Fig. 15.1
Retinal cells and blood supply: (Modified from: Fulton, A.B. et al., Retinal degenerative and hypoxic ischemic disease. Doc Ophthalmol. 2009;118:55–61; and reproduced with permission). Abbreviations: GCL ganglion cell layer, IPL inner plexiform layer, INL inner nuclear layer, OPL outer plexiform layer, ONL outer nuclear layer, RPE retinal pigment epithelium, BRB blood-retinal barrier
The retina has a dual blood supply. The central retinal artery, a branch of the ophthalmic artery, enters the eye at the optic nerve head and branches across the inner retina. It is readily visualized with an ophthalmoscope, and in diabetes, perhaps because of this easy visual access, abnormalities of its vascular tree serve to define the severity of DR. The capillaries of this inner retinal circulation are highly specialized: endothelial cells have tight junctions which form the inner blood-retinal barrier (IBRB), while pericytes are more numerous than in any other capillary bed, equaling the number of endothelial cells. The pericytes are contractile and regulate retinal blood flow (the highest in the body per gram of tissue); they also regulate the growth and maintain the function of the endothelial cells and IBRB. On the outside of the retina, the ophthalmic artery also supplies the choroidal circulation, which lies between Bruch’s membrane and the underlying sclera. This vascular bed is separated from the neural retina by the outer blood-retinal barrier (OBRB), which is formed by tight junctions between the RPE monolayer. The choroidal circulation provides a majority (65–70 %) of the oxygen and nutrients consumed by the retina [6], but it is not visible with the ophthalmoscope, and so in this location, the effects of diabetes are less well-defined.
As mentioned, early DR is defined by damage to the inner retinal capillaries, specifically pericyte loss and leakage of the IBRB. Ophthalmoscopically, “microaneurysms” appear and are thought to be the result of proliferation of endothelial cells following loss of pericytes. Other features include “hard exudates,” which are accretions of lipid-rich material following vascular leakage, and “soft exudates,” areas of retinal edema resulting from ischemia. Later in the disease process, macular edema and neovascularization may be evident, and both are major causes of vision loss as a result of retinal detachment and/or hemorrhage [1, 2, 7–9].
For decades, two assumptions dominated DR research. First, DR was viewed as a “microvascular complication of diabetes”: one in which the retinal insult is primarily or entirely vascular in both its origin and its progression, specifically involving damage to the highly specialized inner retinal capillaries. Second, hyperglycemia has been viewed as the primary cause of both early and advanced disease. While both of these contentions still hold strong elements of truth, it has also become clear that they are oversimplifications. The injury in DR is not confined to the capillaries (and consequent ischemia) but rather affects many (perhaps all) varieties of retinal cell. Hyperglycemia is now regarded as necessary, but not sufficient, for DR to develop: other factors modulate disease severity, and understanding these will bring new opportunities for prevention and therapeutic intervention. In this chapter, we describe the development of a new lipoprotein-related concept for the propagation of DR which is consistent with a generalized retinal injury and which adds a new mechanism, in addition to effects of elevated glucose levels.
The Initiation of DR
The earliest preclinical event in the evolution of DR is unclear. It is likely to vary from one person to another, to involve several simultaneous insults, and to be scattered in space and time across the retina. Breakdown of the IBRB is an early feature: it may result from metabolic or osmotic injury from high (and fluctuating) plasma glucose levels or intermittent exposure of the capillary endothelium to the severe metabolic stresses that accompany uncontrolled diabetes (“diabetic ketoacidosis”). Such stresses include acidosis, osmotic stress, and elevation of plasma ketone bodies and free fatty acids. Supporting this, it is thought that recurrent diabetic ketoacidosis may be a risk factor for retinopathy [10]. Another early feature of DR, pericyte loss, may occur independently of, or as a result of, injury to endothelial cells and/or the IBRB but, regardless, will itself lead to endothelial injury and IBRB leakage [9]. Such leakage can be detected by fluorescein angiography and occurs at the preclinical phase [11]. Furthermore, studies using microspheres show that particles as large as 100 nm diameter can leak from retinal capillaries in the early, preclinical stages of DR in vivo in animal studies [12]. This is of relevance to our present subject, since all major classes of plasma lipoproteins are smaller than these microspheres (HDL: ~9 nm; LDL: ~20 nm; VLDL: 50–70 nm) and therefore can become extravasated early in the course of DR.
In summary, metabolic stresses of diabetes, including exposure to elevated glucose, free fatty acids, osmotic stress, and other factors, may initiate inner retinal capillary leakage, allowing the retina to be flooded with plasma constituents that normally are rigorously excluded. It is also possible that the earliest stages of diabetes, prior to IBRB leakage, may lead directly to dysfunction of other cell types (Müller cells, neurons, RPE, the choroidal circulation), but these effects are not yet well-defined. We contend that, while hyperglycemia and inner retinal capillary damage may indeed be dominant initial causal factors and features of retinal injury in DR, they are soon followed by a cascade of events in which extravasated, glycated, and oxidized lipoproteins are important promoters of endothelial, IBRB, and pericyte injury. As vicious cycles of vascular injury ensue, these modified lipoproteins may promote a generalized retinal injury. As detailed in this chapter, there is evidence that these processes are well advanced by the time clinical retinopathy becomes detectable.
Treatment Considerations for DR
The ideal treatment for DR would arrest its development in the pre-clinical phase. Efforts in this regard currently focus on control of modifiable risk factors, most notably hyperglycemia, and indeed, it appears that complete control of glycemia would completely prevent DR. Unfortunately, in the foreseeable future, it is very unlikely that normalization of glucose levels will be achieved for more than a small proportion of people affected by the diabetes epidemic worldwide. Fortunately, new knowledge of disease mechanisms means that specific measures may be developed to block progression even in the presence of hyperglycemia. For example, treatments that would enhance the integrity of the IBRB would hold promise. Existing treatments for DR address only advanced disease. Laser treatment entirely ablates areas of the retina that are ischemic, removing the angiogenic stimulus that drives PDR in neighboring regions, but in effect it sacrifices peripheral vision to save central vision. More recently, specific anti-angiogenic therapies given by intermittent intravitreal injection also aim to inhibit PDR but, by definition, are effective only when an ischemia-induced angiogenic stimulus has already developed, i.e., at a late stage in disease development.
Evidence Supporting a Role for Plasma Lipoproteins in DR
Numerous studies have sought to define associations between lipoprotein levels and severity of DR, either cross-sectionally or prospectively. There are many challenges to this work: large numbers of subjects must be studied, the plasma lipoprotein system is highly complex (there are many potential metrics), DR severity and progression over time must be assessed objectively (even the fact that a person has two eyes creates challenges), disease progression takes years, and there are numerous confounding clinical variables to be considered (age, sex, diabetes duration, long-term glycemia, renal function, medications over time, and many others). Despite these challenges, a consistent message has emerged from studies over the past 50 years [13–41] (including some recent large cohort studies reviewed below [39–41]), revealing significant associations between adverse lipoprotein levels and DR. Despite this, interest has been muted because the strength of these associations has been weak compared with (a) that between DR and hyperglycemia [42, 43] and (b) those between plasma lipoproteins and risk for atherosclerosis [44, 45].
The term “dyslipidemia” requires definition: it will be used to describe both quantitative and qualitative alterations of lipoproteins found in plasma. The former usage is the standard one and refers to altered levels of simple measures of plasma lipids, e.g., total or LDL cholesterol, HDL cholesterol, and triglycerides. The latter usage includes modification of lipoprotein particles (e.g., by glycation of apolipoproteins and phospholipids, and/or oxidation of any component, but especially unsaturated fatty acids), structural changes, altered distribution of subclasses defined in various ways, and compositional changes in the ratios of component lipids and individual apolipoproteins. Many of these qualitative changes result from or are enhanced by the presence of diabetes, most obviously glycation and oxidation. While enhanced glycation of lipoproteins occurs in plasma in diabetes [46], oxidation predominantly occurs outside the circulation, after extravasation and sequestration in vessel walls, as is established in atherogenesis. Strictly speaking, these extravascular modifications and effects of lipoproteins are distinct from properties found in plasma, i.e., they are not “dyslipidemia.”
In the past, many cross-sectional studies in type 1 and type 2 diabetes have described correlations between retinopathy and standard measures of plasma cholesterol, including total and LDL-cholesterol and LDL:HDL cholesterol ratio [14–24, 38] while some found correlations with plasma triglycerides [17, 18, 25, 28]. A series of studies from the 1960s suggested that lipid-lowering interventions, specifically clofibrate, may reduce retinal hard exudates [29–38]. Recently, two large and important prospective studies of patients with type 2 diabetes, Action to Control Cardiovascular Risk in Diabetes (ACCORD) [47] and the Fenofibrate Intervention and Event Lowering in Diabetes (FIELD) Study [48], demonstrated very significant benefits of another fibrate drug, fenofibrate, in preventing the need for laser treatment for DR. The mechanisms for this beneficial effect are currently unknown but under investigation.
More recently, associations of plasma lipoproteins with DR have been addressed in more detail. We studied 988 type 1 diabetic patients (440 women and 548 men) from the Diabetes Control and Complications Trial (DCCT) [41]. We measured detailed lipoprotein characteristics, including conventional lipid profiles, nuclear magnetic resonance lipoprotein subclass profile (NMR-LSP), apoA1, apoB, lipoprotein(a), and susceptibility of LDL to oxidation [41], to DR as assessed by the rigorous DCCT protocol (serial seven-field stereo retinal photographs read centrally [43, 49]). In brief, the lipid parameters that were positively associated with DR included serum triglycerides, serum concentrations of low-density lipoprotein (LDL), LDL particle concentration, and ApoB. The severity of retinopathy was negatively associated with HDL-cholesterol. In men, but not in women, higher levels of small-dense LDL and lower levels of large buoyant LDL were associated with severe DR, and for HDL, similar size-based associations were observed. In general, an atherogenic plasma lipoprotein profile was associated with more severe retinal disease (and of note, DR is a known risk factor for atherosclerosis in people with diabetes [50]). The Hoorn study [39], which included 2,484 50- to 74-year-old Caucasians, yielded similar findings in type 1 and type 2 diabetes (including newly diagnosed and known diabetes) in a population-based cross-sectional study. The prevalence of retinopathy was positively associated with serum cholesterol and triglyceride levels, and elevated plasma total and LDL-cholesterol levels showed associations with retinal hard exudates. Furthermore, the Pittsburgh Epidemiology of Diabetes Complications (EDC) Study [40] of a large type 1 diabetes cohort demonstrated that serum triglycerides and, to a lesser extent, higher levels of LDL-cholesterol were associated with the progression of retinopathy. Progression to proliferative retinopathy was related to higher LDL-cholesterol, serum triglycerides, as well as albumin excretion rate, and glycated hemoglobin. Furthermore, a recent report demonstrated that apoAI, apoB, and the apoB:apoAI ratio were significantly and independently associated with DR in a cross-sectional study of 224 diabetic patients (85 type 1; 139 type 2) [51].
Overall, several points are notable. The associations between the plasma lipoprotein characteristics and DR are, in general, statistically highly significant, but only moderate in magnitude. Also, in people who do not have diabetes, dyslipidemia does not cause retinal disease. Finally, dyslipidemia is very clearly associated with atherosclerosis not only in the presence [52–56] but also in the absence of diabetes. Taken together, the evidence suggests an indirect effect of plasma lipoproteins in the retina, one which is contingent upon unique properties of that tissue and upon unique effects of diabetes. We posit that this relates to the presence of the IBRB and its breakdown in diabetes.
Extravasated, Modified LDL in the Pathogenesis of DR
In atherosclerosis, elevated plasma levels of LDL and/or modified LDL (oxidized LDL: ox-LDL) are associated with cardiovascular disease [52–56], but both the modification of LDL and its harmful effects occur primarily in the arterial intima, not in plasma. We have developed a new concept: that LDL (and by extension, other plasma lipoproteins) mediate a significant proportion of retinal injury in diabetes, but do so indirectly, not by initiating vascular damage, but rather by becoming extravasated through leaking inner (and perhaps) outer blood-retinal barriers, subsequently being modified by glycation and oxidation, thereby becoming toxic towards any cells in the vicinity. Initially, such damage is patchy and the nearby cells are vascular, but later with more severe leakage, extravasated lipoproteins could permeate throughout all layers of the retina, which is only ~249 μm in total thickness [57].
Effects of Modified LDL on Retinal Capillary Vascular Cells
We have accrued considerable evidence of injurious effects of modified LDL towards a variety of retinal cell species in culture. Generally we employed two control conditions: not only native (N-)LDL (i.e., unmodified LDL), comparing its effects to modified forms, but also serum-free medium, recognizing that in the healthy retina, no extravasation of plasma lipoproteins occurs. We utilized degrees of in vitro modification and LDL concentrations designed to simulate conservatively the stresses present in diabetes in vivo. Initially, we investigated the effects of normal and mildly modified human LDL (from healthy donors) on bovine retinal capillary endothelial cell and pericytes. These modified LDLs, prepared in vitro, were intended to simulate characteristics of circulating, plasma lipoproteins, not those that had undergone more severe oxidation after extravasation. This work was intended to address the question of whether mild glycation and/or oxidation of plasma LDL might contribute to the initiation of retinal capillary injury. We found that indeed, survival of both endothelial cells and pericytes decreased with exposure to low levels of modified LDL and that toxicity increased in the following order: glycation < mild oxidation < combined glycation/mild oxidation [58].
In our more recent cell culture work, we have employed more severe degrees of LDL modification, again imposed in vitro on LDL from healthy donors, to simulate lipoproteins that have become extravasated. To prepare “highly-oxidized glycated LDL” (HOG-LDL), N-LDL was first glycated (as would happen in plasma in diabetes), then copper oxidized to simulate its fate after extravasation [59–61]. In all of this work, it is essential to maintain conditions that are pathophysiologically relevant. It is of interest that antibodies raised against copper-oxidized LDL (that had been prepared by a similar protocol to our own) recognized in vivo oxidized LDL in atheromatous plaque and, in our hands, in human diabetic retinae (see below). The concentration of LDL employed is also critical. In our work, cells are typically exposed to a range of concentrations, of which the highest is about half of typical plasma levels. Tissue levels in the diabetic retina are unknown, but estimates of ApoB levels in atheromatous plaque suggest they may be 2–79 times higher than in plasma [62, 63]. This surprising finding may be explained by extensive sequestration of LDL in vessel walls as a result of covalent cross-linking, and it is reasonable to expect a similar effect in the diabetic retina.
Modified LDL Mediates Apoptosis of Retinal Capillary Endothelial Cells and Pericytes
As detailed above, our early studies demonstrated that mild modification of LDL resulting from separate or combined processes of glycation and oxidation is implicated in chronic retinal capillary injury and thus perhaps to the initiation of DR [58], acting in concert with hyperglycemia. Using more severely modified HOG-LDL, we showed that oxidative stress and inflammation are associated with LDL-induced retinal cell death. HOG-LDL enhanced intracellular reactive oxygen species (ROS), 3-nitrotyrosine (3-NT), tyrosine nitration of prostacyclin synthase, peroxynitrite (ONOO−) formation, inducible nitric oxide synthase (iNOS) expression, and nitric oxide (NO) production, in parallel with induction of monocyte chemoattractant protein-1 (MCP-1) secretion and nuclear factor-kappaB (NF-kappaB) activation in human retinal capillary pericytes [64, 65]. Thus, HOG-LDL has pro-inflammatory and pro-oxidant effects on retinal pericytes. HOG-LDL also induced DNA fragmentation, activated the caspase cascade, and inhibited cell proliferation in pericytes, consistent with a possible contributory role in the apoptotic pericyte loss that occurs in vivo in DR [61, 66]. Exposure to HOG-LDL versus N-LDL induced similar phosphorylation of mitogen-activated protein kinase (MAPK) signaling pathways including extracellular signal-regulated kinase (ERK), p38, and Jun N-terminal kinase (JNK), and blockade of the ERK, p38, and JNK pathways did not inhibit apoptosis of pericyte induced by HOG-LDL, suggesting that apoptosis induced by HOG-LDL is independent of the activation of MAPK signaling pathways [60]. Recently, we implicated Wnt signaling pathways in DR [67, 68]. Wnt signaling pathways regulate cell proliferation and differentiation, apoptosis, stem cell maintenance, angiogenesis, inflammation, fibrosis, and carcinogenesis [69]. In our studies, modified LDL resulted in Wnt pathway activation via oxidative stress [68], and further studies are in progress. In conclusion, this body of cell culture work indicates that modified LDL, if it comes in contact with specialized retinal cells, can activate multiple intracellular pathways consistent with known characteristics of DR.
Modified LDL Influences Gene Expression in Human Retinal Capillary Pericytes
Complementing the studies described above, we used gene array studies to investigate the effects of 24 h exposure to HOG-LDL versus N-LDL in human retinal pericytes [61]. These revealed 60 genes that were altered, including members of functional pathways involving fatty acid, eicosanoid, and cholesterol metabolism; fibrinolytic regulation; cell growth and proliferation; cell stress responses; the kinin system; and angiogenesis, indicating that HOG-LDL elicits gene expression in retinal pericytes that may contribute to pericyte loss and other retinal abnormalities in DR. Pro-apoptotic and pro-angiogenic responses to HOG-LDL may be of particular importance in this regard [70]. Microarray analysis also showed that matrix metalloproteinase 1 (MMP1), MMP2, MMP11, MMP14, and MMP25 and tissue inhibitor of metalloproteinase1 (TIMP1), TIMP2, TIMP3, and TIMP4 were expressed in pericytes. Of these, only TIMP3 mRNA showed altered regulation, being expressed at significantly lower levels in response to HOG-LDL versus N-LDL [56]. Quantitative PCR and immunoblotting of cell/matrix proteins confirmed the reduction in TIMP3 mRNA and protein in response to HOG-LDL. In contrast to cellular TIMP3 protein, analysis of secreted TIMP1, TIMP2, and MMP1 and collagenase activity indicated no changes in their production in response to modified LDL. Thus, HOG-LDL selectively influences tissue inhibitor of metalloproteinase-3 gene expression and protein production among in pericytes and might contribute to microvascular abnormalities in DR [59].
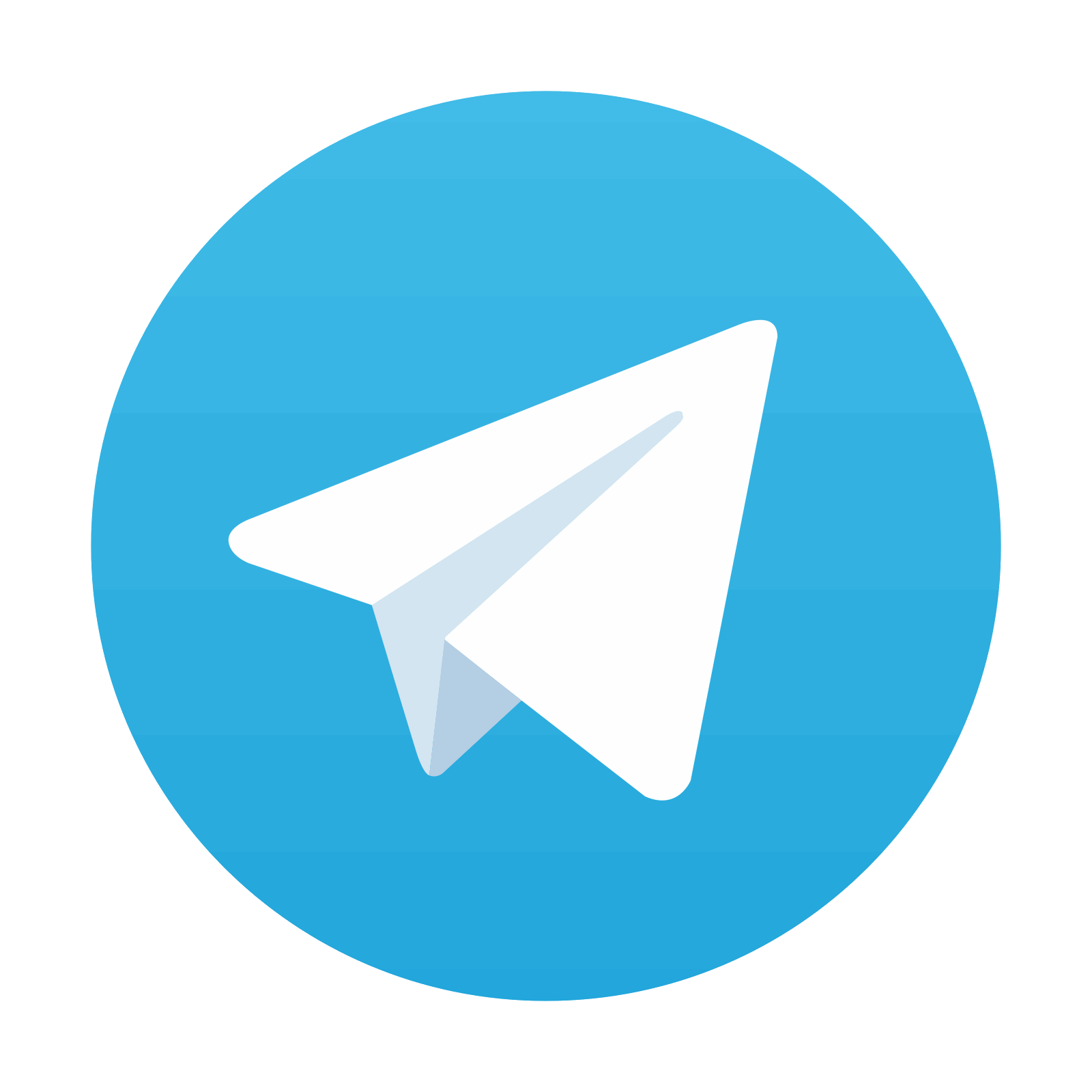
Stay updated, free articles. Join our Telegram channel

Full access? Get Clinical Tree
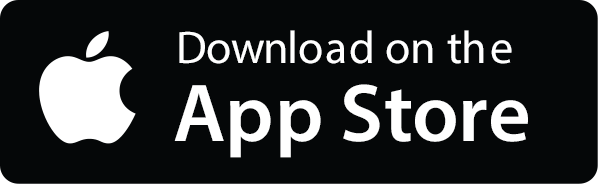
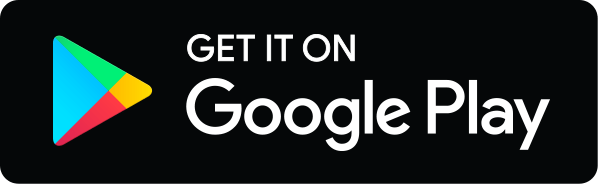