). For example, van Leeuwen et al. examined the rates of metastases and prostate cancer death between a screening population versus Northern Ireland (where only 6% of the population is screened) (71). Overall, metastasis and disease-specific death rates were 53% and 37% lower in the screening group.
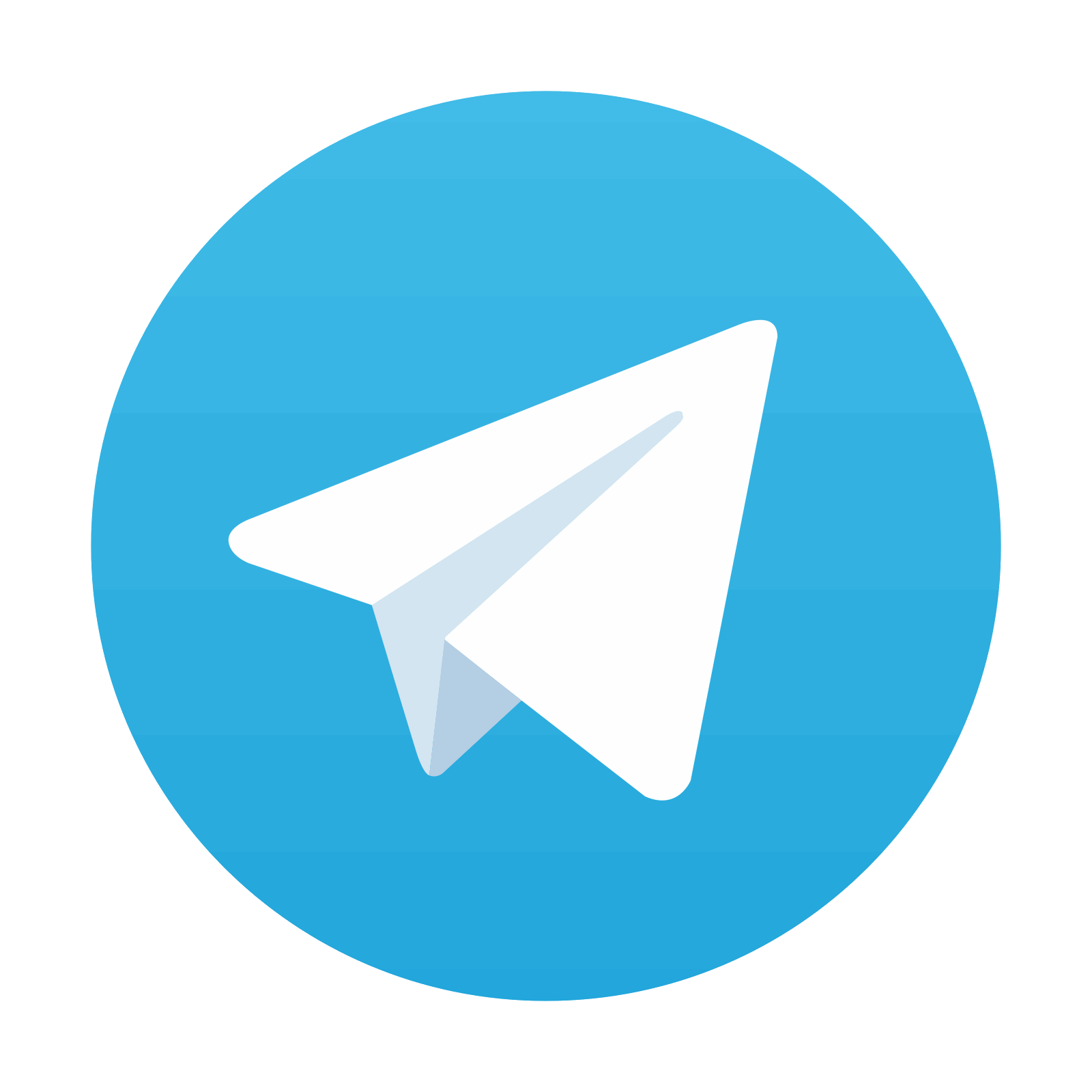
Stay updated, free articles. Join our Telegram channel

Full access? Get Clinical Tree
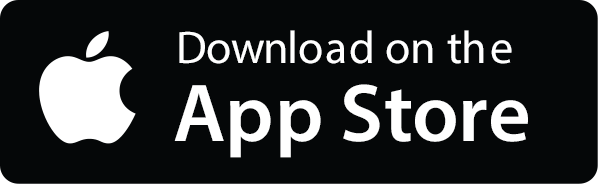
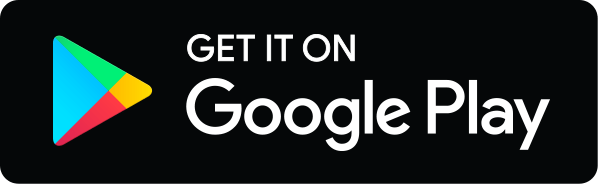