FIGURE 26.1 Sarcoma subtypes discussed in the text. Upper panels, hematoxylin and eosin–stained paraffin sections. Malign., malignant. Lower panels are fluorescence in situ hybridization images showing (left) alveolar rhabdomyosarcoma with fusion of probes for PAX3 (red) and FOXO1 (green) and (right) Ewing sarcoma with break-apart of probes flanking the EWS breakpoint region, EWSR1.
FIGURE 26.2 Nucleotide and copy number alterations in soft tissue sarcoma. The outer ring indicates chromosomal position. The second through fifth rings represent four subtypes with complex karyotypes (as labeled; MYXF, myxofibrosarcoma; PLEO, pleomorphic liposarcoma; LMS, leiomyosarcoma; DEDIFF, dedifferentiated liposarcoma). The three inner rings represent subtypes with simple karyotypes (Myxoid, myxoid/round cell liposarcoma). The plots show the statistical significance of genomic aberrations, with amplification in red and deletion in blue. Green curves indicate the chromosomal breakpoints of pathognomonic translocations in myxoid/round-cell liposarcoma and synovial sarcoma. Genes harboring somatic nucleotide alterations are indicated with green circles whose size is proportional to their frequency of occurrence. (Courtesy of Barry S. Taylor, Computational Biology Center, Memorial Sloan-Kettering Cancer. Adapted from ref. 19.)
Clinically, evidence for FUS-DDIT3 translocations from reverse transcription polymerase chain reaction (RT-PCR)15 or fluorescence in situ hybridization (FISH)16 can help confirm the diagnosis and may be useful for small biopsies dominated by a round cell component. Fusion subtype, however, appears to have little prognostic value beyond what is known from stage and grade. In general, molecular markers in myxoid liposarcoma have been difficult to test for independent prognostic significance, given the difficulty of assembling large series with long follow-up.17 Nevertheless, p53, IGF1R/IGF2, and RET overexpression may be adverse factors.13,18 Such findings support IGF/Akt/mTOR and Ras-Raf-ERK/MAPK pathway inhibitors as potential targeted therapies in myxoid liposarcomas. In addition, mutations in PIK3CA, which were found in 18% of myxoid/round cell liposarcomas, were associated with worse outcome.19
TABLE 26.1
CYTOGENETIC AND MOLECULAR ABNORMALITIES IN SOFT TISSUE SARCOMAS
The dense microvasculature and high levels of IL-810 and vascular endothelial growth factor (VEGF)20 expression seen in this tumor may underlie its observed sensitivity to radiotherapy21 and trabectedin,22 suggesting a value for antiangiogenic therapies. Trabectedin may also function by disrupting the binding of FUS-DDIT3 to target promoters.11
Ewing Sarcoma
Ewing family tumors appear most commonly in adolescents and young adults; primary sites can be either bone or soft tissues. A range of aggressive small, blue, round cell tumors with variations in clinical and morphologic features have been subsumed under the general term Ewing sarcoma family tumor following the recognition of common pathognomonic chromosomal translocations.23,24 EWSR1, the common 5’ translocation partner, is fused to one of several possible ETS family transcription factor genes (usually FLI1). In the fusion protein, EWSR1 provides, at minimum, its 264 amino acid N-terminal transcriptional regulatory domain, and the ETS factor provides its C-terminal DNA-binding domain. In the process, EWSR1 loses its RNA recognition domain, and the ETS factor loses its native transactivation domain. Several direct transcriptional targets for the fusion oncoprotein are supported by strong evidence. Some of these targets are up-regulated (ID2,25 PTPL1,26 MK-STYX,27 DAX128) and some repressed (CIP1,29 TGFBR2,30 IGFBP331) in Ewing sarcoma, but gene repression events, mediated by cofactors, appear to predominate overall.32 The net result is activation of pathways driving proliferation and cell survival (including IGF signaling33), with concurrent repression of pathways promoting mesenchymal differentiation.34,35
Molecular confirmation of an EWSR1 translocation can be critical for patient management because many of the clinical, morphologic, and immunophenotypic features of Ewing sarcoma are shared with entities such as mesenchymal chondrosarcoma and small cell osteosarcoma. Commercially available EWSR1 split-apart FISH probes are valuable ancillary diagnostic tools (Fig. 26.1).36 RT-PCR alternatives are complicated by the need to cover the many alternative gene and exonic fusion sites, but RT-PCR offers the advantage of identifying the specific exon fusion involved, which can have independent prognostic relevance.37
Several existing agents (including IGF/mTOR and histone deacetylase inhibitors38–40) that target recently identified, translocation-induced mechanisms and pathways are currently being tested in clinical trials for Ewing sarcoma, and novel strategies to directly inhibit the oncoprotein itself are in active development.41
Desmoplastic Small Round Cell Tumor
In desmoplastic small round cell tumor, the same 5’ portions of EWSR1 involved in Ewing sarcomas are fused to WT1,42,43 a tumor suppressor deleted in Wilms tumor.44 The 56-kDa EWSR1-WT1 chimeric protein includes the last three of the four WT1 DNA-binding zinc finger domains. Despite some similarities to Ewing sarcoma family tumors, desmoplastic small round cell tumors show little response to conventional chemotherapy. Prognosis is dismal, and new therapies are needed.45 Several transcriptional targets of the EWSR1-WT1 chimeric oncoprotein have been identified.43 PDGFA expression is directly induced by EWSR1-WT1,46 explaining the desmoplastic background and possibly the recent observation of a partial response to sunitinib.47 IL2RB is also induced, and its downstream JAK/STAT signaling pathway appears active in this tumor,43,48 representing a potential target for novel treatment approaches.
Synovial Sarcoma
Synovial sarcoma differs from most translocation-associated sarcomas in that the genes involved in its defining translocation, t(X;18)(p11;q11), encode epigenetic regulators, not transcription factors with direct DNA-binding activity.49 The translocation fuses the widely expressed SYT (aka SS18) gene with an SSX gene normally expressed only in testis.50 In the fusion oncoprotein, SYT, which interacts with components of the SWI/SNF chromatin remodeling complex,51 retains all but the last eight amino acids from its C-terminal transcription activation domain. The SSX partner (SSX1, 2 or 4) retains only its C-terminal 78-residue repressor domain, which confers nuclear localization in association with Polycomb group proteins that mediate chromatin condensation and epigenetic gene silencing.52 The resulting chimeric oncoprotein dysregulates transcription at the level of epigenetic modifications53 and, when forcibly expressed in a mesenchymal stem cell background54 or conditionally expressed in mice,49,55 recapitulates synovial sarcoma. Direct targets of SYT-SSX include the tumor suppressors COM156 and EGR157; both genes are repressed in synovial sarcoma, with the EGR1 promoter undergoing SYT-SSX-dependent histone methylation.57 Thus, transcriptional reactivation agents such as histone deacetylase inhibitors are worth investigation in this disease, as they may reactivate mesenchymal differentiation and reverse some effects of the SYT-SSX oncoprotein.58,59
The synovial sarcoma oncoprotein may also function by disrupting normal interactions between transcription factors and their DNA-binding sites, such as interactions between SLUG/SNAIL and the E-cadherin promoter, leading to transcriptional activation.60 Subtle differences in such interactions may underlie the propensity of SYT-SSX1 translocations, compared to SYT-SSX2 translocations, to be associated with biphasic histology and poorer outcome, although prognostic differences are controversial.61,62 The need for diagnostic molecular translocation testing, however, may be obviated by assays for TLE1, a transcriptional corepressor63 that is highly expressed in synovial sarcoma and serves as a sensitive and specific biomarker.64 Other oncogenic pathways that are activated, directly or indirectly, in synovial sarcoma include IGF2,54,65,66 suggesting potential value for IGF/Akt/mTOR inhibitors in this disease.
Alveolar Rhabdomyosarcoma
In alveolar rhabdomyosarcoma, an aggressive cancer of older children and adolescents, the transcriptional activation domain of FOXO1 (aka FKHR) from 13q14 is fused to the DNA-binding domain of paired box transcription factor PAX3 (2q35) or PAX7 (1p36).67,68 Until recently, about 20% of cases were thought to be translocation-negative, but recent work has proven that such tumors in fact represent histologic variants of embryonal rhabdomyosarcoma.69 Furthermore, those cases with translocations involving PAX3 have a considerably worse prognosis than those involving PAX7.70 Thus, molecular confirmation of the diagnosis by FISH (Fig. 26.1) and/or RT-PCR is required to optimize patient care.71 Either translocation results in high-level nuclear expression of a chimeric transcription factor that abnormally activates PAX targets, many of which are genes involved in neurogenesis that are not expressed in normal skeletal muscle.72 In addition, PAX3-FOXO1 directly induces PDGFRA; small-molecule (imatinib) and antibody inhibitors of this receptor tyrosine kinase are effective in mouse models.73 Another probable direct target of PAX3-FOXO1, based on comparison of primary tumor and mouse model expression profiles, is the cell-cycle regulator SKP2,74 perhaps helping explain why alveolar rhabdomyosarcoma is responsive to conventional cytotoxic chemotherapy.
Alveolar Soft-Part Sarcoma
Alveolar soft-part sarcoma has a clinical presentation and pathognomonic molecular event with many similarities to other translocation-associated sarcomas.75 In this disease, the 5’ half of the widely-expressed ASPSCR1 (aka ASPL) gene on 17q25 is fused to exon 3 or 4 of TFE3 on Xp11, the latter retaining its transcriptional activation, basic helix-loop-helix, and leucine zipper domains.76 Interestingly, the same fusion is present in some renal cell carcinomas.77 Although alveolar soft-part sarcoma has distinctive histology, translocation detection by RT-PCR or by immunohistochemistry for TFE3 can serve as a diagnostic adjunct.78,79 The disease lacks validated prognostic biomarkers. Direct targets of ASPSCR1-TFE3 are not yet identified, although gene expression profiling and tissue microarray studies have highlighted prominent activation of c-Met signaling and angiogenesis pathways.80–82 Antiangiogenic targeted therapy is effective in xenograft models83 and has yielded partial responses in patients with metastatic disease.84
Dermatofibrosarcoma Protuberans
The cytogenetic hallmark of dermatofibrosarcoma protuberans (DFSP) is supernumerary ring chromosomes that contain material from chromosomes 17 and 2285–87 or, less commonly, an unbalanced der(22)t(17;22)(q21-23;q13). The molecular consequence of both types of aberration is the overexpression of the platelet-derived growth factor-beta (PDGFB) gene on chromosome 22, through fusion with the collagen gene COL1A1 on chromosome 17.88,89 The same fusion gene is also seen in two histologic variants: giant cell fibroblastoma and Bednar tumor (pigmented DFSP). FISH and comparative genomic hybridization (CGH) studies have indicated that increased COL1A1–PDGFB copy number is associated with fibrosarcomatous transformation of DFSP, although the copy number increase is not an invariable feature of these cases.90,91
The COL1A1-PDGFB fusion product signals through the PDGF receptor in an autocrine loop.92 This signaling can be blocked using tyrosine kinase inhibitors acting at PDGFR, such as imatinib. A number of clinical studies have shown a high response rate to imatinib therapy in both locally advanced and metastatic DFSP.85,93,94 These results support the concept that DFSP cells depend on aberrant activation of PDGF signaling for proliferation and survival.
Extraskeletal Myxoid Chondrosarcoma
Most extraskeletal myxoid chondrosarcomas show one of four reciprocal translocations: t(9;22)(q22;q12), t(9;17)(q22;q11), t(9;15)(q22;q21), or t(3;9)(q12;q22), with t(9;22) being the most common. These translocations fuse NR4A3 in 9q22-q31.1 with either EWSR1 in 22q12, TAF15 in 17q11, TCF12 in 15q21, or TFG in 3q12.95–97 Because these fusion genes have not been described in any other tumor type, they represent useful diagnostic markers. The four different fusion partners have unknown prognostic significance.
NR4A3 encodes a ubiquitously expressed orphan nuclear receptor also known as NOR-1, TEC, MINOR, or CHN.98 The t(9;22) fuses the transactivation domain of EWSR1 to the full length of NR4A3. Analogous to EWSR1-ETS fusions, the EWSR1-NR4A3 fusion protein not only displays strong transcriptional activity, but also regulates RNA splicing.99
TAF15, like EWSR1 and FUS, belongs to the TET family, and contains a characteristic 87-amino acid RNA recognition motif implicated in protein-RNA binding.100 The N-terminal regions of EWSR1, FUS, and TAF15 contain degenerate repeats of the SYGQ motif and mediate powerful transcriptional activation when fused to the heterologous DNA-binding domains of a variety of transcription factors.101
By gene profiling, extraskeletal myxoid chondrosarcomas constitute a distinct genomic entity, showing up-regulation of several genes, including NMB, DKK1, DNER, CLCN3.102 In situ hybridization confirmed that NMB is highly expressed in extraskeletal myxoid chondrosarcoma but not in other sarcoma types, suggesting its potential value as a diagnostic marker. Somewhat surprisingly, the up-regulated genes seen in two different profiling studies had only limited overlap.96,102
SIMPLE KARYOTYPE TUMORS ASSOCIATED WITH MUTATIONS
Desmoid Fibromatosis
Desmoid fibromatoses are locally infiltrative, clonal fibroblastic proliferations that arise in the deep soft tissues and never metastasize. Although about 70% result from mutations in APC or CTNNB1, tumorigenesis may be also be influenced by endocrine and physiologic factors such as pregnancy, trauma, and prior surgery. Desmoids are usually divided into two groups: sporadic desmoids and those in individuals with a heterozygous germline mutation in the adenomatous polyposis coli (APC) gene (chromosome 5q). Although germline APC mutations also often result in familial adenomatous polyposis,103 some desmoid patients harboring such a mutation have no polyposis. The desmoids in individuals with a germline APC mutation display inactivation of the second copy of APC, which usually occurs by point mutation or deletion.103,104
Among sporadic desmoids, only a minority display APC inactivation. A majority (52%–85%) have an activating point mutation in the β-catenin gene, CTNNB1.105,106 These CTNNB1 mutations stabilize β-catenin, resulting in its overabundance. β-catenin, a mediator of Wnt signaling, is negatively regulated by APC, so both APC inactivation and CTNNB1 activating mutations result in up-regulation of the Wnt pathway. The specific CTNNB1 mutation may have prognostic significance; patients with S45F-mutant desmoids were reported to have a 5-year recurrence-free survival of only 23%, compared with 57% for those with T41A-mutant tumors and 65% for those with wild type CTNNB1.106 These results raise the possibility that mutation status might aid in selecting patients for more aggressive therapy.
Based on the findings of APC inactivation or activating CTNNB1 mutations in the majority of patients, the development of small-molecule β-catenin antagonists would be likely to provide significant benefit, particularly for patients with advanced disease in whom surgical resection is not feasible. Although such β-catenin–targeted agents are still in preclinical development, an inhibitor of matrix metalloproteinase, a downstream target of β-catenin, substantially reduced tumor volume and tumor invasion in a transgenic Apc+/Apc1638N mouse model of aggressive fibromatosis.107
Patients with desmoids were found to have elevated levels of PDGF-AA and PDGF-BB, leading to a trial of the tyrosine kinase inhibitor imatinib in patients with advanced desmoid. Three of 19 patients (16%) had a partial response to treatment and four additional patients had stable disease for more than 1 year; overall, the 1-year tumor control rate was 37%.108 The response in these tumors was thought to be mediated by inhibition of PDGFRB kinase activity.
COMPLEX SARCOMA TYPES
Well-Differentiated and Dedifferentiated Liposarcoma
Well-differentiated and dedifferentiated liposarcomas represent the most common biological group of liposarcoma. This group is characterized by amplification of 12q, including the oncogenes MDM2, HMGA2, and CDK4. The amplifications usually occur in double minutes, ring chromosomes, and large marker chromosomes. In addition, the 12q13.2-q23.1 locus often harbors complex rearrangements (Fig. 26.2).19,109,110 On the basis of the rearrangements and correlated overexpression results, 12q may contain additional driver genes besides MDM2, HMGA2, and CDK4. The possibilities include NAV3, WIF1, MDM1, DYRK2, ELK3, DUSP6, YEATS4, TBK1, and FRS2, which were found to be amplified in ∼14% to 80% of tumors. Some of these genes are known to be involved in liposarcomagenesis, while others were not previously implicated in this disease or in cancer. Aside from 12q aberrations, dedifferentiated liposarcomas have been found to contain significant amplifications of 1p, 1q, 5p, 6q, and 20q.19,109,110 Amplification of JUN (on 1p32) has been suggested as the explanation for the block in adipocyte differentiation in undifferentiated sarcomas.111 However, more recent studies suggest that JUN is amplified or overexpressed in only a subset of dedifferentiated liposarcomas and that other alterations or activated pathways may be required to induce the dedifferentiated phenotype.19,112
Microarray analysis of differentially expressed genes in liposarcoma subtypes compared with normal fat has demonstrated activation of cell-cycle and checkpoint pathways, including the up-regulation of CDK4, MDM2, CDK1, CDC7, cyclin B1, cyclin B2, and cyclin E2 in well-differentiated and dedifferentiated liposarcoma, suggesting that these pathways may be useful as therapeutic targets.113 In fact, nutlin-3a, a selective MDM2 antagonist, induces apoptosis and inhibits proliferation of dedifferentiated liposarcoma cell lines at concentrations that have no phenotypic effects in normal adipose-derived stem cells.113 Thus, downstream p53 pathway signaling appears to be much more pronounced in dedifferentiated liposarcoma cell lines with overexpressed MDM2 than in adipose-derived stem cells, and MDM2 antagonists might serve as a effective therapeutics for liposarcomas with MDM2 amplification while having few effects on normal cells. Furthermore, PD0332991, a selective CDK4/CDK6 inhibitor currently in clinical trials, inhibits proliferation of dedifferentiated liposarcoma cells, inducing G1 cell-cycle arrest.19 These results provide a rationale for use of MDM2 antagonists and CDK4 inhibitors in patients with well-differentiated and dedifferentiated liposarcoma.
Pleomorphic Liposarcoma
Pleomorphic liposarcoma is the least common liposarcoma subtype, accounting for 5% of all liposarcomas. This subtype is characterized by high chromosome counts and complex structural rearrangements, with numerous unidentifiable marker chromosomes, nonclonal alterations, and polyploidy. This complexity has made the detection of specific recurrent rearrangements difficult.
A high-resolution single nucleotide polymorphism (SNP) array analysis has revealed that pleomorphic liposarcoma harbors multiple regions of significant copy number amplification and deletion.114 The most common alteration, found in approximately 60% of tumors, was a deletion of 13q14.2-q14.3 that includes the RB1 tumor suppressor. The next most common event was loss of 17p13.1 including TP53. Both RB1 and TP53 deletions were a mixture of hemizygous loss and less frequent homozygous deletion. Recently, TP53 point mutations were found in 17% of pleomorphic liposarcomas.19 Small molecules that reactivate mutant p53, such as PRIMA-1, are presently undergoing phase 1 trials and may have particular utility for pleomorphic liposarcomas harboring deletion or mutation in TP53.
A third genetic alteration identified in SNP analysis was frequent deletions of 17q11.2 including the tumor suppressor NF1 (neurofibromin 1). Among 24 pleomorphic liposarcomas, nine had genomic loss at this locus, eight of which were hemizygous and one was homozygous. In addition, two tumors (8%) had somatic point mutations in NF1, and both these tumors also showed deletion of the wild type allele and correspondingly reduced gene expression. These data indicate a diverse pattern of NF1 aberrations in approximately 38% of pleomorphic liposarcomas19 and suggest that MEK or mTOR inhibitors may have clinical utility, as loss of NF1 function appears to activate the RAS and mTOR pathways.
Myxofibrosarcoma and Pleomorphic Malignant Fibrous Histiocytoma
Pathologists now regard myxofibrosarcoma as a distinct tumor type with clearly defined criteria for diagnosis.115–117 Myxofibrosarcoma contains variable degrees of myxoid stroma composed of hyaluronic acid and solid sheets of spindled and pleomorphic (irregularly shaped) tumor cells. Pleomorphic malignant fibrous histiocytoma, however, is less well defined, and it remains controversial whether it represents (1) a pleomorphic sarcoma showing fibroblastic/myofibroblastic differentiation and thus sharing a common set of genomic alterations with myxofibrosarcoma, or (2) an end-stage undifferentiated morphologic pattern with genomic alterations distinct from those of myxofibrosarcoma.
Myxofibrosarcoma
Myxofibrosarcoma, also known as myxoid variant of malignant fibrous histiocytoma, includes a spectrum of malignant fibroblastic lesions with variably myxoid stroma (at least 10%), pleomorphism, and a distinctive curvilinear vascular pattern. At present, little is known about the genetic events specific to myxofibrosarcoma. Cytogenetic data are scarce, with only 49 cases described in the literature.118 Cytogenetic karyotypes tend to be highly complex, often with multiple numerical and structural rearrangements and with chromosome numbers in the triploid or tetraploid range.119,120 No specific or consistent chromosomal aberration has emerged, although some chromosomes appear to be more involved than others and ring chromosomes have been reported. In general, karyotype complexity has been greater in high-grade lesions and in recurrences.120
In an SNP array analysis of 38 myxofibrosarcomas, approximately 55% of tumors harbored chromosome 5p amplification.19 This region contains RICTOR (the rapamycin-insensitive binding partner of mTOR), CDH9, and LIFR. Other amplified regions included several discontinuous loci on 1p and 1q spanning PI4KB, ETV3, and MCL1, among others. MCL1, an antiapoptotic gene, was concomitantly overexpressed in these tumors. Myxofibrosarcomas also harbored deletions of classic tumor suppressors, including CDKN2A/CDKN2B, RB1, and TP53. These events, in combination with the inactivating mutations detected in NF1 in 11% and PTEN in 3% of myxofibrosarcomas, demonstrate extensive loss of function in several known tumor suppressors.19
Pleomorphic Malignant Fibrous Histiocytoma
Over 50% of soft tissue sarcomas occurring in older adults are histologically pleomorphic and high grade. Most have traditionally been classified as malignant fibrous histiocytoma (MFH), which has been regarded as the most common sarcoma in adults.121,122 MFH was originally defined as a pleomorphic spindle cell malignant neoplasm showing fibroblastic and histiocytic differentiation. More recently, pathologists have accepted that the morphologic pattern known as pleomorphic MFH may be shared by a wide range of malignant neoplasms.123 Many sarcomas that were previously classified as pleomorphic MFH, on careful immunohistochemical and histopathologic analysis, revealed a specific line of differentiation and could be reclassified as myxofibrosarcoma (30%), myogenic sarcoma (30%), liposarcoma (4%), malignant peripheral nerve sheath tumor (2%), or soft tissue osteosarcoma (3%), and about 30% had no specific line of differentiation or were myofibroblastic.115 Thus, the term pleomorphic MFH/sarcoma not otherwise specified is now reserved for pleomorphic sarcomas that show no definable line of differentiation by current technology.
Because diagnostic criteria have shifted over the years, the genetic aspects of pleomorphic MFH are difficult to evaluate. Among the more than 60 cases in the Mitelman Database of Chromosome Alterations in Cancer published as storiform or pleomorphic MFH or MFH not otherwise specified, the karyotypes are highly complex. The majority of tumors have chromosome numbers in the triploid or tetraploid range, but a few have near-haploid karyotypes.124–148 No specific aberrations have emerged, but telomeric associations, ring chromosomes, and/or dicentric chromosomes are frequent, although not specific for pleomorphic MFH. Unfortunately many of the cytogenetic and array-based CGH studies to date have included heterogeneous groups of tumors characterized as MFH; these studies typically show loss of 2p24, 2q32, and chromosomes 11, 13, and 16 along with gains of 7p15, 7q32, and 1p31.129–132 In many of these CGH studies, the copy number profiles of the majority of pleomorphic MFH closely resembled those of leiomyosarcomas133–135 or pleomorphic or dedifferentiated liposarcomas.136–138 Mutations and/or deletions of TP53, RB1, and INK4a have been suggested to play a critical role in pleomorphic MFH oncogenesis.131,133,139–2
Leiomyosarcoma
Leiomyosarcoma is a malignant tumor composed of cells showing distinct smooth muscle features and typically containing intersecting, sharply marginated groups of spindle cells. Karyotypes tend to be complex, with amplifications, gains, and losses involving multiple chromosomes, and karyotype generally differs between tumors.142–144 Frequent losses of 1p12-pter, 2p, 13q14-q21 (including the RB1 tumor suppressor),145 10q (including PTEN),146 and 16q and gains of 17p, 8q, and 1q21-31regions have been observed and have been associated with aggressive clinical behavior. In an analysis of copy number alterations in 27 leiomyosarcomas,19 deletions, which were more common than amplifications, included well-characterized tumor suppressors like TP53, BRCA2, RB1, and FANCA. The most prominent changes were chromosome 10 deletions (approximately 50%-70% of cases) (Fig. 26.2). Indeed, genetic inactivation of Pten (human 10q23.21) in smooth muscle in mice recapitulates human leiomyosarcoma,147 suggesting 10q loss occurs early in leiomyosarcomagenesis. In addition to PTEN inactivation, we identified homozygous deletions in MTOR. Because PTEN is a repressor of Akt, both these events suggest a critical role for aberrant Akt-mTOR signaling in leiomyosarcoma. Inhibition of mTOR using rapamycin analogues such as everolimus (RAD001) has shown some efficacy in patients with leiomyosarcoma in recent clinical trials.148 Furthermore, RB1 deletion is common in leiomyosarcomas, with 70% harboring heterozygous deletions and 8% homozygous deletions. A role for RB1 deletion in leiomyosarcoma fits with the high frequency of leiomyosarcoma observed in individuals with hereditary retinoblastoma.149
Malignant Peripheral Nerve Sheath Tumor
Malignant peripheral nerve sheath tumors (MPNSTs) are highly aggressive soft tissue sarcomas that rarely occur in the general population, but are much more common in patients with the hereditary tumor predisposition syndrome neurofibromatosis type 1 (NF1), which is caused by heterozygous mutations of the NF1 gene. NF1 patients have a lifetime incidence of MPNST of 8% to 13%.150 Morphologically, MPNSTs are monomorphic spindle cell tumors often with alternating myxoid and cellular areas. In well-differentiated lesions, tumor cell nuclei are typically buckled, resembling the pattern in neurofibroma. The spindle cells in MPNSTs are typically focally reactive for S100 protein in 50% to 70% of cases.
The NF1 gene is implicated in sporadic as well as NF1-associated MPNST. In a CGH study, about 70% of sporadic and NF1-associated MPNSTs displayed monoallelic or biallelic loss at the NF1 locus on 17q.151 NF1 encodes neurofibromin, a Ras GTPase-activating protein; it accelerates Ras–GTP hydrolysis and thus negatively regulates Ras.152 In individuals with NF1, neurofibromas develop when an unknown cell type in the Schwann cell lineage loses its remaining functional NF1 gene (by mutation, deletion, or loss of heterozygosity), leading to neurofibromin loss and subsequent activation of Ras signaling.153–155
Both sporadic and NF1-associated MPNSTs display complex karyotypes and clonal chromosomal aberrations.118,156,157 In CGH analyses of MPNSTs, the most frequent minimal regions of gain were 1q24.1-q24.2, 1q24.3-q25.1, 8p23.1-p12, 9q34.11-q34.13, and 17q23.2-q25.3.158 The 17q gain was associated with poor survival and with the overexpression of genes previously implicated in cancer: TOP2A, ETV4, ERBB2, and BIRC5.158–160
Recent studies have identified some of the genetic alterations that contribute to malignant transformation of neurofibroma to MPNST and many involve the p19ARF-MDM2-p53,161 p16INK4A-RB1,162 and EGFR signaling pathways.163,164 TP53 (on 17p13) is frequently inactivated in MPNST through mutations or deletions, correlating with the frequent loss of 17p.157 Other frequent alterations include rearrangement or loss of 13q14 and 9p21, resulting in inactivation of, respectively, RB1 and CDKN2A (encoding the p16INK4A and p14ARF cell cycle inhibitory proteins). In fact, in a recent genomewide, high-resolution analysis of copy number alterations in NF1-associated MPNST, the most frequently deleted locus (33% of cases) encompassed CDKN2A, CDKN2B, and MTAP genes on 9p21.3.154
mTOR has been found to be constitutively activated in both NF1-deficient primary cells and NF1-deficient Schwann cells derived from human neurofibromas cultured in the absence of growth factors. Furthermore, tumor cell lines derived from NF1 patients, and genetically engineered cells in which NF1 knockdown induces transformation, are highly sensitive to the mTOR inhibitor rapamycin.165 Finally, in a genetically engineered murine model, rapamycin potently suppresses the growth of aggressive NF1-associated malignancies by suppressing the mTOR target cyclin D1. These results demonstrate that mTOR inhibitors may be an effective targeted therapy for patients with neurofibromatosis and MPNST.
The activation of the Ras/Raf/MAPK pathway in the majority of MPNSTs supported targeting B-Raf with the B-Raf tyrosine kinase inhibitor sorafenib. MPNST cell lines are sensitive to sorafenib at nanomolar concentrations, and this was found to be mediated through inhibition of phospho-MEK and phospho-ERK, suppression of cyclin D1, and hypophosphorylation of RB1 at the CDK4-specific sites, resulting in G1 cell-cycle arrest.166 A phase 2 trial of sorafenib in patients with metastatic MPNST was recently completed. Although none of the 12 patients with MPNST had RECIST responses, 3 had stable disease and 2 had regression or cystification of metastatic disease.167
Angiosarcoma
Angiosarcomas (ASs) are rare vascular malignancies of endothelial cell differentiation that arise either de novo or secondary to radiation therapy or chronic lymphedema. By expression profiling, ASs are characterized by up-regulation of vascular-specific receptor tyrosine kinases, including TIE1, KDR (VEGFR2), TEK (TIE2), and FLT1 (VEGFR1).168 Full sequencing of these candidate genes identified mutations in KDR in 10% of AS patients, all of whom had tumors in the breast, with or without prior radiation. The observed KDR mutations were located in the extracellular immunoglobulinlike C2 domain, transmembrane domain, and kinase domain. KDR mutations were typically associated with strong KDR protein expression. No gains in KDR copy number were detected, even in tumors that overexpressed KDR protein. KDR mutants expressed in COS-7 cells showed ligand-independent activation of the kinase, which was inhibited with specific KDR inhibitors.168 In contrast with other sarcoma types, AS showed down-regulation of VEGF ligand expression (VEGFA and VEGFB), in keeping with the constitutive activation of KDR independent of exogenous VEGF.168 These results provide a basis for the activity of VEGFR-directed therapy in primary and radiation-induced AS.
In a recent array-CGH study, recurrent genetic abnormalities were identified in secondary but not primary AS.169 The most frequent recurrent changes were high-level amplifications on chromosome 8q24.21 (50%), followed by amplification on 10p12.33 (33%) and 5q35.3 (11%). High-level amplification of MYC on 8q24.21 was confirmed by FISH in AS associated with radiation and chronic lymphedema, but not in primary tumors. MYC amplification was not found to predispose to higher-grade morphology or increased proliferation. These findings suggest that, despite having similar morphology, primary and secondary AS are genetically distinct.
Solitary Fibrous Tumor and Hemangiopericytoma
Solitary fibrous tumor (SFT) and hemangiopericytoma are closely related histopathologically and have been suggested to represent different aspects of a single biological entity. No consistent aberrations have emerged among the approximately 20 cases of each type that have been investigated by chromosome banding analysis, although one-third of hemangiopericytomas and 10% of SFTs have shown involvement of the 12q13-15 region. The remaining cases reveal gains, losses, or structural rearrangements in several other regions, such as 4q13, 9p22-9p23, and 12q24.170,171 A homogeneous profile for SFTs has, however, emerged from gene expression profiling.172 The SFT profile is independent of anatomic location, and is distinct from profiles of other sarcoma types. This finding reinforces clinicopathologic studies that unify pleural and extrapleural SFT as a single biological entity.
The SFT expression profile includes overexpression of several tyrosine kinase receptors, such as DDR1, ERBB2, and FGFR1.172 Furthermore, overexpression of IGF2 was uniformly detected in SFT, regardless of anatomic location. IGF2 is subject to imprinting on the paternal allele in most adult tissues, and IGF2 overexpresssion in SFTs was related to loss of imprinting. Although IGF2 exerts its function by binding to IGF1R, SFTs do not show up-regulation of IGF1R, and it was suggested that IGF2 signaling occurs through the insulin receptor A pathway.175 Overexpression of IGF2 and consequent activation of the insulin receptor may also explain why a subset of SFT patients presents with hypoglycemia. This syndrome, known as Doege-Potter syndrome, has been associated with large tumor size and aggressive clinical behavior and is resolved by surgical resection of the lesion.175
Although a PDGFRB mutation (D850V) was reported in a malignant pleural SFT,175 none of the 39 SFTs tested subsequently showed mutation in this hot spot, nor did they show up-regulation of PDGFRB mRNA.172
FUTURE DIRECTIONS: FUNCTIONAL SCREENS AND NEXT-GENERATION SEQUENCING
New targeted therapies are desperately needed for the ∼4,000 patients who die each year in the United States of soft tissue sarcoma.176 A key challenge will be to identify the alterations that drive sarcomagenesis for each subtype of sarcoma. Once these driver alterations are identified, new small molecules to target them can be sought through a combination of functional screens, high-throughput compound screens, combinatorial chemistry, and structural biology information. Use of next-generation sequencing technologies can vastly expand our knowledge of the mutations, translocations, epigenetic alterations, and aberrant signaling pathways associated with specific sarcoma types and subtypes. The major advantage of next-generation sequencing is its ability to produce enormous amounts of data cheaply—in some cases more than 109 short reads per instrument run.177 Concurrent massively parallel sequencing experiments and integrative analysis now enables genomewide analysis of copy number alterations, structural rearrangements, expressed coding mutations, alternative splice forms, digital expression, chimeric/fusion transcripts, and DNA methylation status. This approach will provide a deeper view of the sarcoma genome than was previously possible. For example, on a single tumor sample it is now possible to resequence all of the protein-coding regions of the human genome, generate detailed transcriptome profiles (RNA–seq),178 and perform genomewide profiling of epigenetic marks and chromatin structure using other seq-based methods (ChIP–seq, methyl–seq, and DNase–seq).179 In gene expression studies, microarrays are being replaced by seq-based methods because RNA-seq provides far more precision on transcript levels, alternative splicing, and sequence variation in identified genes. RNA-seq has an additional advantage in that it can identify rare transcripts without prior knowledge of a particular gene.178–181 These seq-based methods, when integrated with data from high-throughput RNA interference screens in cell lines harboring the genetic alterations found in human sarcoma samples, will substantially enhance our ability to identify and target the critical signaling pathways and proteins driving sarcomagenesis.
“Smart” compounds, reflecting the three-dimensional structure of the targeted protein, may then be designed using high-throughput biochemical screens capable of identifying low-affinity compounds, together with sensitive biophysical techniques such as nuclear magnetic resonance, x-ray diffraction, and protein-ligand cocrystallography.182–185 The resulting physicochemical data should facilitate virtual screening of library structures for their three-dimensional fit with pharmacophores186 and speed the discovery of new selective small-molecule inhibitors targeting a signaling pathway essential for sarcoma growth and survival.
Selected References
The full list of references for this chapter appears in the online version.
3. Sandberg AA. Updates on the cytogenetics and molecular genetics of bone and soft tissue tumors: liposarcoma. Cancer Genet Cytogenet 2004;155:1–24.
11. Forni C, Minuzzo M, Virdis E, et al. Trabectedin (ET-743) promotes differentiation in myxoid liposarcoma tumors. Mol Cancer Ther 2009;8:449–457.
19. Barretina J, Taylor BS, Banerji S, et al. Subtype-specific genomic alterations define new targets for soft tissue sarcoma therapy. Nature Genetics 2010;42:715–721.
22. Germano G, Frapolli R, Simone M, et al. Antitumor and anti-inflammatory effects of trabectedin on human myxoid liposarcoma cells. Cancer Res 2010;70:2235–2244.
24. Ordonez JL, Osuna D, Herrero D, de Alava E, Madoz-Gurpide J. Advances in Ewing’s sarcoma research: where are we now and what lies ahead? Cancer Res 2009;69: 7140–7150.
28. Garcia-Aragoncillo E, Carrillo J, Lalli E, et al. DAX1, a direct target of EWS/FLI1 oncoprotein, is a principal regulator of cell-cycle progression in Ewing’s tumor cells. Oncogene 2008;27:6034–6043.
32. Owen LA, Kowalewski AA, Lessnick SL. EWS/FLI mediates transcriptional repression via NKX2.2 during oncogenic transformation in Ewing’s sarcoma. PLoS One 2008;3:e1965.
34. Kauer M, Ban J, Kofler R, et al. A molecular function map of Ewing’s sarcoma. PLoS One 2009;4:e5415.
35. Kovar H. Downstream EWS/FLI1 – upstream Ewing’s sarcoma. Genome Med 2010;2:8.
39. Toretsky JA, Gorlick R. IGF-1R targeted treatment of sarcoma. Lancet Oncol 2010;11:105.
40. Wachtel M, Schafer BW. Targets for cancer therapy in childhood sarcomas. Cancer Treat Rev 2010;36:318–321.
41. Erkizan HV, Kong Y, Merchant M, et al. A small molecule blocking oncogenic protein EWS-FLI1 interaction with RNA helicase A inhibits growth of Ewing’s sarcoma. Nat Med 2009;15:750–756.
43. Gerald WL, Haber DA. The EWS-WT1 gene fusion in desmoplastic small round cell tumor. Semin Cancer Biol 2005;15:197–205.
45. Stuart-Buttle CE, Smart CJ, Pritchard S, Martin D, Welch IM. Desmoplastic small round cell tumour: a review of literature and treatment options. Surg Oncol 2008;17: 107–112.
49. Haldar M, Randall RL, Capecchi MR. Synovial sarcoma: from genetics to genetic-based animal modeling. Clin Orthop Relat Res 2008;466:2156–2167.
53. de Bruijn DR, Nap JP, van Kessel AG. The (epi)genetics of human synovial sarcoma. Genes Chromosomes Cancer 2007;46:107–117.
57. Lubieniecka JM, de Bruijn DR, Su L, et al. Histone deacetylase inhibitors reverse SS18-SSX-mediated polycomb silencing of the tumor suppressor early growth response 1 in synovial sarcoma. Cancer Res 2008;68:4303–4310.
59. Nielsen TO, West RB. Translating gene expression into clinical care: sarcomas as a paradigm. J Clin Oncol 2010;28:1796–1805.
71. Wexler LH, Ladanyi M. Diagnosing alveolar rhabdomyosarcoma: morphology must be coupled with fusion confirmation. J Clin Oncol 2010;28:2126–2128.
74. Nishijo K, Chen QR, Zhang L, et al. Credentialing a preclinical mouse model of alveolar rhabdomyosarcoma. Cancer Res 2009;69:2902–2911.
75. Fisher C. Soft tissue sarcomas with non-EWS translocations: molecular genetic features and pathologic and clinical correlations. Virchows Arch 2010;456:153–166.
82. Stockwin LH, Vistica DT, Kenney S, et al. Gene expression profiling of alveolar soft-part sarcoma (ASPS). BMC Cancer 2009;9:22.
84. Stacchiotti S, Tamborini E, Marrari A, et al. Response to sunitinib malate in advanced alveolar soft part sarcoma. Clin Cancer Res 2009;15:1096–1104.
85. McArthur GA, Demetri GD, van Oosterom A, et al. Molecular and clinical analysis of locally advanced dermatofibrosarcoma protuberans treated with imatinib: Imatinib Target Exploration Consortium Study B2225. J Clin Oncol 2005;23:866–873.
105. Tejpar S, Nollet F, Li C, et al. Predominance of beta-catenin mutations and beta-catenin dysregulation in sporadic aggressive fibromatosis (desmoid tumor). Oncogene 1999;18:6615.
106. Lazar AJ, Tuvin D, Hajibashi S, et al. Specific mutations in the beta-catenin gene (CTNNB1) correlate with local recurrence in sporadic desmoid tumors. Am J Pathol 2008;173:1518–1527.
108. Heinrich MC, McArthur GA, Demetri GD, et al. Clinical and molecular studies of the effect of imatinib on advanced aggressive fibromatosis (desmoid tumor). J Clin Oncol 2006;24:1195–1203.
111. Mariani O, Brennetot C, Coindre JM, et al. JUN oncogene amplification and overexpression block adipocytic differentiation in highly aggressive sarcomas. Cancer Cell 2007;11:361–374.
112. Snyder EL, Sandstrom DJ, Law K, et al. c-Jun amplification and overexpression are oncogenic in liposarcoma but not always sufficient to inhibit the adipocytic differentiation programme. J Pathol 2009;218:292–300.
113. Singer S, Socci ND, Ambrosini G, et al. Gene expression profiling of liposarcoma identifies distinct biological types/subtypes and potential therapeutic targets in well-differentiated and dedifferentiated liposarcoma. Cancer Res 2007;67:6626–6636.
115. Fletcher CD, Gustafson P, Rydholm A, Willen H, Akerman M. Clinicopathologic re-evaluation of 100 malignant fibrous histiocytomas: prognostic relevance of subclassification. J Clin Oncol 2001;19:3045–3050.
116. Fletcher CD, Unni KK, Mertens F. Pathology and genetics of tumors of soft tissue and bone. In: Kleihues P, Sobin LH, eds. World Health Organization Classification of Tumours. Lyon, France: IARC Press, 2002.
136. Chibon F, Mariani O, Derre J, et al. ASK1 (MAP3K5) as a potential therapeutic target in malignant fibrous histiocytomas with 12q14-q15 and 6q23 amplifications. Genes Chromosomes Cancer 2004;40:32–37.
143. Sandberg AA. Updates on the cytogenetics and molecular genetics of bone and soft tissue tumors: leiomyosarcoma. Cancer Genet Cytogenet 2005;161:1–19.
144. Yang J, Du X, Chen K, et al. Genetic aberrations in soft tissue leiomyosarcoma. Cancer Lett 2009;275:1.
147. Hernando E, Charytonowicz E, Dudas ME, et al. The AKT-mTOR pathway plays a critical role in the development of leiomyosarcomas. Nat Med 2007;13:748–753.
149. Kleinerman RA, Tucker MA, Abramson DH, et al. Risk of soft tissue sarcomas by individual subtype in survivors of hereditary retinoblastoma. J Natl Cancer Inst 2007;99: 24–31.
152. Cichowski K, Jacks T. NF1 tumor suppressor gene function: narrowing the GAP. Cell 2001;104:593–604.
154. Mantripragada KK, de Stahl TD, Patridge C, et al. Genome-wide high-resolution analysis of DNA copy number alterations in NF1-associated malignant peripheral nerve sheath tumors using 32K BAC array. Genes Chromosomes Cancer 2009;48:897–907.
155. Upadhyaya M, Kluwe L, Spurlock G, et al. Germline and somatic NF1 gene mutation spectrum in NF1-associated malignant peripheral nerve sheath tumors (MPNSTs). Hum Mutat 2008;29:74–82.
163. Keizman D, Issakov J, Meller I, et al. Expression and significance of EGFR in malignant peripheral nerve sheath tumor. J Neurooncol 2009;94:383–388.
164. Ling BC, Wu J, Miller SJ, et al. Role for the epidermal growth factor receptor in neurofibromatosis-related peripheral nerve tumorigenesis. Cancer Cell 2005;7: 65–75.
165. Johannessen CM, Reczek EE, James MF, et al. The NF1 tumor suppressor critically regulates TSC2 and mTOR. Proc Natl Acad Sci U S A 2005;102:8573–8578.
166. Ambrosini G, Cheema HS, Seelman S, et al. Sorafenib inhibits growth and mitogen-activated protein kinase signaling in malignant peripheral nerve sheath cells. Mol Cancer Ther 2008;7:890–896.
167. Maki RG, D’Adamo DR, Keohan ML, et al. Phase II study of sorafenib in patients with metastatic or recurrent sarcomas. J Clin Oncol 2009;27:3133–3140.
168. Antonescu CR, Yoshida A, Guo T, et al. KDR activating mutations in human angiosarcomas are sensitive to specific kinase inhibitors. Cancer Res 2009;69:7175–7179.
169. Manner J, Radlwimmer B, Hohenberger P, et al. MYC high level gene amplification is a distinctive feature of angiosarcomas after irradiation or chronic lymphedema. Am J Pathol 2010;176:34–39.
172. Hadju M, Singer S, Maki RG, et al. IGF2 over-expression in solitary fibrous tumours is independent of anatomical location and is related to loss of imprinting. J Pathol 2010;221:300–307.
177. Metzker ML. Sequencing technologies—the next generation. Nat Rev Genet 2010;11:31–46.
178. Wang Z, Gerstein M, Snyder M. RNA-Seq: a revolutionary tool for transcriptomics. Nat Rev Genet 2009;10: 57–63.
180. Mortazavi A, Williams BA, McCue K, Schaeffer L, Wold B. Mapping and quantifying mammalian transcriptomes by RNA-Seq. Nat Methods 2008;5:621–628.
182. Strebhardt K, Ullrich A. Paul Ehrlich’s magic bullet concept: 100 years of progress. Nat Rev Cancer 2008;8: 473–480.
185. Oltersdorf T, Elmore SW, Shoemaker AR, et al. An inhibitor of Bcl-2 family proteins induces regression of solid tumours. Nature 2005;435:677–681.
186. Schneider G, Fechner U. Computer-based de novo design of drug-like molecules. Nat Rev Drug Discov 2005;4: 649–663.
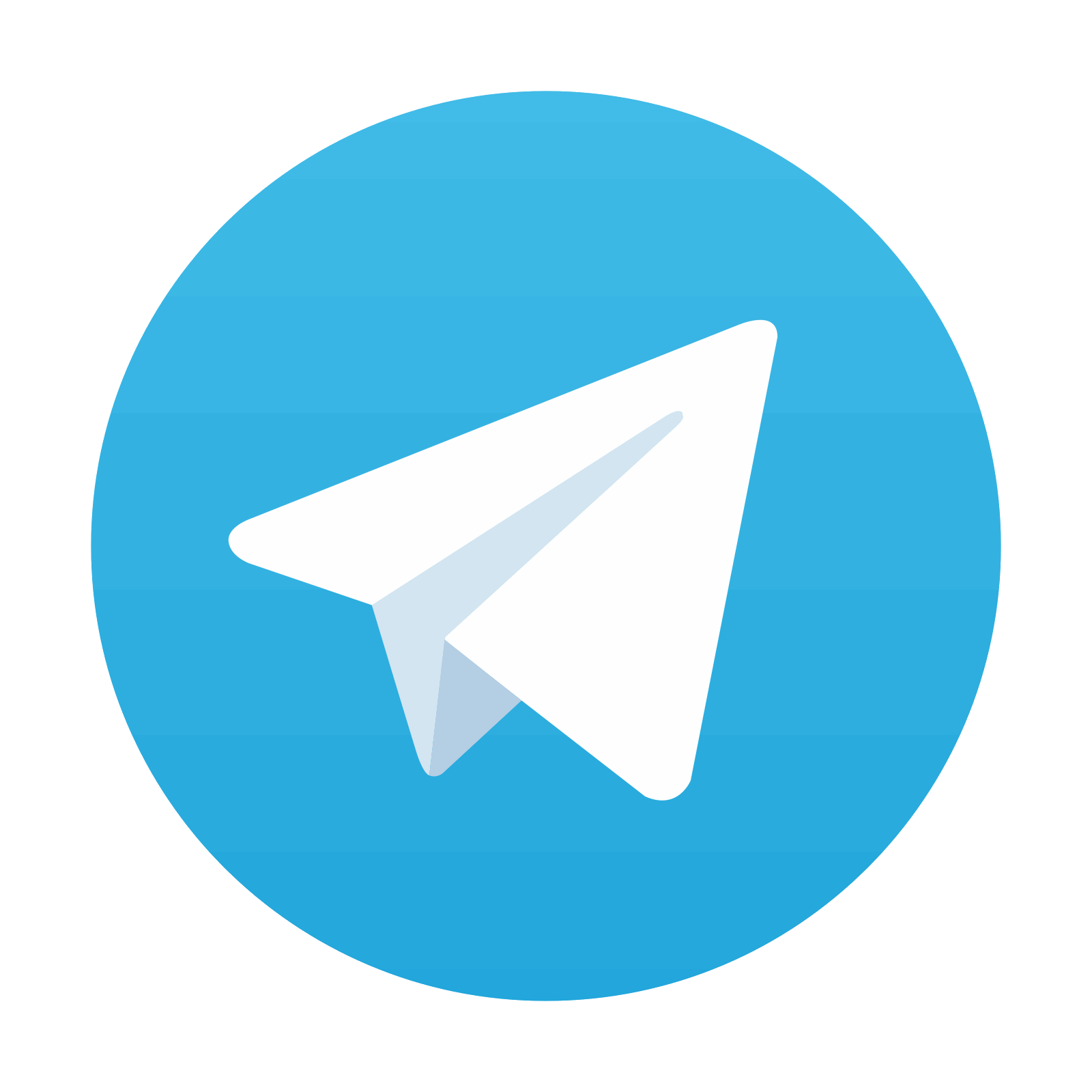
Stay updated, free articles. Join our Telegram channel

Full access? Get Clinical Tree
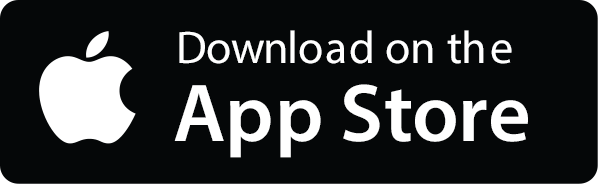
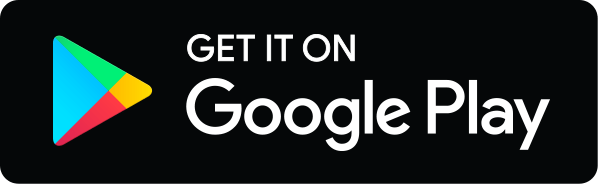